Abstract
Based on the finding of a novel berberine (BBR)-utilizing bacterium, Rhodococcus sp. strain BD7100, we investigated the degradation of BBR and its analog berberrubine (BRU). Resting cells of BD7100 demethylenated BBR and BRU, yielding benzeneacetic acid analogs. Isolation of benzeneacetic acid analogs suggested that BD7100 degraded the isoquinoline ring of the protoberberine skeleton. This work represents the first report of cleavage of protoberberine skeleton by a microorganism.
Graphical abstract
BBR-utilizing bacterium, Rhodococcus sp. BD7100 demethylenated BBR and BRU, yielding benzeneacetic acid analogs in which their protoberberine skeletons were cleaved.
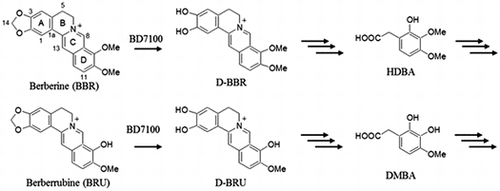
Berberine is a quaternary benzylisoquinoline alkaloid with a protoberberine skeleton. Whereas alkaloids exhibit relatively limited distribution in the plant kingdom, BBR is more widely produced in higher plants (e.g. in the roots of Coptis japonica, the leaves of Berberis fremonti, and the stem bark of Mahonia aquifolum).Citation1–3) Plant materials containing BBR have long been used as traditional medicines around the world.Citation4) BBR shows many pharmacological activities, including antidiarrheal,Citation5,6) antibacterial,Citation7,8) antifungal,Citation8,9), and anticancer properties.Citation10–13) Furthermore, BBR has been the subject of clinical trials for activity against several important diseases such as metabolic syndrome and diabetes.Citation14)
BBR analogs such as palmatine (PAL) and berberrubine (BRU) also have been investigated for their biological activities. PAL (a 2,3-methoxylated BBR derivative) has been shown to protect cardiac tissue from ischemia-reperfusion injury in rats.Citation15) BRU exhibits anti-HIV activity in CEM-GFP cells.Citation16) In the present work, we have attempted to obtain new derivatives of BBR and related compounds. To facilitate the production of new derivatives, we isolated two BBR-utilizing microbes from the soil. These bacteria were identified as Sphingobium sp. strain BD3100 and Rhodococcus sp. strain BD7100.Citation17) There is a report about BBR degradation by bacteria in sludge of chemical synthetic pharmaceutical company in lab-scale wastewater treatment process.Citation18) However, the isolation of these bacteria was first report of BBR-utilizing bacterium. Characterization of BD3100 revealed that this bacterium degraded BBR and PAL, while also producing 11-hydroxyberberine (H-BBR) and 11-hydroxydemethyleneberberine (HD-BBR) from BBR and 11-hydroxypalmatine (H-PAL) from PAL.
In the present report, we investigated the degradation of BBR and BRU by BD7100. Our investigation revealed that BD7100 degraded BBR and BRU via a pathway that resulted in the production of benzenacetic acid derivatives, thus employing a mechanism distinct from that of BD3100.
Material and methods
Chemicals and reagents
BBR sulfate, BBR chloride, palmatine chloride, and other chemicals were purchased from Tokyo Chemical Industry Co., Ltd. (Tokyo, Japan), Wako Pure Chemical Industries, Ltd. (Osaka, Japan), or LKT Laboratories (St. Paul, USA). Demethyleneberberine (D-BBR) and BRU were synthesized according to the previously reported methods, and 1H and 13C NMR of the synthesized compounds were compared with literature values.Citation19,20) Demethyleneberberrubine (D-BRU) was chemically synthesized as described in Supplemental Information and Figs. S1–S4.
