Abstract
Inorganic polyphosphate (polyP) was previously identified as a probiotic-derived substance that enhances intestinal barrier function. PolyP-accumulating bacteria are expected to have beneficial effects on the human gastrointestinal tract. In this study, we selected Lactobacillus paracasei JCM 1163 as a strain with the potential to accumulate polyP, because among the probiotic bacteria stored in our laboratory, it had the largest amount of polyP. The chain length of polyP accumulated in L. paracasei JCM 1163 was approximately 700 phosphate (Pi) residues. L. paracasei JCM 1163 accumulated polyP when Pi was added to Pi-starved cells. We further improved the ability of L. paracasei JCM 1163 to accumulate polyP by nitrosoguanidine mutagenesis. The mutant accumulated polyP at a level of 1500 nmol/mg protein—approximately 190 times that of the wild-type strain. PolyP extracted from the L. paracasei JCM 1163 significantly suppressed the oxidant-induced intestinal permeability in mouse small intestine. In conclusion, we have succeeded in breeding the polyP-accumulating Lactobacillus mutant that is expected to enhance intestinal barrier function.
Graphical abstract
Time course of polyP production and overplus induction in L. paracasei JCM 1163
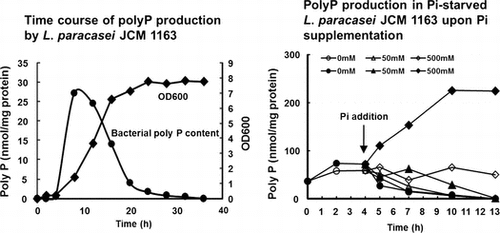
The human gastrointestinal tract is colonized by a vast community of bacteria. Well-balanced interactions between the host and gut microbiota provide health benefits for the body.Citation1) Probiotics in particular deliver beneficial effects including competitive exclusion of pathogenic bacteria,Citation2) induction of defensin production,Citation3,4) modulation of host immune function,Citation5) and improvement of intestinal barrier function.Citation6) In our previous study, we analyzed unknown substances in the culture supernatant of Lactobacillus brevis SBC8803 that enhance intestinal barrier function and discovered that polyphosphate (polyP), a liner polymer composed of tens to hundreds of phosphate (Pi) residues linked by phosphoanhydride bonds,Citation7,8) induces cytoprotective inducible heat-shock protein (HSP) 27 in human gastrointestinal epithelial Caco2/bbe cells.Citation9) Enzymatically synthesized polyP also significantly suppressed the oxidant-induced intestinal permeability in mouse small intestine. Daily intrarectal administration of polyP improved the inflammation grade and survival rate in 4% sodium dextran sulfate-administered mice.Citation9) However, the amount of polyP used in the above-described experiment was higher than that contained in a reasonable ingested dose of L. brevis SBC8803. Thus, breeding of probiotic bacteria capable of producing higher amounts of polyP would be necessary for polyP-derived enhancement of intestinal barrier function in humans.
PolyP is ubiquitous in nature and is widely distributed among microorganisms.Citation10) Polyphosphate kinase 1 (PPK1) is an enzyme that catalyzes the transfer of the terminal Pi residue of ATP to short-chain polyP, generating long-chain polyP.Citation10,11) Another widely distributed polyphosphate kinase (PPK2), which has no sequence identity to PPK1, has been found as an enzyme catalyzing GTP synthesis from GDP and polyP.Citation10,11) However, polyP-accumulating ability differs among microorganisms. In this study, we first investigated the ability of probiotic bacteria stored in our laboratory to accumulate polyP, and selected L. paracasei JCM 1163 as a high polyP-accumulating strain. PolyP accumulation is affected by culture conditions. For example, Pi-starved cells replenished with Pi show excessive polyP accumulation.Citation12) We therefore also investigated suitable culture conditions for the production of PolyP in L. paracasei JCM 1163. Finally, with the aim of breeding probiotic bacteria that potentially enhance intestinal barrier function in humans, we selected an L. paracasei JCM1163 mutant that accumulated a much higher amount of polyP than the wild type.
