Abstract
We describe the syntheses of three different deoxy-D-allose analogs [2-deoxy-D-allose (2-DOAll), 1,2-dideoxy-D-allose (1,2-DOAll), and 1,2-didehydro-1,2-dideoxy-D-allose (1,2-DHAll)] and their fatty acid esters via regioselective lipase-catalyzed transesterification. Among them, 2-DOAll and its decanoate (2-DOAll-C10) showed higher inhibitory activity on plant growth, which is similar to D-allose (All) and its decanoate (All-C10). Bioassay results of deoxy-All-C10 on four plant species suggest that the hydroxy group at the C-1 position might be important showing growth inhibitory activity. In addition, co-addition of gibberellin (GA3) with 1,2-DHAll-C10 and 2-DOAll-C10 recovered plant growth, suggesting that they might mainly inhibit biosynthesis of gibberellin.
Graphical abstract
Biological activities of three different deoxy allose esters and allose ester on the shoots of lettuce.
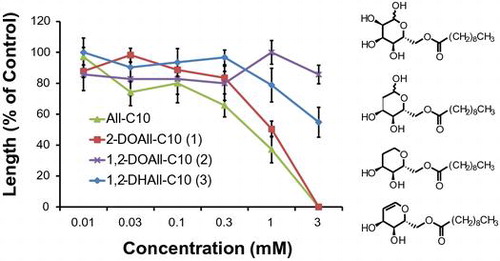
D-Allose, a C-3 epimer of D-glucose, rarely exists in nature and is defined as a rare sugar,Citation1,2) more than 50 different kinds of which are present in nature. Despite their limited occurrence, rare sugars exhibit a wide range of biological functions and are used in the pharmaceutical, cosmetics, and food industries.Citation3–6) The biological activities of D-allose, such as immunosuppressive,Citation7) anti-tumor,Citation8) anti-inflammatory,Citation9) anti-oxidative,Citation10) anti-hypertensive,Citation11) cryoprotective,Citation12) and protective effects against liver damageCitation13) in animals, have been extensively studied. It also inhibits cancer cell proliferation,Citation14) production of reactive oxygen species (ROS) in neutrophils,Citation15) and retards the plant growth.Citation16,17)
Our research group previously reported the synthesis of 6-O-acyl-D-alloses and that they showed six times greater inhibitory activity on plant growth than D-allose.Citation18,19) In addition, we described the effect of the length of the acyl group on rice growth-retarding activity.Citation20) The possibility of hydrolysis of 6-O-acyl-D-allose may be ruled out because the retardation of D-allose itself was not rescued by co-addition of gibberellin.Citation21) We also reported that the α-hydroxy group at C-3 of the D-allose derivatives might play an important role in inhibitory activity because 6-O-dodecanoate of D-mannose (a C-2 epimer of D-glucose) and D-galactose (a C-4 epimer of D-glucose) showed a weaker effect on rice growth than D-allose.Citation20) Furthermore, we have described the syntheses of 6-O-decanoyl derivatives of D-gulose (a C-4 epimer of D-allose) and D-altrose (a C-2 epimer of D-allose) and their inhibitory activities similar to that of D-allose,Citation22) confirming that the ß-hydroxy group at C-2 or C-4 did not significantly affect inhibitory activity.
In this study, to examine the structure–activity relationship (SAR) of the hydroxy groups at C-1 and C-2 of the D-allose esters, we synthesized three different analogs of deoxy-D-allose ester: 6-O-decanoyl-2-deoxy-D-allose (1: 2-DOAll-C10), lacking a hydroxy group at C-2; 6-O-decanoyl-1,2-dideoxy-D-allose (2: 1,2-DOAll-C10), which is saturated at C-1 and C-2; and 6-O-decanoyl-1,2-didehydro-1,2-dideoxy-D-allose (3:1,2-DHAll-C10), having a double bond between C-1 and C-2 (Fig. ). We then evaluated their biological activities on plant growth.
Fig. 2. Biological activities of three different deoxy alloses and D-allose on the (A) shoots and (B) roots of lettuce. Values are mean ± SE from three independent experiments.