Experimental instruments
UV and IR spectra were recorded on an Ultrospec 2100 pro UV–visible spectrophotometer (Amersham Biosciences, Ltd., Tokyo, Japan) and a FT/IR-4100 instrument (JASCO Co., Ltd., Tokyo, Japan), respectively. 1H and 13C NMR spectra were recorded using a Bruker AVANCE-400 spectrometer (400.13 MHz for 1H, 100.61 MHz for 13C; Bruker Biospin K. K., Kanagawa, Japan). Chemical shifts (δ) were measured in ppm using tetramethylsilane as an internal standard. BioShakers BR43-FH (TAITEC Co., Ltd., Saitama, Japan) and FTW-502 M (Hirayama Manufacturing Corp., Saitama, Japan) were used for incubation of bacteria. The turbidity of cultures was monitored using an OD-MonitorA&S (TAITEC Co.). The quantification and analysis of samples were performed by PDA-HPLC using a PU-980 and PU-1580 pump (flow rate 1 mL/min; JASCO Co., Ltd., Tokyo, Japan) and Inertsil ODS-3 column (5 μm, 4.6 mm × 250 mm; GL Science Inc., Tokyo, Japan) maintained at a temperature of 30 °C using a CO-2065 Plus column oven (JASCO Co.) and equipped with a MD-2010 Plus photodiode array (JASCO Co.). BBR and BRU metabolites were isolated using a LC-20AT prominence pump (Shimadzu Corp., Kyoto, Japan) and a Inertsil ODS-P column (5 μm, 10 mm × 250 mm; GL Science Inc.) maintained at a temperature of 30 °C using a CO-965 column oven (JASCO Co., Ltd., Tokyo, Japan) and equipped with a SPD-20A UV detector (Shimadzu Corp.). Determination of molecular weight and molecular formula was performed by LC-ESI-TOF-MS using a JMS-T100LP (JEOL, Ltd., Tokyo, Japan) and a 1200 series system (Agilent Technologies, Inc., Tokyo, Japan).
Strains and culture conditions
The isolation (from the soil of a dumping site at an herbal medicine factory) and identification of Sphingobium sp. BD3100 and Rhodococcus sp. strain BD7100 have been described previously.Citation17) For the present work, these bacteria were incubated in Luria-Bertani (LB) medium (containing 0.5 g of Bacto Yeast Extract, 1.0 g of Bacto Tryptone, and 0.5 g of sodium chloride in 100 mL of water); at 0.1 × LB agar medium [containing Bacto Yeast Extract and Bacto Tryptone at 10-fold lower levels and 1.5% (w/v) agar]. BBR was added to these media as indicated.
PDA-HPLC analysis conditions
Flow rate was 1 mL/min and the column oven temperature was 30 °C. Analysis was performed using a gradient system of the mobile phase as follows. Initial conditions were 15% B with linear gradient to 50% B from 5 to 15 min, followed by a linear gradient to 100% B at 15.1 min; then this proportion was maintained until 18 min. The mobile phase was returned to initial conditions at 18.1 min and maintained until the end of the run at 25 min. Mobile solvents A and B corresponded to H2O containing 0.1% trifluoroacetic acid (TFA) and acetonitrile, respectively.
LC-ESI-TOF-MS analysis conditions
HPLC was performed with flow rate of 0.2 mL/min and mobile solvent of 100% MeOH.
Investigation of optimal culture conditions for BD7100
BD7100 was grown at 30 °C on 0.1 × LB medium agar plates containing 1.0 mM BBR. Individual colonies of BD7100 were used to inoculate 10 mL of LB medium, and the resulting cultures were incubated for 24 h at 30 °C on a rotary shaker (180 rpm). Aliquots (1 mL) of this seed culture were used to inoculate separate 100 mL of LB medium (pH 7.0), and the resulting cultures were incubated at 25, 30, 35, or 37 °C to investigate optimal growth temperature. Optimal initial pH was investigated using equivalent cultures of LB medium in which the pH had been adjusted by adding 4 N hydrochloric acid (HCl) or 4 N NaOH; then these cultures then were incubated at 30 °C on a rotary shaker (180 rpm).
The turbidities (OD600) of the resulting cultures were monitored at intervals of 3 h for 24 h. The OD600 values were plotted against the incubation time to generate the growth curves.
Growing-cell assay of BD7100 in LB medium
Seed cultures of BD7100 were prepared as described above. Aliquots (1 mL) of the seed culture were inoculated into separate 100 mL of LB medium containing 1.0 mM BBR. The resulting cultures were incubated under the same conditions as perfomed for the respective seed culture. The turbidities of cultures were monitored as described above. Aliquots were harvested at intervals of 2–6 h and growth was quenched by combining with 100 mL of MeOH containing 0.05 N HCl. Immediately following quenching, samples were centrifuged (18,800 × g, 5 min, 4 °C), and the resulting supernatants were assayed for the amount of BBR and D-BBR using PDA-HPLC.