Materials and methods
Microorganisms
The lactic acid bacteria and bifidobacteria used in this study were obtained from Sapporo Breweries Ltd (Tokyo, Japan) or purchased from RIKEN Bioresource Center (Tsukuba, Japan). These lactobacilli and bifidobacteria were cultured in de Man–Rogosa–Sharpe (MRS) broth (Difco Laboratories, Detroit, MN), or soy peptide HK (SP) medium (Fuji Oil Co., Ltd., Osaka Japan), or simplified chemically defined medium (SCDM)Citation13) with or without dipotassium hydrogen phosphate for 24 h at 37 °C.
Extraction of polyP with glass milk
PolyP extraction from the lactic acid bacteria and bifidobacteria or the culture supernatant and determination of polyP concentration were conducted according to a previously reported procedure.Citation14) The bacterial broth was pelleted in a 1.5-mL tube for 5 min in a centrifuge (12,000 × g). Lysis buffer containing 350 μL of 4 M guanidine isothiocyanate (GITC), 50 mM Tris-hydrochloric acid, pH 7.0, was added to the pellet. Tubes were vortexed, incubated for 2 min at 90 °C, and sonicated for 3 min. A sample (50 μL) was removed for protein estimation with Coomassie Plus Protein Assay Reagent (Thermo Fisher Scientific K.K., Yokohama, Japan). Thirty microliters of 10% sodium dodecyl sulfate (SDS), 300 μL of ethanol, and 3 μL of glass milk were added to the tubes. After vortexing, the tubes were centrifuged briefly to pellet the glass milk. The pellet was then suspended in 300 μL of cold NEW Wash buffer (5 mM Tris-hydrochloric acid [pH 7.5], 50 mM sodium chloride, 5 mM ethylenediamine tetraacetic acid, 50% ethanol) by vortexing and subsequently pelleted by centrifugation; these washing steps were repeated twice. The washed pellet was then suspended in 50 μL of 50 mM Tris-hydrochloric acid (pH 7.4), 10 mM magnesium chloride, 20 μg each of deoxyribonuclease and ribonuclease per mL, and then incubated at 37 °C for 15 min. The pellet was washed first with 150 μL of 4 M GITC lysis buffer and 150 μL of ethanol and then twice with NEW Wash buffer. PolyP was eluted from the pellet with 100 μL of distilled water at 90 °C for 2 min.
Assay of polyP
PolyP was converted to adenosine triphosphate (ATP) by polyphosphate kinase (PPK) under the condition of high adenosine diphosphate (ADP) concentration (0.5 mM). Ten microliters of the reaction mixture (3 μL of sample or blank, 2 μL of 0.5 mM ADP, 1 μL of 15 μg/mL PPK, 3 μL of 3.3 × buffer [165 mM HEPES-potassium hydroxide, 132 mM ammonium sulfate, 13.2 mM magnesium chloride]) was incubated at 37 °C for 60 min. Five microliters of the reaction mixture was added to 40 μL of luciferase reaction mixture (ATP Bioluminescence Assay Kit CLS II, Roche Applied Science, Indianapolis, IN). Luminescence was measured by a luminometer (GloMax™ 96 Microplate Luminometer, Promega KK, Tokyo, Japan). A standard curve for ATP (0, 0.55, 1.1, 1.65, and 3.3 pmol) was used for the calculation of ATP concentration in the reaction mixture.
Measurement of Pi concentration
One milliliter of sample solution was mixed with 200 μl of molybdenum blue assay reagent (0.1 M ascorbic acid, 2.5 N sulfuric acid, 0.27 M potassium antimonyl tartrate sesquihydrate, 2.16 M ammonium molybdate tetrahydrate) and incubated at room temperature for at least 10 min. Pi concentration was determined from the absorbance at 880 nm and calculated from a standard curve.
