Abstract
To identify the major mutagen in pyroligneous acid (PA), 10 wood and 10 bamboo pyroligneous acids were examined using the Ames test in Salmonella typhimurium strains TA100 and TA98. Subsequently, the mutagenic dicarbonyl compounds (DCs), glyoxal, methylglyoxal (MG), and diacetyl in PA were quantified using high-performance liquid chromatography, and the mutagenic contribution ratios for each DC were calculated relative to the mutagenicity of PA. Eighteen samples were positive for mutagens and showed the strongest mutagenicity in TA100 in the absence of S9 mix. MG had the highest mutagenic contribution ratio, and its presence was strongly correlated with the specific mutagenicity of PA. These data indicate that MG is the major mutagen in PA.
Graphical abstract
Pyroligneous acids showed the mutagenicity by the Ames test, and methylglyoxal was identified as a major mutagen.
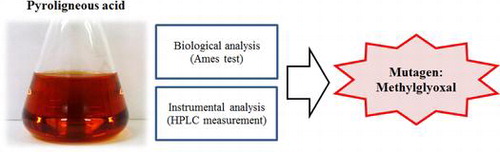
Pyroligneous acid (PA) is obtained by cooling the smoke that is generated during the carbonization of wood and is a predominant by-product of charcoal production in Japan. It is known that PA has bactericidal,Citation1) antiviral,Citation2) antifungal,Citation3) and odor maskingCitation4) activities because it contains multiple compounds, such as organic acids, phenols, and carbonyl compounds.Citation5,6) Accordingly, PA is sold at gardening stores as an agricultural antiseptic and soil improvement agent and is sold at drugstores as a bath agent. In addition, PA is used as a food additive to give a smoked flavor to foodstuffs.Citation7,8) However, several studies show that PA was mutagenic in the Ames tests using Salmonella typhimurium strains TA100 and TA98.Citation9) Furthermore, these studies have produced diverse results, and the primary mutagen in PA has not been sufficiently identified.Citation10–13) Nonetheless, because wood smoke has been shown to be mutagenic,Citation14) it can be assumed that the major mutagen in PA is formed during carbonization.
Similar to PA, roasted coffee beans are produced by a high-temperature heating process and contain mainly carbohydrate as raw materials. It had been reported that the mutagens of coffee beans were produced during the roasting process.Citation15) Roasted coffee beans showed mutagenicity in the TA100 in the absence of S9 mix,Citation16) and methylglyoxal (MG) was shown to be the primary mutagen.Citation17) MG is one of the several dicarbonyl compounds (DCs) that are formed during decomposition of carbohydrates.Citation18,19) DCs have demonstrated mutagenicity in TA100Citation20) and are likely mutagenic components of PA because the D-glucose polymer cellulose is a major constituent of wood.
Most studies of PA mutagenicity in S. typhimurium have been conducted on smoke condensates from wood. In Japan, both wood pyroligneous acid (WPA) and bamboo pyroligneous acid (BPA) are commercially available and have been sold in equal proportions. In this study, the mutagenicity of 10 WPAs and 10 BPAs were examined using the Ames test in TA100 or TA98, and furthermore, MG, glyoxal (G), and diacetyl (DA) in WPAs and BPAs were quantified using high-performance liquid chromatography (HPLC). The involvement of each DC in the mutagenicity of PA was then investigated, and the major PA mutagen was determined.
Materials and methods
PA samples
WPAs and BPAs were purchased as liquids from gardening or drug stores, and WPA and BPA samples were denoted W-1–W-10 and B-1–B-10, respectively.
Reagents
G and MG were purchased from Sigma-Aldrich Corp. (St. Louis, MO, USA). DA, quinoxaline, 2-methyl-quinoxaline, 2,3-dimethyl-quinoxaline, and 1,2-phenylenediamine were purchased from Tokyo Chemical Industry Co., Ltd. (Tokyo, Japan). HPLC grade methanol and formic acid were purchased from Kanto Chemical Co., Inc. (Tokyo, Japan). S9 was purchased from Kikkoman Biochemifa Company (Tokyo, Japan).
Instrument
The C18 cartridge Sep-Pak Plus was purchased from Waters Corporation (Milford, MA, USA), and the automatic colony counter MCL-2000 was purchased from SK-Electronics CO. Ltd. (Kyoto, Japan). The HPLC system (Shimadzu Corporation, Kyoto, Japan) was equipped with a system controller (CBM-20A), a pump (LC-20AD), a diode array detector (SPD-M20A), an auto sampler (SIL-20AC), a column oven (CTO-20AC), and an Inertsil ODS-4 column (5 μm particle size, 4.6 mm i.d. × 250 mm, GL Sciences Inc., Tokyo, Japan).