Preparation of resting cells of BD7100
Seed cultures of BD7100 were prepared as described above. Aliquots (1 mL) of the seed culture were inoculated into separate 100 mL of LB medium without (induction free) or with 1.0 mM BBR (BBR induction). The resulting cultures were incubated under the same conditions as used for the respective seed culture. After 24 h of incubation, cells were harvested by centrifugation (2610 × g, 15 min, room temperature); the resulting pellets were washed twice with 50 mM phosphate buffer (pH 7.0) and then resuspended in phosphate buffer for use in the resting-cell assay.
The resting-cell assay of BD7100
The turbidities of suspension of resting cells were adjusted to OD600 values of 5.0 in 20 mL of 50 mM phosphate buffer (pH 7.0). Individual aliquots were supplemented with degradation substrates to yield final concentrations of 1.0 mM BBR, 0.5 mM PAL, or 0.5 mM BRU, then incubated with shaking (180 rpm) at 30 °C. At the indicated time points, 500-μL samples were withdrawn, combined with an equal volume of MeOH containing 0.05 N HCl (to quench the degradation reactions), and centrifuged (18,800 × g, 15 min, 4 °C) to remove cells and cell debris. The resulting supernatants were assessed by PDA-HPLC.
Isolation of metabolites using preparative HPLC
Metabolites of BBR and BRU were prepared from the degradation of 1.0 mM BBR or 0.5 mM BRU by the resting-cell assay using resting cells grown in LB medium containing BBR. The degradation conditions by resting cells were described in above. After 1.5 h (for D-BRU) or 3 h (for HDBA and DMBA) of incubation, degradation reaction was stopped by adding 20 mL of MeOH. Cells and cells debris were removed by centrifugation (2610 × g, 15 min, room temperature). The resulting supernatants were filtered and evaporated at 40 °C under reduced pressure. The remaining residues were dissolved in MeOH, and the solutions were chromatographed to isolate 2-hydroxy-3,4-dimethoxybenzeneacetic acid (HDBA), 2,3-dihydroxy-4-methoxybenzeneacetic acid (DMBA), and D-BRU using HPLC under conditions as follows: flow rate, 2.0 mL/min; column oven temperature, 30 °C; UV detection at 254 nm; mobile solvent, H2O containing 0.1% (v/v) TFA (A) and acetonitrile (B). The gradient system of mobile phase for isolation of metabolites was as follows: initial conditions were 20% (for DMBA and D-BRU) or 25% (for HDBA) B with linear gradient to 40% B from 0 to 15 min, followed by linear gradient to 100% B at 15.1 min; this proportion was maintained until 18 min. The mobile phase was returned to initial conditions starting at 18.1 min and continuing until the end of the run (25 min).
2-hydroxy-3,4-dimethoxybenzeneacetic acid
Colorless amorphous; UV λmax (MeOH) nm (log ε): 210 (4.2), 275 (3.1); IR (KBr) cm−1: 3422, 1685; HRESIMS (negative) m/z: 211.0626 ([M]− calcd. for C10H11O5 1.0607) 211.0626. NMR data are shown in Figs. S5–S8 and Table S1.
2,3-dihydroxy-4-methoxybenzeneacetic acid
Colorless amorphous; UV λmax (MeOH) nm (log ε): 211 (4.2), 268 (2.9); IR (KBr) cm−1: 3418, 1682; HRESIMS (negative) m/z: 197.0457 (M]− calcd. for C9H9O5, 197.0450). NMR data are shown in Figs. S6–S9 and Table S1.
Antibacterial assay
Antibacterial assay was performed using the paper disk method and Staphylococcus aureus strain H209P. Defined quantities (50, 125, 250, or 500 nmol) of test compounds (BBR, D-BBR, BRU, D-BRU, HDBA, or DMBA) were placed on each disk. The disks were placed on LB agar plates that had been inoculated with bacteria, and the plates were incubated at 30 °C. After 12 h of incubation, the zones of inhibition (the diameter measured in millimeters) were recorded.
Results
Optimization of growth condition of BD7100 in LB medium
To optimize the growth conditions of BD7100, the optimum growth temperature was examined at 25, 30, 35, and 37 °C in LB medium (pH 7.0). BD7100 grew well at 25 and 30 °C, but did not grow at 35 and 37 °C (Fig. (A)). Next, the optimum initial pH condition was examined at pH 4.1–10.1 at 30 °C in LB medium (Fig. (B)). BD7100 actively grew at initial pH 6.2–8.2, whereas the growth was slower at initial pH 5.3 and pH 9.2, and did not grow at initial pH 4.1 and pH 10.1. Therefore, subsequent experiments were performed in LB medium at pH 7.0 and 30 °C.