Electrophoresis of polyP
For analytical polyacrylamide gel electrophoresis, the polyP isolated from lactic acid bacteria was dissolved in 6 × loading dye. The sample (10 μL) was loaded on a 15% polyacrylamide gel (90 mm × 105 mm × 1 mm). The gel was electrophoresed at 10 mA. After electrophoresis, the gel was stained with the staining solution [0.05% Toluidine Blue O dissolved in 25% methanol] for at least 15 min, and destained with destaining solution (25% methanol) for at least 10 min several times until a clear background was obtained.
Improvement of polyP production in L. paracasei JCM 1163 mutants obtained by nitrosoguanidine treatment
L. paracasei JCM 1163 at the early logarithmic growth phase was treated with 0.5 mg/ml nitrosoguanidine (NTG) for 1 h, and then seeded in MRS medium containing 0.005% 5-bromo-4-chloro-3-indolyl-phosphate (X-Pi). Mutants that showed blue-colored colonies on the MRS medium were selected and their polyP contents were measured.
Ex vivo intestinal loop studies
Male C57BL/6 mice (6-week-old) were housed in an air-conditioned room maintained at 23 ± 2 °C at a relative humidity of 55 ± 15%. All procedures were conducted in accordance with the guidelines for the care and use of experimental animals of the Japanese association for laboratory animals and approved by the Ethical Committee at Sapporo Breweries. C57BL/6 mice (n = 5) were sacrificed and the small intestine was removed beginning at the ligament of Treitz. The small intestine was divided into 3–6 pieces, each end was ligated with silk sutures and the loops were filled with RPMI 1640 medium containing the polyP extracted from L. paracasei JCM 1163. Loops were incubated for 2 h at 37 °C in a 5% CO2 incubator. To measure the permeability effects, the loops were filled with RPMI 1640 medium containing 1 μCi/mL [3H]-mannitol with or without 0.3 mM freshly prepared monochloramine. Loops were placed into the middle section of an organ culture dish in 4 mL of RPMI 1640 without monochloramine. RPMI 1640 medium samples from the culture dish were taken at 5, 20, and 35 min to determine the flux of mannitol from the lumen to the medium outside the loops. All values are expressed as mean ± standard errors of the mean (SEM). Statistical evaluation of the results was performed by one-way analysis of variance (ANOVA) followed by the Dunnett test. The analysis was performed using Graph Pad Prism (Ver. 4) software. A probability value of less than 0.05 was considered statistically significant.
Results and discussion
Selection of polyP-accumulating lactic acid bacteria and bifidobacteria
In our previous study, we found that polyP of L. brevis SBC8803 enhances the epithelial barrier function and maintains intestinal homeostasis through integrin-p38 MAPK pathway.Citation9) To screen lactic acid bacteria and bifidobacteria capable of accumulating high amounts of polyP, we tested the amounts of polyP in 55 different strains of Lactobacillus species (L. acidophilus, L. brevis, L. buchneri, L. delbureckii, L. fermentum, L. gasseri, L. paracasei, L. plantarum, L. reuteri, L. rhamnosus, and L. vaginalis), 10 different strains of Enterococcus species (E. avium, E. casseliflavus, E. cecorum, E. durans, E. faecalis, E. faecium, E. gallinarum, E. hirae, E. malodoratus, and E. mundtii), 7 different strains of Bifidobacterium species (B. animalis, B. bifidum, B. breve, B. longum, and B. pseudolongum), and 7 different strains of Weissella species (W. cibaria, W. confusa, W. halotolerans, W. hellenica, W. minor, W. paramesenteroides, and W. viridescens) cultured in MRS medium for 24 h at 37 °C. PolyP accumulation was observed in a wide variety of Lactobacillus strains, but not in Enterococcus strains except E. faecalis (Table ). Whereas L. brevis SBC8803 accumulated polyP at 1 nmol/mg protein, L. paracasei JCM 1163 did so at 13.4 nmol/mg protein. Recently, Alcántara et al. reported that 18 of 34 Lactobacillus strains accumulated polyP and that its accumulation was correlated with the presence of ppk genes. We also performed a tBLASTn search for genes homologous to Escherichia coli ppk1 and Pseudomonas aeruginosa ppk2 in the Lactobacillus and Enterococcus genomes deposited in the NCBI Microbial Genome database. ppk1 and ppk2 homologous genes were found in 10 and 7, respectively, of the 20 Lactobacillus genomes (using a cutoff E-value of 1 × e−20); none was found in 6 Enterococcus genomes. The lack of polyP in Enterococcus strains could be ascribed to the absence of ppk1 and ppk2. On the other hand, the abilities to accumulate polyP in the Lactobacillus strains were quite different. It is known that the polyP accumulation depends on not only PPK but also polyP-degrading enzyme (PPX) and Pi-uptake activities.Citation12,16) It is reported that the number of ppx gene is different in the Lactobacillus strains.Citation15) The wide variety of polyP-accumulating abilities of the Lactobacillus strains might be generated by the differences in their PPK, PPX, and Pi-uptake activities.