Mutagenicity test
The Ames test was performed according to a previously described preincubation methodCitation21) using S. typhimurium strains TA100 and TA98 in the absence and presence of S9 mix. Both strains were kindly provided by Dr Bruce N. Ames, University of California, Berkeley, California. The S9 mix contained 50 μL of S9, which was prepared from the livers of male Sprague Dawley rats by treatment with phenobarbital and 5,6-benzoflavone in a total volume of 500 μL. Revertants were counted using a colony counter after incubation for 48 h at 37 °C, and mutagenicity tests were performed in more than 2 plates. Distilled water was used as a negative control, and 2-(2-furyl)-3-(5-nitro-2-furyl)acrylamide or 2-aminoanthracene was used as positive controls. PA mutagenicity was recorded as positive when PA samples induced more than twice the number of negative control revertants. Specific mutagenicity values of PA represented net revertants per 1 μL of sample and were calculated using dose–revertant response curves.
Determination of DCs in PA
G, MG, and DA in PA were derivatized to quinoxaline, 2-methylquinoxaline, and 2.3-dimethylquinoxaline, respectively, using 1,2-phenylenediamine in a modified quinoxaline derivatization method.Citation22) Briefly, PA was diluted 10-fold with 0.2 M phosphate buffer solution (pH 8.0) and 2 mL of diluted PA was added to 2 mL of 0.3 M 1,2-phenylenediamine solution containing 0.2 M phosphate buffer (pH 8.0) and was allowed to react for 20 min at 37 °C. After cooling to room temperature, distilled water was added to a final volume of 10 mL. Subsequently, 5 mL aliquots were loaded onto a Sep-Pak Plus C18 cartridge that had been conditioned with 5 mL of methanol and 5 mL of distilled water and then washed with 5 ml of distilled water. Subsequently, the cartridge was eluted with 10 mL of 50% (v/v) methanol, and eluates were analyzed using HPLC with a mobile phase of 50%(v/v) methanol containing 0.1% (v/v) formic acid, a flow rate of 1.0 mL/min, injection volume of 10 μL, column temperature of 40 °C, and detection wavelength of 315 nm. A recovery test was performed 3 times using B-1, and G, MG, and DA were added at 4.0, 2.4, and 0.4 μmol/mL, respectively.
Calculation of mutation incidence
The effect on the mutation expression by the cytotoxicity of PA was examined using the Ames tests. Tests of mutagenicity were simultaneously performed in PA with (A) and without (B) added 0.3 μmol MG for each dose using TA100 in the absence of S9 mix. PA was prepared at doses of 20, 40, 60, and 80 μL, and 0.3 μmol MG was added to the same doses in parallel. As a control, 0.3 μmol MG (C) was used, and all sample solutions were adjusted to 100 μL. The recovery rate of revertants by added MG at each dose was calculated using the following equation and was defined as the mutation incidence of PA:
A regression line of mutation incidence of PAs was obtained using the values calculated in each dose from the above equation.
Calculation of mutagenic contribution ratios of DCs in PA
Mutagenic contribution ratios were expressed as percentages of mutagenicity from individual DCs relative to the total mutagenicity of PA and were calculated for the PA dose that showed twice the number of negative control revertants. The number of the net revertants induced by DC contents of PA (D) and the number of the net revertants of PA (Ec) were calculated using dose-response curves from Ames tests. Ec values were calculated by correcting the number of the net revertants of PA (Em) with mutation incidence rate (F), and Em values were the actual measured number of the net revertants of PA. Mutagenic contribution ratios were calculated under TA100 in the absence of S9 mix as follows:
Statistical analysis
Differences were identified using Student’s t-test and were considered significant when p < 0.05.