Fig. 1. Effect of initial medium conditions (temperature and pH) on the growth of BD7100.
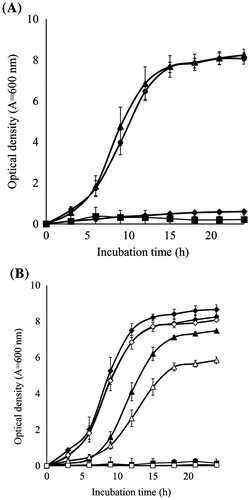
Degradation of BBR by growing cells of BD7100
First, degradation of BBR was measured in growing cells of BD7100 in LB medium containing 1.0 mM BBR. BD7100 cells exhibited logarithmic growth after 6 h of culturing, and reached a maximum OD600 at 30 h of incubation (Fig. (A)). PDA-HPLC analysis revealed that BBR levels fell during growth of BD7100 cells; the initial 1.0 mM BBR level was almost completely exhausted by 23 h of incubation. As shown in Fig. (B), a new peak (tR = 15.4 min) was observed on the PDA-HPLC chromatogram after 19 h of incubation, concomitant with the depletion of BBR (tR = 17.7 min). This new peak was identified as D-BBR by the direct comparison of the tR values with that of a chemically synthesized standard (tR = 15.4 min). D-BBR levels increased concomitantly with the decrease in BBR levels, with D-BBR reaching a peak of 0.8 mM at 21 h of incubation (Fig. (A)). Subsequently, D-BBR levels fell, and this compound was no longer detectable at 36 h of incubation. In this growing-cell assay, other BBR metabolites were not detected on PDA-HPLC chromatograms.
Fig. 2. The growth of BD7100 and BBR degradation in the growing-cell assay.
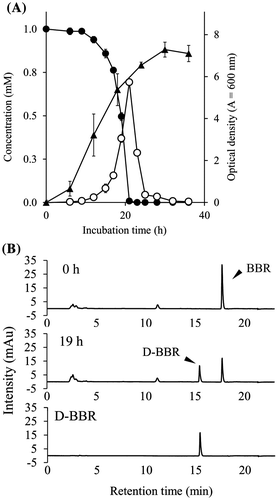
Degradation of BBR by resting cells of BD7100
To detect other metabolites of BBR, we incubated BBR with resting cells of BD7100. Degradation of BBR and its metabolites was not observed on PDA-HPLC chromatograms in the absence of BBR induction (Fig. (A)). However, BBR-induced cells of BD7100 degraded BBR to D-BBR. D-BBR levels rose concomitantly with the depletion of BBR, with D-BBR levels peaking at 0.1 mM at 120 min. Subsequently, D-BBR (tR = 15.4 min) levels gradually fell; an unknown peak with similar retention time (tR = 15.3 min) was detected in the phosphate buffer incubated for 180 min (Fig. (B)). In UV absorption spectrum, the new peak (tR = 15.3 min) did not exhibit absorption at 340 nm, presumably derived from the protoberberine skeleton; this compound differed from protoberberine alkaloids such as BBR and D-BBR (Fig. (C)). Isolation of the peak by preparative HPLC permitted structural determination by 1D- (1H and 13C NMR) and 2D NMR spectra (Fig. (D), Table S1 and Figs. S5–S8), revealing that the metabolite was HDBA.
Fig. 3 The degradation of BBR by resting cells of BD7100 and the resulting metabolites.