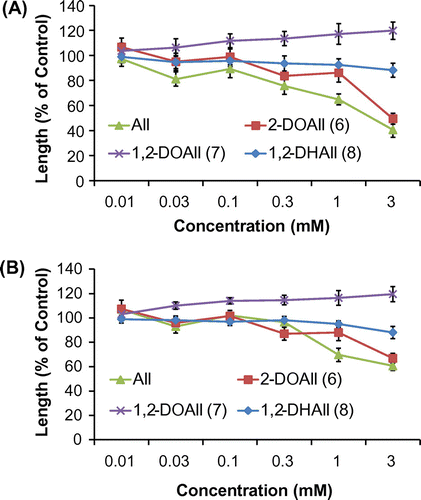
Fig. 3. Biological activities of three different deoxy allose fatty acid esters and All-C10 on the (A) shoots and (B) roots of lettuce. Values are mean ± SE from three independent experiments.
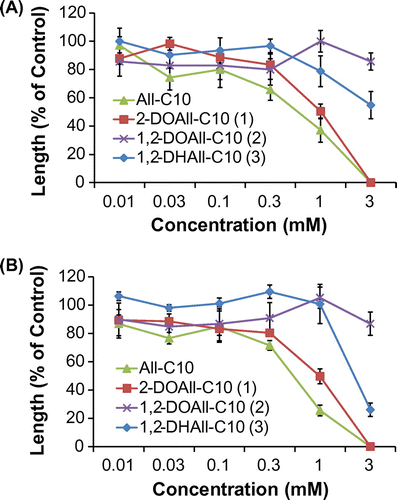
Fig. 4. Biological activities of three different deoxy allose fatty acid esters and All-C10 on the (A) full length and (B) secondary leaf sheath of rice seedlings. Values are mean ± SE from three independent experiments.
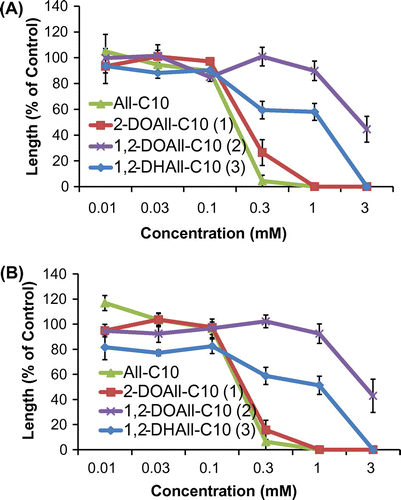
Fig. 5. Effect of GA3, 1,2-DHAll-C10 (3), and daminozide on rice seedlings. The concentration of GA3 was 1 μM and that of DHAll-C10 and daminozide was 0.4 mM and 1.5 mM, respectively, which are the IC50 value for rice seedlings. Values are mean ± SE (n = 7) and bars with different letters differ significantly from each other as determined by Tukey’s honestly significant difference comparison (p < 0.05).
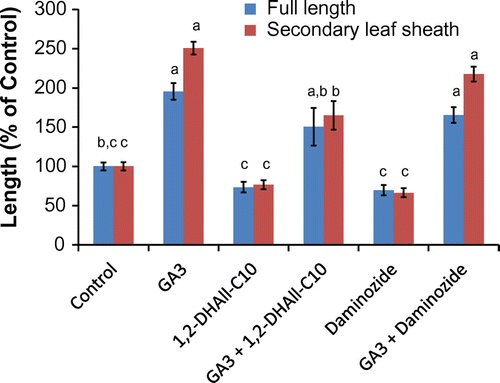
Fig. 6. Effect of GA3, 2-DOAll-C10 (1) and daminozide on rice seedlings. The concentration of GA3 was 1 μM and that of DOAll-C10 and daminozide was 0.2 mM and 1.5 mM, respectively, which are the IC50 values for rice seedlings. Values are mean ± SE (n = 7) and bars with different letters differing significantly from each other as determined by Tukey’s honestly significant difference comparison (p < 0.05).