Results and discussion
Mutagenicity of PA
In the Ames tests of PA in TA100 and TA98 (Tables and ), 18 (9 WPA and 9 BPA) of 20 samples had positive mutagenicity. In TA100, 17 samples (8 WPA and 9 BPA) were positive in the absence of S9 mix, and 6 samples (2 WPA and 4 BPA) were positive in the presence of S9 mix. In TA98, 10 samples (4 WPA and 6 BPA) were positive in the absence of S9 mix, and only B-8 was positive in the presence of S9 mix. It was found that PA samples showed the strongest mutagenicity in TA100 in the absence of S9 mix because the values of specific mutagenicity in TA100 in the absence of S9 mix were the highest in the Ames test under various conditions. In particular, W-2 and B-10 were highly mutagenic in TA100 in the absence of S9 mix and led to the generations of more than 1000 revertants. In agreement with these observations, previous studies showed stronger mutagenicity of PA in TA100 than in TA98 but showed differing effects of metabolic activation.Citation10–12) In this study, the mutagenicity of PA was strongest in TA100 in the absence of S9 mix, although mutagenicity was reduced by metabolic activation in both TA100 and TA98. Similarly, Nakama et al. and Kim Oanh et al. reported that the mutagenicity of smoke generated by burning wood was strongest in TA100 in the absence of S9 mix.Citation11,14)
Table 1. Mutagenicity tests of pyroligneous acids in Salmonella typhimurium TA100.
Table 2. Mutagenicity tests of pyroligneous acids in Salmonella typhimurium TA98.
Determination of DCs in PA
DC contents of PA (Table ) were determined with a detection limit of 0.03 μmol/mL and a quantitation limit of 0.1 μmol/mL. Mean recovery rates ± coefficients of variation of G, MG, and DA were 98.5 ± 1.4, 90.1 ± 2.1, and 96.1 ± 1.8%, respectively. HPLC chromatograms of W-1 and B-1 were shown in Fig. . The highest DC contents were observed in sample B-1 and were present at a total of 0.83 mg/mL. DC contents and composition ratios of the 3 DCs, G, MG, and DA in PA were quite different between samples. Some among the 20 samples, e.g., a pair of W-1 and B-1, W-9 and B-9, and W-10 and B-10, are produced by the same maker. With regard to W-1 and B-1, although the composition ratios of DCs were different, the total DC content was the highest value in the group of WPAs or BPAs. With regard to W-10 and B-10, DC contents were lower values or less than the quantification limit, although these samples showed relatively strong mutagenicity and the equivalent specific mutagenicity values in TA100 in the absence of S9 mix. With regard to W-9 and B-9, DC contents were comparatively high, and the composition ratios of DCs were similar. It was suggested that DC contents and the composition ratios of DCs were similar in WPA and BPA of the same maker. Therefore, differing DC contents and the composition ratios of DCs in the present 20 PA samples may be caused by different production heating temperature and time for each maker. In agreement, previous studies showed that the composition ratios of DCs in coffee beans and cookies fluctuated with changes in heating times,Citation23,24) and MG production during heating varied with temperatures and heating times.Citation18) Therefore, differing contents and composition ratios of DCs in the present PA samples likely reflect differences in heating temperatures and times during manufacture. However, no significant differences in DC contents were observed between WPA and BPA samples by statistical analysis. DCs are the decomposition products of carbohydrate combustion and are the major components of common WPAs and BPAs. Previous reports also show no large differences in composition of most volatile and odor components between WPA and BPA,Citation5,25) suggesting similar compositions of these PAs.
Table 3. Contents of dicarbonyl compounds in pyroligneous acids.