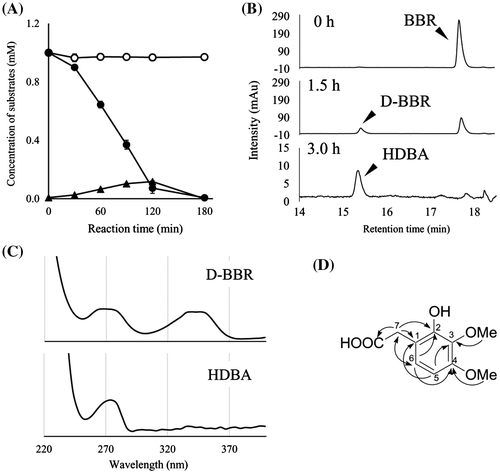
Degradation of BRU and PAL by resting cells of BD7100
Using resting cells, degradation of other BBR analogs, BRU, and PAL, also was examined. As with BBR, BRU was not degraded by resting cells in the absence of BBR induction, (Figs. (A) and (B)). When induced resting cells were incubated with BRU at an initial concentration of 0.5 mM, BRU levels gradually decreased until BRU (tR = 16.9 min) was no longer detected at 180 min. There was a concomitant accumulation of a novel peak (tR = 14.2 min); detailed analysis of ESI-MS and NMR spectra revealed that the new metabolite was D-BRU (Figs. S15 and S16). Levels of D-BRU peaked at 0.03 mM at 90 min of incubation before subsequently falling, until D-BRU was no longer detectable after 180 min. In the same interval, some unknown peaks were detected at 180 min of incubation (Fig. (B)). In contrast to D-BRU, the one peak of these peaks (tR = 11.9 min) did not exhibit UV absorption at 340 nm (Fig. (C)). Detailed analysis using ESI-MS and NMR spectrometry including 2D NMR revealed a structure of DMBA (Fig. (D), Table S1 and Figs. S9–S12). On the other hand, PAL was not degraded when incubated with either induction-free or BBR-induced cells (Fig. S17).
Fig. 4. The degradation of BRU by resting cells of BD7100 and the resulting metabolites.
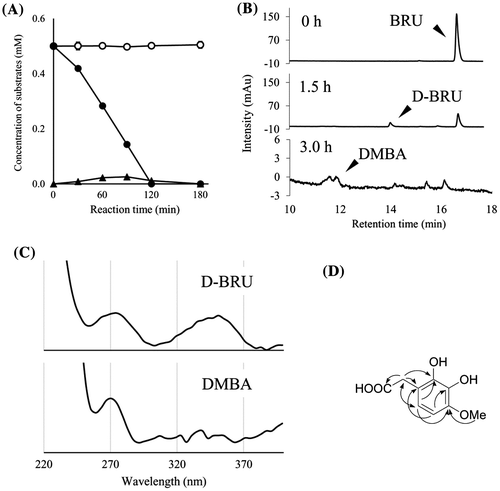
Antibacterial activities
Antibacterial activities of BBR, its analogs, and metabolites (D-BBR, BRU, D-BRU, HDBA and DMBA) were tested using the paper disk method. Growth of S. aureus was inhibited semiquantitatively in the presence of disks containing BBR at 125 (11 mm), 250 (12 mm), or 500 (15 mm) nmol; none of the analogs or metabolites exhibited antibacterial activity against S. aureus. These results suggest that the methylenedioxy ring of BBR is critical for antibacterial activity against Staphylococcus.
Discussion
In this study, we determined that the optimal conditions for the growth of Rhodococcus sp. strain BD7100 in culture with BBR were 30 °C and initial pH 7.0 in LB medium. Growing cells of BD7100 were able to quantitatively convert BBR to D-BBR within the first 20 h of incubation, but other metabolites were not observed through the first 36 h of culturing under these conditions. Therefore, resting cells-induced BBR-degradation enzymes, by exposure to BBR, were assessed for degradation of BBR, revealing the production of HDBA. Similarly, incubation of BRU with BBR-induced cells of BD7100 resulted in the sequential production of D-BRU and DMBA. To our knowledge, this work represents the first report of the natural production of D-BRU. A previous report showed that D-BRU is an antagonist of dopamine D2 receptors.Citation21) In other work, HDBA and DMBA, which are relatively expensive reagents, were used for the chemical synthesis of protoberberine and isoflavonoid derivatives for drug development.Citation22–25) In the present work, the isolation of HDBA and DMBA strongly suggests that the respective parent substrates are being degraded by cleavage of the protoberberine skeleton; the structures of these two benzeneacetic acid analogs are similar to the D-rings of BBR and BRU, respectively. Thus, this work represents the first example of microbial cleavage of the protoberberine skeleton.
Two benzeneacetic acid analogs were detected upon incubation with BBR-induced cells of BD7100. Incubation of resting cells with other incubation conditions and/or substrates may provide bioconversion to generate other metabolites of BBR and its analogs.