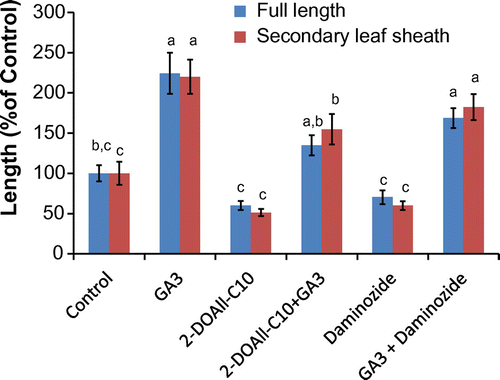
Results and discussion
2-DOAll-C10 (1) was synthesized as shown in Scheme . First, triacetyl 1,2-DHAll (4)Citation23) was added with AcOHCitation24) to give 2-deoxy-D-allose tetraacetate (5) in a 67% yield because hydration with dilute H2SO4 afforded 5 in low yield.Citation23) Then, 5 was hydrolyzed to 2-deoxy-D-allose (6) in a 69% yield. Finally, regioselective lipase-catalyzed transesterification with vinyl decanoate gave 2-DOAll-C10 (1) in a 54% yield.
6-O-Decanoyl-1,2-dideoxy-D-allose (2) and 6-O-decanoyl-1,2-didehydro-1,2-dideoxy-D-allose (3) were synthesized via regioselective lipase-catalyzed transesterification of 1,2-dideoxyallose (7) Citation23) and 1,2-didehydro-dideoxyallose (8)Citation23,25) with vinyl decanoate to give 1,2-DOAll-C10 (2) and 1,2-DHAll-C10 in a 58% and 31% yield, respectively (Scheme ). The reaction condition of lipase-transesterification of deoxy-D-alloses with vinyl decanoate was not optimized, and this may be the reason for their low-to-moderate yields.
Scheme 2. Synthesis of 6-O-decanoyl-1,2-dideoxy-D-allose (2) and 6-O-decanoyl-1,2-didehydro-1,2-dideoxy-D-allose (3).
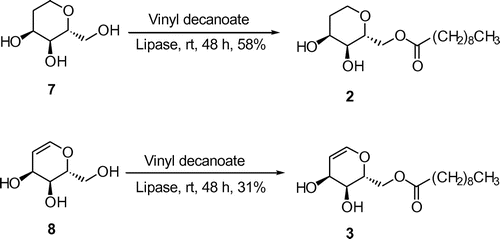
The biological activities of the deoxy alloses and their fatty acid esters were examined using lettuce, cress, Italian ryegrass, and rice seedlings. Figs. show data for lettuce and rice. The biological activities of the three analogs (6, 7, and 8) were evaluated using lettuce seedlings (Fig. ). 2-DOAll (6) showed inhibitory activity similar to that of D-allose at concentrations from 0.3 to 3 mM, whereas 1,2-DOAll (7) exhibited slight growth-promoting activity at the same concentrations. On the other hand, 1,2-DHAll (8) showed little inhibitory activity, suggesting that 8 was not converted to 6 in the assay and that the hydroxy group at C-1 is important for the activity.
The fatty acid esters of the deoxy alloses (1–3) showed higher growth inhibitory activity on lettuce than the deoxy alloses (Fig. ). 2-DOAll-C10 (1) inhibited the growth of lettuce seedlings in a concentration-dependent manner at concentrations from 0.03 to 1 mM, and completely inhibited growth at 3 mM, which was a similar tendency to that of All-C10. Although 1,2-DOAll (7) showed growth-promoting activity, its ester, 1,2-DOAll-C10 (2), showed weak inhibitory activity at 3 mM. 1,2-DHAll-C10 (3) exhibited increased inhibitory activity to some extent compared with 1,2-DHAll (8). These results suggest the effectiveness of esterification of the hydroxy group with a medium fatty acid chainCitation19) which could enhance surface activity and membrane permeability.Citation26,27)
Of the plant species tested, we found that rice seedlings were most susceptible to the deoxy allose esters and that inhibitory activity increased in a concentration-dependent manner (Fig. ). The growth of the rice seedlings was completely inhibited by 2-DOAll-C10 (1), like All-C10, at 1 and 3 mM. 1,2-DHAll-C10 (3) exhibited inhibitory activity in a concentration-dependent manner for both the full length and secondary leaf sheath of rice seedlings. 1,2-DOAll-C10 (2) showed very weak inhibitory activity even at 3 mM.