Mutagenic contribution ratios of DCs from PA in TA100 in the absence of S9 mix
Most PA samples contained DCs and were mutagenic. Thus, in TA100 in the absence of S9 mix, the mutagenic contribution ratios of DCs were calculated as percentages of total PA mutagenicity. We calculated the mutagenic contribution ratios of DCs not only positive PA samples but also W-4, because W-4 was regarded as pseudo-positive, showing 1.5–2 times the number of negative control revertants. These analyses showed the mutagenic contribution ratios of MG in W-1, W-9, B-1, and B-9 exceed 100% (data are not shown), and these PA samples showed clear cytotoxicity in Ames tests (Table ). The presence of bactericidal components in PA has been previously reported,Citation1,5–7) and the cytotoxicity of these has been shown in S. typhimurium.Citation10–12) The present MG mutagenic contribution ratios exceeding 100% may reflect the underestimation of the mutagenicity of PA due to PA cytotoxicity. Therefore, to examine the effects on mutation expression by various components including toxicity to test bacteria, we performed the Ames test of PAs with and without added MG. Specifically, the recovery rate of the number of net revertants derived from MG that added to PA was calculated, and the recovery rates of MG were regarded as the mutation incidence. In a previous report, MG was identified as the most mutagenic of the 3 DCs in TA100 in the absence of S9 mix (about 18 times the mutagenicity of G).Citation26) Following similar observations (data are not shown), we used MG for recovery tests and confirmed that 0.3 μmol MG was optimum mutagenic dose but not cytotoxic. Under these conditions, the mutation incidence rates decreased with increasing PA doses, except in the case of the W-5 (Fig. ). The mutation incidence rates of W-1, W-4, W-9, B-1, B-6, and B-9 particularly decrease compared with those of other samples, and these PA samples showed marked growth inhibition (Fig. , Table ). Therefore, it was found that the mutation expression was decreased with increasing PA doses because components toxic to test bacteria increase with increasing PA doses. In addition, it was suggested that toxic components and amounts were different between samples because the mutation incidence showed a significantly different decreasing trend between samples. Therefore, to calculate the mutagenic contribution ratios, we determined to correct the number of the net revertants of PA by the mutation incidence rates with using a method for adjusting the decrease in the mutation expression level with the values of the mutation incidence rates. For the consistency of mutagenic contribution ratios of DCs, calculations were performed at doses that corresponded with 2-fold increases in the number of the revertants compared with the negative control, according to the threshold of positive PA mutagenicity. Mutagenic contribution ratios ranged 0.0–8.8% for G and 0.0–93.0% for MG, and they ranged 1.7–101.8% among all DCs (Table ). With regard to DA, mutagenic contribution ratio was not calculated because DA showed negative mutagenicity due to the cytotoxicity in this study. DCs contributed more than 25% of the mutagenicity of 12 PA samples (60% of all samples), and MG accounted for the highest mutagenic contribution ratio in all PA samples. In particular, W-1, W-9, B-1, and B-9 contained MG at concentrations of more than 2.4 μmol/mL, and MG contributed more than 70% of the mutagenicity. These data suggest that MG is the major mutagen in PA. However, despite the strong mutagenicity of W-2, W-10, and B-10, MG contents were low and showed mutagenic contribution ratios of less than 1.7%. Correlations between specific mutagenicity and MG contents among all 20 samples and after the exclusion of W-2, W-10, and B-10 showed coefficient (r) values of 0.17 and 0.90, respectively. Consequently, with the exception of W-2, W-10, and B-10, MG was a major mutagen in TA100 in the absence of S9 mix. On the other hand, the mutagenic activities of W-2, W-10, and B-10 indicate the presence of other mutagens, and these are currently under investigation.
Fig. 2. Mutation incidence rates in methylglyoxal recovery tests in Salmonella typhimurium TA100 in the absence of S9 mix.
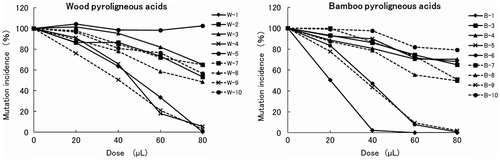
Table 4. Mutagenic contribution ratios of dicarbonyl compounds to the mutagenicity of pyroligneous acids in Salmonella typhimurium TA100 in the absence of S9 mix.
In TA100 in the presence of S9 mix, MG showed the strongest mutagenicity among 3DCs, and showed the mutagenicity in a dose-dependent manner at higher level than 0.75 μmol by dose-response curve (data are not shown). B-1 contains the highest concentration of MG between samples and showed the mutagenicity positive; however, the amount of MG was in low level to induce the mutation. Asita et al.Citation10) reported that in the TA100 or TA98 in the presence of S9 mix, the contribution of the polycyclic aromatic hydrocarbons (PAHs) to the mutagenicity of the wood smoke condensates could not be determined because of the concentrations of the PAHs was much lower level for inducing the mutation. These results suggested that many more mutagens contained in PA might contribute to the mutagenicity of PA in the presence of S9 mix.
In this study, we showed that MG is the major mutagen of WPAs and BPAs in TA100 in the absence of S9 mix. However, although PA has been used in various applications such as a bath agent and food additive whereby it is in direct contact with the human body, it appeared that PA has a serious safety issue because of the mutagenicity. Therefore, it is important to explore and evaluate the mutagenic substance in PA to ensure the safety of PA. The mutagens of PA are produced in the manufacturing process; so when the production conditions of PA are examined in a way the generation of MG is controlled or the formed MG is removed, PA might become safe. In this study, no significant differences in DCs contents were observed between WPA and BPA samples; however, it appears that there is great variability among quality of PA because of differences in the materials and production method based on the maker. At the present day, with respect to the components of PA, standard values of PAHs including benzo[a]pyrene have been established by JECFA (Joint FAO/WHO Expert Committee on Food Additives). However, the risk of these mutagenic substances has not been managed in Japan. Therefore, the setting of standard values of harmful substances and mutagenic substances such as MG in PA is desired in future. The present data will contribute to further studies of the risks associated with multiple harmful compounds that are present in consumables and the environment.