In a previous report, we demonstrated that Sphingobium sp. strain BD3100 converted BBR and PAL to 11-hydroxylated analogs (H-BBR and HD-BBR) and H-PAL.Citation17) In contrast, BD7100 did not generate 11-hydroxylated BBR analogs at detectable levels, and BD7100 did not degrade PAL at all (Fig. ). These facts suggest that BD7100 does not harbor an enzyme capable of catalyzing 11-hydroxylation, or that such an activity was not expressed under the conditions used in this study.
Fig. 5. The inferred pathways for degradation of BBR and its analogs in BBR-utilizing bacteria.
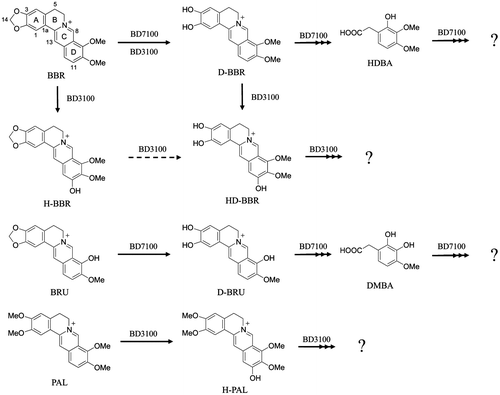
In both the present and previous studies, we successfully produced new compounds using BBR-utilizing bacteria. Thus, bioconversion using a degradative microbe might be an effective means of producing valuable compounds. However, the conversion rate of the BBR to HDBA reaction was low. In future studies, we propose to identify the enzymes involved in the BBR degradation pathway; optimization of the expression and/or activity of these enzymes is expected to provide improved techniques for the production of benzeneacetic acids and related compounds.
Author contributions
KI, HT, and TH conceived the experiments. KI and HT performed the experiments and analyzed the data together with DW, TH, and SF. HT, DW, FS, and TH provided valuable advice. HT and TH equally contributed to the manuscript writing. All authors discussed the results and commented on the manuscript.
Supplemental material
The supplemental material for this paper is available at http://dx.doi.org/10.1080/09168451.2015.1136878.
Disclosure statement
No potential conflict of interest was reported by the authors.
Acknowledgments
We also thank Der Deutsche Akademische Austauschdienst (DAAD) for support within the program ‘‘PAJAKO – Partnerschaften mit Japan und Korea.’’
20151211_supporting_materials_th_.ppt
Download MS Power Point (511.5 KB)Additional information
Funding
Notes
Abbreviations: BBR, berberine; BRU, berberrubine; D-BBR, demethyleneberberine; D-BRU, demethyleneberberrubine; DMBA, 2,3-dihydroxy-4-methoxybenzeneacetic acid; H-BBR, 11-hydroxyberberine; HDBA, 2-hydroxy-3,4-dimethoxybenzeneacetic acid; HD-BBR, 11-hydroxydemethyleneberberine; H-PAL, 11-hydroxypalmatine; LB, Luria-Bertani; PAL, palmatine.
References
- Jung HA, Yoon NY, Bae HJ, et al. Inhibitory activities of the alkaloids from Coptidis Rhizoma against aldose reductase. Arch. Pharm. Res. 2008;31:1405–1412.10.1007/s12272-001-2124-z
- Stermitz FR, Lorenz P, Tawara JN, et al. Synergy in a medicinal plant: antimicrobial action of berberine potentiated by 5′-methoxyhydnocarpin, a multidrug pump inhibitor. Proc. Natl. Acad. Sci. U.S.A. 2000;97:1433–1437.10.1073/pnas.030540597
- Volleková A, Košťálová D, Sochorová R. Isoquinoline alkaloids from Mahonia aquifolium stem bark are active against Malassezia spp. Folia Microbiol (Praha). 2001;46:107–111.10.1007/BF02873586
- Imanshahidi M, Hosseinzadeh H. Pharmacological and therapeutic effects of Berberis vulgaris and its active constituent, berberine. Phytother. Res. 2008;22:999–1012.10.1002/ptr.2399
- Sack RB, Froehlich JL. Berberine inhibits intestinal secretory response of Vibrio cholerae and Escherichia coli enterotoxins. Infect. Immun. 1982;35:471–475.