The concentrations required for 50% inhibition (IC50) of lettuce, cress, Italian ryegrass, and rice seedling hypocotyls are listed in Table . 2-DOAll-C10 (1) showed inhibitory activity on four plant species similar to that of All-C10. 1,2-DHAll-C10 (3) showed inhibitory activity on cress, Italian ryegrass, and rice seedlings similar to that of All-C10, but lower inhibitory activity on lettuce. On the other hand, saturated 1,2-DOAll-C10 (2) exhibited considerably weaker inhibitory activity than the other deoxy d-allose esters against the four plant species. These results suggest that the hydroxy group at C-2 is not necessary for inhibitory activity and that the hydroxy group and/or hemiacetal structure at C-1 might play an important role in activity.
Table 1. IC50 values of deoxy-D-allose esters and All-C10 against four plant species.
Next, we investigated the mechanism of plant growth inhibition by the deoxy-D-alloses. Known plant growth retardants such as prohexadione-calcium and daminozide which are structural mimics of 2-oxoglutaric acid are reported to block 3ß-hydroxylation of GA, the last step of GA biosynthesis.Citation28,29) It is also reported that such inhibition can be abolished by co-addition of active gibberellin (GA3). Thus, we examined the effect of co-addition of GA on growth inhibition by the deoxy-D-allose esters. As shown in Fig. , the recovery of rice growth (155%) was observed when GA3 (1 μM) was co-added with a solution of 3 (0.40 mM) as well as daminozide (1.5 mM). The withered shoot and root were not observed. In the case of 1, a similar recovery of growth by GA3 was also observed (Fig. ). These results implied that deoxy-D-allose esters might mainly inhibit gibberellin biosynthesis due to the similar behavior to daminozide.
In summary, three different deoxy-D-allose decanoate analogs were synthesized and their biological activities were evaluated. Among them, 2-DOAll-C10 exhibited inhibitory activity almost same as All-C10, and 1,2-DOAll-C10 showed little inhibitory effect on plant growth, suggesting that the hydroxy group at C-1 might play an important role in inhibitory activity. In addition, we found that growth inhibition of rice seedlings by 1,2-DHAll-C10 was abolished by co-treatment with exogenous gibberellin, GA3, implying that the deoxy-D-allose esters might mainly inhibit biosynthesis of GA.
Experimental
General experimental procedures
Candida antarctica lipase (Novozym 435) immobilized on a macroporous acrylic resin was obtained from Novo Nordisk (Bagsvaerd, Denmark). D-Allose was provided by the Rare Sugar Research Center at Kagawa University, Japan. Dehydrated acetonitrile and acetone (dehydrated and analytical grade) were purchased from Wako Chemical Co. (Tokyo, Japan) and used without further dehydration. 2-DOAll (6), 1,2-DOAll (7), and 1,2-DHAll (8) were already described.Citation23,25,30) All other reagents were purchased from Wako Chemical Co. A qualitative analysis of the reaction mixtures was carried out using TLC on silica gel 60 (0.25 mm; Merck), and column chromatography was performed with silica gel (Wakogel C-300HG, 40–60 μm). 1H and 13C-NMR were measured at 600 and 150 MHz, respectively, with a Jeol JNM-ECA600 spectrometer in CD3OD at room temperature using TMS as an internal standard. Optical rotation data were measured with a Jasco P-1010 optical rotation polarimeter using methanol. High-resolution mass spectrum (HRMS) was measured by a Waters Xevo G2-XS-TOF mass spectrometer.