Table 1. PolyP production by lactic acid bacteria and bifidobacterium.
Chain length of polyP accumulated by L. paracasei JCM 1163
L. paracasei JCM 1163 accumulated the largest amount of polyP among the investigated strains. The chain length of polyP extracted from L. paracasei JCM 1163 was analyzed using polyacrylamide gel electrophoresis and found to be approximately 700 Pi residues (Fig. ). PolyP accumulated by E. coli is composed of two fractions (acid-soluble and alkali-soluble), whose chain lengths are different. The acid-soluble polyP fraction contained mainly low-molecular weight polyP with an average chain length between 17 and 20. On the other hand, the alkali-soluble fraction exhibited four separate peaks, with chain lengths ranging from 23 to >1000 Pi residues.Citation17) In this study, we found that the chain length of polyP accumulated by L. paracasei JCM 1163 was relatively long and homogenous (Fig. ).
Fig. 1. Chain length determination of polyP from L. paracasei JCM 1163 using polyacrylamide gel electrophoresis.
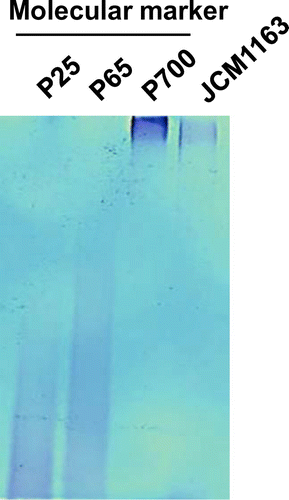
Measurement of polyP concentration in culture supernatant
The time course of polyP concentration in the L. paracasei cells and its culture supernatant in MRS medium at 37 °C was measured. PolyP concentration in the bacterial cells showed a steep increase during the early logarithmic growth phase, then underwent a gradual decrease up to the stationary phase, and finally reached undetectable levels (Fig. ). Alterations in the polyP concentration in the culture supernatant followed a similar pattern to that observed in the bacterial cells (Fig. ). However, the amounts of polyP in the culture supernatant were found to be only a few percent of those in the bacterial cells, suggesting that polyP is not actively excreted but rather simply leaked out from the cells.
PolyP overplus in L. paracasei JCM 1163
It is known that some bacteria and yeast accumulate polyP when Pi is added to Pi-starved cells. This phenomenon is called polyP overplus.Citation18) However, E. coli does not show polyP overplus.Citation16) To determine whether L. paracasei JCM 1163 shows polyP overplus, we modified the culture conditions to induce Pi-starvation. Since the peptone and meat extract in MRS medium contain a high concentration of Pi, we replaced these with a low-Pi SP medium. The Pi concentration in SP medium was extremely low (0.5–1 mM) compared to MRS medium (approximately 20 mM). When the Pi concentration in the SP medium reached less than 0.1 mM after 4 h incubation at 37 °C, we assumed that L. paracasei was starved for Pi. The addition of 500 mM Pi, but not 50 mM Pi, to the culture resulted in significant polyP accumulation after 6 h incubation (Fig. ). The addition of Pi to L. paracasei cultured on Pi-rich MRS medium was not effective (Fig. ). These results indicated that L. paracasei shows polyP overplus.