- Taylor CT, Baird AW. Berberine inhibition of electrogenic ion transport in rat colon. Br. J. Pharmacol. 1995;116:2667–2672.10.1111/bph.1995.116.issue-6
- Iwasa K, Kamigauchi M, Ueki M, Taniguchi M. Antibacterial activity and structure-activity relationships of berberine analogs. E. J. Med. Chem. 1996;31:469–478.10.1016/0223-5234(96)85167-1
- Slobodníková L, Kost’álová D,, Labudová D, et al. Antimicrobial activity of Mahonia aquifolium crude extract and its major isolated alkaloids. Phytother. Res. 2004;18:674–676.10.1002/(ISSN)1099-1573
- Volleková A, Kost’álová D, Kettmann V, et al. Antifungal activity of Mahonia aquifolium extract and its major protoberberine alkaloids. Phytother. Res. 2003;17:834–837.10.1002/(ISSN)1099-1573
- Inoue K, Kulsum U, Chowdhury SA, et al. Tumor-specific cytotoxicity and apoptosis-inducing activity of berberines. Anticancer Res. 2005;25:4053–4059.
- Lin CC, Kao ST, Chen GW, et al. Apoptosis of human leukemia HL-60 cells and murine leukemia WEHI-3 cells induced by berberine through the activation of caspase-3. Anticancer Res. 2006;26:227–242.
- Yu FS, Yang JS, Lin HJ, et al. Berberine inhibits WEHI-3 leukemia cells in vivo. In Vivo. 2007;21:407–412.
- Kettmann V, Kosfálová D, Jantová S, et al. In vitro cytotoxicity of berberine against HeLa and L1210 cancer cell lines. Pharmazie. 2004;59:548–551.
- Kumar A, Ekavali, KC, Mukherjee M, et al. Current knowledge and pharmacological profile of berberine: an update. Eur. J. Pharmacol. 2015;761:288–297.10.1016/j.ejphar.2015.05.068
- Kim YM, Ha YM, Jin YC, et al. Palmatine from Coptidis rhizoma reduces ischemia-reperfusion-mediated acute myocardial injury in the rat. Food Chem. Toxicol. 2009;47:2097–2102.10.1016/j.fct.2009.05.031
- Bodiwala HS, Sabde S, Mitra D, et al. Synthesis of 9-substituted derivatives of berberine as anti-HIV agents. Eur. J. Med. Chem. 2011;46:1045–1049.10.1016/j.ejmech.2011.01.016
- Takeda H, Ishikawa K, Wakana D, et al. 11-hydroxylation of protoberberine in the novel berberine-utilizing aerobic bacterium, Sphingobium sp. Strain BD3100. J. Nat. Prod. 2015;78:2880–2886.
- Qiu G, Song Y, Zeng P, et al. Combination of upflow anaerobic sludge blanket (UASB) and membrane bioreactor (MBR) for berberine reduction from wastewater and the effects of berberine on bacterial community dynamics. J Hazard. Mater. 2013;246–247:34–43.10.1016/j.jhazmat.2012.12.010
- Li YH, Li Y, Yang P, et al. Design, synthesis, and cholesterol-lowering efficacy for prodrugs of berberrubine. Bioorg. Med. Chem. 2010;18:6422–6428.10.1016/j.bmc.2010.06.106
- Spinozzi S, Colliva C, Camborata C, et al. Berberine and its metabolites: relationship between physicochemical properties and plasma levels after administration to human subjects. J. Nat. Prod. 2014;77:766–772.10.1021/np400607k
- Liang X, Wang C, Zhang X, et al. United States patent US 20150164870 A1. 2015.
- Kametani T, Okubo K. Capaurimine and related compounds. 3. Synthesis of dl-5,6,13,13a-tetrahydro-1,2,3,10,11-pentamethoxy-8H-dibenzo[a, g]quinolizine and dl-5,6,13,13a-tetrahydro-2,3,10,11,12-pentamethoxy-8H-dibenzo[a, g]quinolizine (studies on the syntheses of heterocyclic compounds. 286). Yakugaku Zasshi. 1969;89:279–282.
- Adesomoju AA, Davis WA, Rajaraman R, et al. Total synthesis of leucoxylonine. J. Org. Chem. 1984;49:3220–3222.10.1021/jo00191a037
- Kranich R, Busemann AS, Bock D, et al. Rational design of novel, potent small molecule pan-selectin antagonists. J Med. Chem. 2007;50:1101–1115.10.1021/jm060536g
- Berry RC, Eade RA, Simes JJH. Extractives of Australian timbers. XVII. The isolation, structure and synthesis of koparin (7, 2′, 3′-trihydroxy-4′-methoxyisoflavone). Aust. J. Chem. 1977;30:1827–1835.10.1071/CH9771827