2-deoxy-D-allose (6)Citation23,30) AcOH (24 μL) and Ph3PHBr (7.4 mg) were added to 4 (39.0 mg, 0.143 mmol) in CH2Cl2 (1.43 mL) at room temperature for 3 h. The reaction was quenched adding saturated NaHCO3 until gas evolution ceased. The aqueous layer was extracted with ethyl acetate. The combined organic layers were dried over Na2SO4, filtered, and concentrated in vacuo. The crude mixture was purified by flash column chromatography using a solvent mixture of hexane and ethyl acetate (1:1) to give 5 as a colorless liquid (14.9 mg, 0.0448 mmol, 56%). 5 (0.028 mmol) was hydrolyzed using potassium carbonate (3.0 mg) in methanol (0.50 mL) for 20 min at room temperature to give 6 as a colorless liquid (2.5 mg, 0.015 mmol, 69%). 1H and 13C-NMR spectra showed that it existed in a mixture of α-pyranose, ß-pyranose, α-furanose, and ß-furanose (1 : 2.30 : 0.70 : 0.50). 1H-NMR (600 MHz, CD3OD) δ for ß-pyranose: 3.44 (1H, dd, J = 9.60, 3.12 Hz), 3.65 (2H, m), 3.74 (2H, m), 3.80 (1H, ddd, J = 11.64, 6.36, 2.58 Hz), 4.03 (1H, t, J = 3.12 Hz), 5.09 (1H, dd, J = 9.60, 2.22 Hz). 13C-NMR (150 MHz, CD3OD) δ for ß-pyranose: 92.96, 75.53, 69.19, 68.97, 63.63, 40.39; for α-pyranose: 93.04, 69.74, 69.52, 68.47, 63.10, 36.50; for α-furanose: 99.67, 87.83, 74.08, 72.89, 64.50, 43.48; for ß-furanose: 99.52, 87.10, 73.52, 72.34, 64.54, 43.0.
6-O-Decanoyl-2-deoxy-D-allose (1)
Vinyl decanoate (57 μL, 3 equiv) was added to a mixture of 6 (17.0 mg, 0.103 mmol) and Novozym 435 (56.0 mg) in acetonitrile (0.25 mL). The suspension was stirred using a magnetic bar (300 rpm) at 45 ºC. Enzyme reaction progress was monitored using TLC. After 21 h, the filtrate through Celite was purified by column chromatography on silica gel using a solvent mixture of ethyl acetate and methanol (1:1, v/v) to give 1 as a colorless liquid (17.8 mg, 0.0563 mmol, 54%). 1H-NMR (600 MHz, CD3OD) δ for ß-pyranose: 1.75 (3H, m), 1.94 (7H, m), 2.15 (2H, m), 2.24 (16 H, m), 3.37 (5H, ddd, J = 15.12, 9.96, 3.06 Hz), 3.81(3H, m), 3.95 (5H, t, J = 3.12 Hz), 4.08 (6H, m), 4.15 (4 H, m), 4.27 (6H, m), 4.42(1H, t, J = 5.5 Hz), 5.00 (3 H, m), 5.04 (2H, d, J = 3.12), 5.34 (1H, m), 5.43 (1H, m). 13C-NMR (150 MHz, CD3OD) δ for ß-pyranose: 14.43, 23.72, 25.99, 30.22, 30.41, 30.43, 30.57, 33.04, 34.97, 40.36, 65.65, 68.82, 69.04, 73.02, 93.05, 175.62. + 40.0º (c 0.300, MeOH). Rf 0.45 (ethyl acetate/methanol = 30/1). HRMS m/z (M + Na)+: calculated for C16H30O6Na, 341.1940; found, 341.1968.