Fig. 3. PolyP production in Pi-starved L. paracasei JCM 1163 upon Pi supplementation.
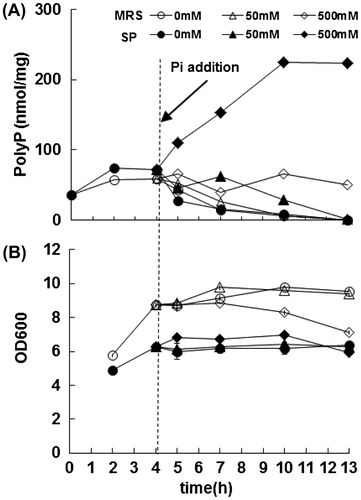
Improvement of polyP production ability in L. paracasei JCM 1163 by NTG treatment
PolyP overplus depends on Pi-starvation-inducible genes in Klebsiella aerogenes.Citation16) Since L. paracasei showed polyP overplus, we expected that a mutation of a phoU gene, a negative regulator of Pi-starvation-inducible genes,Citation19) might lead to polyP accumulation. To improve polyP production ability in L. paracasei JCM 1163, we tried to isolate phoU mutants that accumulate polyP. Importantly, phoU mutants also express alkaline phosphatase constitutively; therefore, they could be easily screened as blue-colored colonies on a Pi-rich agar medium containing 0.005% X-Pi. This method is not perfect, as some other mutants, such as pstSCAB, also show blue-colored colonies. However, it remains an easy and efficient way of screening polyP-accumulating mutants.Citation20,21)
Similar to the case of E. coli and other bacteria, L. paracasei JCM 1163 showed white-colored colonies on 3 mM Pi-supplemented SCDM and blue ones on Pi-depleted SCDM containing 0.005% X-Pi (data not shown). We therefore used NTG treatment to obtain mutants that show blue-colored colonies on Pi-rich MRS medium. Expectedly, some of these mutants accumulated more polyP than wild-type strain. We selected a mutant No. 14 that accumulated polyP at a level of 60 nmol/mg protein (Fig. (A)). The mutant No. 14 was further treated with NTG and a resultant mutant, named No. 14–42, accumulated polyP at a level of 1500 nmol/mg protein, approximately 100-fold of the wild-type strain (Fig. (B)).
Fig. 4. PolyP production by NTG-treated L. paracasei JCM 1163.
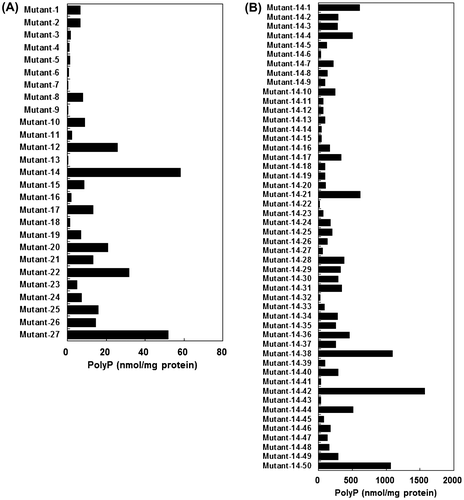
In our previous study, intrarectal administration of 10 μg polyP significantly ameliorated gastrointestinal inflammation caused by 4% sodium dextran sulfate.Citation9) In this study, L. brevis SBC8803 accumulated polyP at 0.8 nmol/mg protein. Lyophilized lactic acid bacteria contained 20–30% protein (data not shown). Considering that the molecular weight of Pi is approximately 100 and the protein content of lyophilized lactic acid bacteria is approximately 20%, then 625 mg of lyophilized L. brevis SBC8803 is needed to obtain an equivalent polyP dose. This means that an extraordinary amount of 30 g lyophilized lactic acid bacteria/kg of mouse body weight is needed for such an effect. The L. paracasei JCM1163 mutant accumulated polyP at 1500 mmol/mg protein—approximately 1900 times the amount accumulated by L. brevis SBC8803. The calculated amount of lyophilized mutant strain expected to exert an equivalent physiological effect is 150 mg/kg of mouse body weight (3 mg/mouse), which is a more reasonable dose for probiotic ingestion.
Rescue of intestinal barrier function with polyP accumulated by lactic acid bacteria
In our previous study, administration of 10 μg/mL polyP, which was synthesized using PPK, significantly inhibited oxidant-induced (0.3 mM NH2Cl) increase in mucosal permeability in mouse small intestinal loops.Citation9) Therefore, we tested whether polyP of L. paracasei JCM 1163 also inhibits the mucosal permeability caused by oxidative stress. As shown in Fig. , 0.1 μM polyP extracted from L. paracasei JCM 1163 completely inhibited transmural [3H]-mannitol fluxes in the small intestinal loop caused by oxidative stress.
Fig. 5. Rescue of intestinal barrier function with polyP accumulated by lactic acid bacteria.
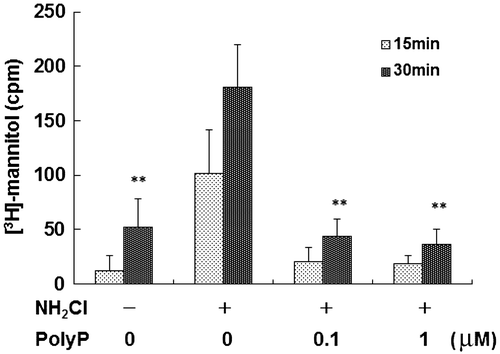
In summary, the present study shows that the ability to accumulate polyP in lactic acid bacteria is highly variable among different strains. Among the investigated strains, L. paracasei JCM 1163 was found to accumulate a large amount of polyP. We succeeded in obtaining the high polyP-accumulating mutant. PolyP extracted from L. paracasei JCM 1163 suppressed the oxidant-induced intestinal permeability in mouse small intestine. The amount of polyP accumulated in 3 mg of the mutant strain is equivalent to that required to increase survival rates in sodium dextran sulfate-administered mice. Thus, the mutant strain could become a candidate probiotic for enhancing intestinal barrier function and could have a beneficial effect on the human gastrointestinal tract. However, the amount of polyP released from cells was found to be relatively low compared to intracellular polyP. Therefore, we need to confirm that polyP could be released from cells when orally ingested lactic acid bacteria are exposed to digestive juices such as gastric acid and bile acid. Evaluating the preventive effect of oral administration of the high polyP-accumulating L. paracasei JCM 1163 mutant on gastrointestinal disorders is necessary for the development of future therapeutic.
Author contributions
A.K. and S.S. designed the study and wrote the manuscript. A.S. and Y.I performed the experiments and contributed to the development of the manuscript. R.H. and T.N. supervised the experiments and contributed to the development of the manuscript. All authors have read and approved the final manuscript.
Disclosure statement
No potential conflict of interest was reported by the authors.
Notes
Abbreviations: polyP, polyphosphate; Pi , phosphate; HSP, heat-shock protein; MRS, de Man–Rogosa–Sharpe; SP, soy peptide HK; SCDM, simplified chemically defined medium; GITC, guanidine isothiocyanate; SDS, sodium dodecyl sulfate; ATP, adenosine triphosphate; PPK, polyphosphate kinase; ADP, adenosine diphosphate; NTG, nitrosoguanidine; X-Pi, 5-bromo-4-chloro-3-indolyl-phosphate.
References
- Hooper LV, Gordon JI. Commensal host-bacterial relationships in the gut. Science. 2011;292:1115–1118.
- De Keersmaecker SC, Verhoeven TL, Desair J, et al. Strong antimicrobial activity of Lactobacillus rhamnosus GG against Salmonella typhimurium is due to accumulation of lactic acid. FEMS Microbiol. Lett. 2006;259:89–96.10.1111/fml.2006.259.issue-1
- Ayabe T, Satchell DP, Wilson CL, et al. Secretion of microbicidal α-defensins by intestinal Paneth cells in response to bacteria. Nat. Immunol. 2000;1:113–118.10.1038/77783
- Vaishnava S, Behrendt CL, Ismail AS, et al. Paneth cells directly sense gut commensals and maintain homeostasis at the intestinal host-microbial interface. Proc. Natl. Acad. Sci. USA. 2008;105:20858–20863.