6-O-Decanoyl-1,2-dideoxy-D-allose (2)
Vinyl decanoate (50 μL, 0.22 mmol) was added to a mixture of 7 (20.3 mg, 0.137 mmol) and Novozym 435 (37.0 mg) in acetone (0.62 mL). The suspension was stirred using a magnetic bar at 300 rpm. Enzyme reaction progress was monitored using TLC. After 2 d, the filtrate through Celite was purified by column chromatography on silica gel using a solvent mixture of hexane and ethyl acetate (1:1, v/v) to give 2 as a colorless liquid (24.2 mg, 0.0800 mmol, 58%). 1H-NMR (600 MHz, CD3OD) δ: 0.90 (3H, t, J = 7.56 Hz), 1.31 (12H, m), 1.61 (2H, m), 1.76 (1H, m), 1.84 (1H, m), 2.34 (2H, t, J = 7.56 Hz), 3.42 (1H, dd, J = 9.66, 2.76 Hz), 3.65 (1H, dd, J = 5.52, 1.38 Hz), 3.74 (2H, ddd, J = 10.32, 4.8, 2.04), 4.04 (1H, dd, J = 5.52, 2.76 Hz), 4.14 (1H, dd, J = 11.7, 6.18 Hz), 4.35 (1H, dd, J = 11.7, 2.04 Hz). 13C-NMR (150 MHz, CD3OD) δ: 14.44, 23.71, 26.03, 30.15, 30.40, 30.42, 30.58, 33.03, 33.63, 34.97, 62.69, 65.70, 67.94, 69.31, 75.25, 175.62. + 42.2o (c 1.16, MeOH). Rf 0.38 (hexane/ethyl acetate = 30/1). HRMS m/z (M + Na)+: calculated for C16H30O5Na, 325.1991; found, 325.2010.
6-O-Decanoyl-1,2-didehydro-1,2-dideoxy-D-allose (3)
Vinyl decanoate (48 μL, 0.21 mmol) was added to a mixture of 8 (19.1 mg, 0.131 mmol) and Novozym 435 (36.0 mg) in acetone (0.60 mL). The suspension was stirred using a magnetic bar at 300 rpm. Enzyme reaction progress was monitored using TLC. After 2 d, the filtrate through Celite was purified by column chromatography on silica gel using a solvent mixture of hexane and ethyl acetate (1:1, v/v) to give 1 as a colorless liquid (11.3 mg, 0.0376 mmol, 31%). 1H-NMR (600 MHz, CD3OD) δ: 0.90 (3H, t, J = 6.9 Hz), 1.30 (14H, m), 1.62 (2H, m), 2.35 (2H, t, J = 6.8 Hz), 3.69 (1H, dd, J = 11.0, 4.1 Hz), 3.98 (1H, ddd, J = 11.0, 4.8, 2.1 Hz), 4.02 (1H, dd, J = 5.5, 4.1 Hz), 4.28 (1H, dd, J = 12.4, 4.8 Hz), 4.48 (1H, dd, J = 12.4, 2.1 Hz), 4.92 (1H, t, J = 5.5), 6.40 (1H, d, J = 5.5). 13C-NMR (150 MHz, CD3OD) δ: 14.44, 23.72, 26.06, 30.15, 30.42, 30.58, 33.04, 34.97, 63.21, 64.48, 68.32, 73.40, 102.44, 146.70. + 62.2º (c 0.225, MeOH). Rf 0.45 (hexane/ethyl acetate = 30/1). HRMS m/z (M + Na)+: calculated for C16H28O5Na, 323.1834; found, 323.1866.
Biological assay
Activities of the synthesized compounds were tested using four different plant species: lettuce (Lactuca sativa), cress (Lepidium sativum), Italian ryegrass (Lolium multiflorum), and rice seedlings (Oryza sativa L. cv. Nihonbare). Experiments were carried out as described previously.Citation21)
Bioassay on lettuce, cress, and Italian ryegrass
2-DOAll and 6-O-decanoyl-2-DOAll, 1,2-DOAll and 6-O-decanoyl-1,2-DOAll, and 1,2-DHAll and 6-O-decanoyl-1,2-DHAll were dissolved in a small volume of methanol, added to a sheet of filter paper (Toyo No. 2) in a 3.5-cm Petri dish and then dried. The filter paper in the Petri dish was then moistened with 0.8 mL of a 0.05% (v/v) aqueous solution of Tween 20. Ten (10) test species were arranged on the filter paper and grown in the dark at 25 ºC. The control seedlings were treated with only a solution of Tween 20. The lengths of the hypocotyls or shoots and roots of the lettuce (Lactuca sativa), cress (Lepidium sativum), and Italian ryegrass (Lolium multiflorum) seedlings were measured after 48 h, and the percentage of shoot length and root lengths were calculated with reference to the length of the control plants.