- Belkaid Y, Oldenhove G. Tuning microenvironments: induction of regulatory T cells by dendritic cells. Immunity. 2008;29:362–371.10.1016/j.immuni.2008.08.005
- Zyrek AA, Cichon C, Helms S, et al. Molecular mechanisms underlying the probiotic effects of Escherichia coli Nissle 1917 involve ZO-2 and PKCζ redistribution resulting in tight junction and epithelial barrier repair. Cell Microbiol. 2007;9:804–816.10.1111/cmi.2007.9.issue-3
- Kulaev IS, Vagabov V, Kulakovskaya T. The biochemistry of inorganic polyphosphates, 2nd ed. Chichester: John Wiley & Sons; 2004.
- Kornberg A. Inorganic polyphosphate: toward making a forgotten polymer unforgettable. J. Bacteriol. 1995;177:491–496.
- Segawa S, Fujiya M, Konishi H, et al. Probiotic-derived polyphosphate enhances the epithelial barrier function and maintains intestinal homeostasis through integrin–p38 MAPK pathway. PLoS ONE. 2011;6:e23278.10.1371/journal.pone.0023278
- Rao NN, Gómez-García MR, Kornberg A. Inorganic polyphosphate: essential for growth and survival. Annu. Rev. Biochem. 2009;78:605–647.10.1146/annurev.biochem.77.083007.093039
- Brown MR, Kornberg A. The long and short of it – polyphosphate, PPK and bacterial survival. Trends Biochem. Sci. 2008;33:284–290.10.1016/j.tibs.2008.04.005
- Harold FM. Inorganic polyphosphates in biology: structure, metabolism, and function. Bacteriol. Rev. 1966;30:772–794.
- Hebert EM, Raya RR, De Giori GS. Nutritional requirements and nitrogen-dependent regulation of proteinase activity of Lactobacillus helveticus CRL 1062. Appl. Environ. Microbiol. 2000;66:5316–5321.10.1128/AEM.66.12.5316-5321.2000
- Ault-Riché D, Fraley CD, Tzeng CM, et al. Novel assay reveals multiple pathways regulating stress-induced accumulations of inorganic polyphosphate in Escherichia coli. J. Bacteriol. 1998;180:1841–1847.
- Alcantara C, Blasco A, Zuniga M. Accumulation of polyphosphate in Lactobacillus spp. and its involvement in stress resistance. Appl. Environ. Microbiol. 2014;80:1650–1659.10.1128/AEM.03997-13
- Kuroda A, Ohtake H. Molecular analysis of polyphosphate accumulation in bacteria. Biochemistry (Moscow). 2000;65:304–308.
- Rao NN, Roberts MF, Torriani A. Amount and chain length of polyphosphates in Escherichia coli depend on cell growth conditions. J. Bacteriol. 1985;162:242–247.
- Kuroda A, Murphy H, Cashel M, et al. Guanosine tetra- and pentaphosphate promote accumulation of inorganic polyphosphate in Escherichia coli. J. Biol. Chem. 1997;272:21240–21243.10.1074/jbc.272.34.21240
- Wanner BL. Gene regulation by phosphate in enteric bacteria. J. Cell Biochem. 1993;51:47–54.10.1002/(ISSN)1097-4644
- Morohoshi T, Maruo T, Shirai Y, et al. Accumulation of inorganic polyphosphate in phoU mutants of Escherichia coli and Synechocystis sp. strain PCC6803. Appl. Environ. Microbiol. 2002;68:4107–4110.10.1128/AEM.68.8.4107-4110.2002
- Morohoshi T, Yamashita T, Kato J, et al. A method for screening polyphosphate-accumulating mutants which remove phosphate efficiently from synthetic wastewater. J. Biosci. Bioeng. 2003;95:637–640.10.1016/S1389-1723(03)80177-9