Bioassay on rice seedlings
Rice seeds (Oryza sativa L. cv. Nihonbare) were sterilized with ethanol for 5 min and then washed with water. The seeds were then sterilized for 30 min with 1% sodium hypochlorite and washed again with water. The sterilized seeds were soaked in water for 2 d at 30 ºC under fluorescent light. Seven germinated seeds were transplanted into a tube containing the test solution and 0.05% Tween 20 (2 mL each). After incubating for 7 d under light, the total length and length of the second leaf sheath of each rice seedling were measured and the growth ratios against the control value were calculated.
Author contributions
M.T.I.C. and Y.K. designed the study. M.T.I.C. and H.A. carried out the experiments. M.T.I.C., Y.K., and R.C.Y. wrote the manuscript.
Disclosure statement
No potential conflict of interest was reported by the authors.
Funding
This work was supported by the Kagawa University and the United Graduate School of Agricultural Sciences, Ehime University and the Ministry of Education, Culture, Sports, Science and Technology (MEXT), Japan.
Acknowledgments
The first author is grateful to the Ministry of Education, Culture, Sports, Science and Technology (MEXT), Japan for providing financial support.
References
- Izumori K. Bioproduction strategies for rare hexose sugars. Naturwissenschaften. 2002;89:120–124.10.1007/s00114-002-0297-z
- Izumori K. Izumoring: a strategy for bioproduction of all hexoses. J. Biotechnol. 2006;124:717–722.10.1016/j.jbiotec.2006.04.016
- Wanmeng M, Zhang W, Feng Y, et al. Recent advances on applications and biotechnological production of d-psicose. Appl. Microbiol. Biotechnol. 2012;94:1461–1467.
- Hoshikawa H, Indo K, Mori T, et al. Enhancement of the radiation effects by d-allose in head and neck cancer cells. Cancer Lett. 2011;306:60–66.10.1016/j.canlet.2011.02.032
- Granström TB, Izumori K, Leisola M. A rare sugar xylitol. part II: biotechnological production and future applications of xylitol. Appl. Microbiol. Biotechnol. 2007;74:273–276.10.1007/s00253-006-0760-4
- Levin GV, Zehner LR, Saunders JP, et al. Sugar substitutes: their energy values, bulk characteristics and potential health benefits. Am. J. Clin. Nutr. 1995;62:1161S–1168S.
- Hossain MA, Wakabayashi H, Goda F, et al. Effect of immunesuppressants FK506 and D-allose on allogenic orthotopic liver transplantation in rats. Transplant. Proc. 2000;32:2021–2023.10.1016/S0041-1345(00)01540-2
- Yamaguchi F, Kamitori K, Sanada K, et al. Rare sugar d-allose enhances anti-tumor effect of 5-fluorouracil on the human hepato cellular carcinoma cell line HuH-7. J. Biosci. Bioengin. 2008;106:248–252.10.1263/jbb.106.248
- Gao D, Kawai N, Tamiya T. The anti-inflammatory effects of d-allose contribute to attenuation of cerebral ischemia–reperfusion injury. Med. Hypotheses. 2011;76:911–913.10.1016/j.mehy.2011.03.007
- Ishihara Y, Katayama K, Sakabe M, et al. Antioxidant properties of rare sugar D-allose: effects on mitochondrial reactiveoxygen species production in Neuro A cells. J. Biosci. Bioengin. 2011;112:638–642.10.1016/j.jbiosc.2011.08.005
- Kimura S, Zhang G-X, Nishiyama A, et al. D-allose, an all-cis aldo-hexose, suppresses development of salt-induced hypertension in Dahl rats. J. Hypertens. 2005;23:1887–1894.10.1097/01.hjh.0000182523.29193.e3
- Sui L, Nomura R, Dong Y, et al. Cryoprotective effects of d-allose on mammalian cells. Cryobiology. 2007;55:87–92.10.1016/j.cryobiol.2007.05.003
- Hossain MA, Izuishi K, Maeta H. Protective effects of D-allose against ischemia reperfusion injury of the liver. J. Hepato-Biliary-Pancreatic Sci. 2003;10:218–225.10.1007/s00534-002-0785-8
- Sui L, Dong Y, Watanabe Y, et al. The inhibitory effect and possible mechanisms of D-allose on cancer cell proliferation. Int. J. Oncol. 2005;27:907–912.
- Murata A, Sekiya K, Watanabe Y, et al. A novel inhibitory effect of d-allose on production of reactive oxygen species from neutrophils. J. Biosci. Bioengin. 2003;96:89–91.10.1016/S1389-1723(03)90104-6
- Kano A, Gomi K, Yamasaki-Kokudo Y, et al. A rare sugar, D-allose, confers resistance to rice bacterial blight with upregulation of defense-related genes in Oryza sativa. Phytopathology. 2010;100:85–90.10.1094/PHYTO-100-1-0085
- Kato-Noguchi H, Takaoka T, Izumori K. Psicose inhibits the lettuce growth via a hexokinase-independent pathway. Physiol. Plant. 2005;125:293–298.10.1111/ppl.2005.125.issue-3
- Afach G, Kawanami Y, Izumori K. Synthesis of D-allose fatty acid esters via lipase-catalyzed regioselective transesterification. Biosci. Biotechnol. Biochem. 2005;69:833–835.10.1271/bbb.69.833
- Afach G, Kawanami Y, Kato-Noguchi H, et al. Practical production of 6-O-octanoyl-d-allose and its biological activity on plant growth. Biosci. Biotechnol. Biochem. 2006;70:2010–2012.10.1271/bbb.60150
- Kobayashi M, Ueda M, Furumoto T, et al. Retarding activity of 6-O-acyl-D-allose against plant growth. Biosci. Biotechnol. Biochem. 2010;74:216–217.10.1271/bbb.90713
- Fukumoto T, Kano A, Ohtani K, et al. Rare sugar D-allose suppresses gibberellin signaling through hexokinase-dependent pathway in Oryza sativa L. Planta. 2011;234:1083–1095.10.1007/s00425-011-1463-3
- Chowdhury MTI, Naito M, Yanagita RC, et al. Synthesis of 6-O-decanoyl-d-gulose and 6-O-decanoyl-D-gulose and evaluation of their biological activity on plant growth. Plant Growth Regul. 2015;75:707–713.10.1007/s10725-014-9972-2
- Haga M, Tejima S. Synthesis of 3,4,6-tri-O-acetyl-1,5-anhydro-2-deoxy-d-ribo-hex-1-enitol and 2,3,4,6-tetra-O-acetyl-1,5-anhydro-d-ribo-hex-1-enitol. Carbohydr. Res. 1974;34:214–218.10.1016/S0008-6215(00)80388-2
- Morris WJ, Shair MD. Stereoselective synthesis of 2-deoxy-ß-glycosides using anomeric O-alkylation/arylation. Org. Lett. 2009;11:9–12.10.1021/ol8022006
- Guthrie RD, Irvine RW. A facile synthesis of D-allal and its derivatives. Carbohydr. Res. 1979;72:285–288.10.1016/S0008-6215(00)83951-8
- Fujii T. Biosurfactants. J. Jpn. Oil Chem. Soc. 1996;45:1013–1021.10.5650/jos1996.45.1013
- Ogino K. Research and development of novel surfactants. J. Jpn. Oil Chem. Soc. 1996;45:921–932.10.5650/jos1996.45.921
- Crozier A, Kamiya Y, Bishop G, et al. Biosynthesis of hormones and elicitor molecules. In: Buchanan B, Guissem W, Jones R, editors. Biochemistry and molecular biology of plants. Rockville, Maryland: American Society of Plant Physiologist; 2000. p. 850–929.
- Rademacher W. Growth retardants effects on gibberellins biosynthesis and other metabolic pathways. Annu. Rev. Plant Physiol. Plant Mol. Biol. 2000;51:501–531.10.1146/annurev.arplant.51.1.501
- Robins MJ, Nowak I, Wnuk SF, et al. Deoxygenative [1,2]-hydride shift rearrangements in nucleoside and sugar chemistry: analog with the [1,2]-electron shift in the deoxygenation of ribonucleotide reductases. J. Org. Chem. 2007;72:8216–8221.10.1021/jo071102b