Author Contribution
A.O., M.A. and H.N. conceived and designed the experiments, the results, and wrote the manuscript. A.O. and M.A. performed the experiments and analyzed the data.
Disclosure statement
No potential conflict of interest was reported by the authors.
References
- Morey A, Bratcher CL, Singh M, et al. Effect of liquid smoke as an ingredient in frankfurters on Listeria monocytogenes and quality attributes. Poult. Sci. 2012;91:2341–2350.10.3382/ps.2012-02251
- Marumoto S, Yamamoto SP, Nishimura H, et al. Identification of a germicidal compound against picornavirus in bamboo pyroligneous acid. J. Agric. Food Chem. 2012;60:9106–9111.10.1021/jf3021317
- Ikegami F, Sekine T, Fujii Y. Anti-dermaptophyte activity of phenolic compounds in “Mokusaku-Eki”. J. Pharm. Soc. Japan. 1998;118:27–30.
- Takahara Y, Katoh K. Study on odor control using wood vinegars (II). Jpn. J. Public Health. 1994;41:147–156.
- Yasuhara A, Sugiura G. Volatile compounds in pyroligneous liquids from karamatsu and chishima-sasa. Agric. Biol. Chem. 1987;51:3049–3060.10.1271/bbb1961.51.3049
- Guillén MD, Manzanos MJ, Ibargoitia ML. Carbohydrate and nitrogenated compounds in liquid smoke flavorings. J. Agric. Food Chem. 2001;49:2395–2403.10.1021/jf000760t
- Fujimaki M, Kim K, Kurata T. Analysis and comparison of flavor constituents in aqueous smoke condensates from various woods. Agric. Biol. Chem. 1974;38:45–52.10.1271/bbb1961.38.45
- Jodai S, Yano S, Uehara T. Components of wood-vinegar liquors and their smoke flavors. J. Japan Wood Res. 1989;35:555–563.
- Ames BN, McCann J, Yamasaki E. Methods for detecting carcinogens and mutagens with the salmonella/mammalian-microsome mutagenicity test. Mutat. Res. 1975;31:347–363.10.1016/0165-1161(75)90046-1
- Asita AO, Matsui M, Nohmi T, et al. Mutagenicity of wood smoke condensates in the Salmonella/microsome assay. Mutat. Res. 1991;264:7–14.10.1016/0165-7992(91)90039-7
- Nakama A, Shimizu M, Fujita T, et al. Mutagenicity of smoke flavorings. J. Food Hyg. Soc. Japan. 1992;33:533–538_1.10.3358/shokueishi.33.533
- Putnam KP, Bombick DW, Avalos JT, et al. Comparison of the cytotoxic and mutagenic potential of liquid smoke food flavourings, cigarette smoke condensate and wood smoke condensate. Food Chem. Toxicol. 1999;37:1113–1118.10.1016/S0278-6915(99)00104-0
- Guillén MD, Sopelana D, Partearroyo MA. Determination of polycyclic aromatic hydrocarbons in commercial liquid smoke flavorings of different compositions by gas chromatography-mass spectrometry. J. Agric. Food Chem. 2000;48:126–131.10.1021/jf9908998
- Kim Oanh NT, Nghiem LH, Phyu YL. Emission of polycyclic aromatic hydrocarbons, toxicity, and mutagenicity from domestic cooking using sawdust briquettes, wood, and kerosene. Environ. Sci. Technol. 2002;36:833–839.10.1021/es011060n
- Kosugi A, Nagao M, Suwa Y, et al. Roasting coffee beans produces compounds that induce prophage λ in E. coli and are mutagenic in E. coli and S. typhimurium. Mutat. Res. 1983;116:179–184.10.1016/0165-1218(83)90058-7
- Nagao M, Takahashi Y, Yamanaka H, et al. Mutagens in coffee and tea. Mutation Res. 1979;68:101–106.10.1016/0165-1218(79)90137-X
- Kasai H, Kumeno K, Yamaizumi Z, et al. Mutagenicity of methylglyoxal in coffee. Gann. 1982;73:681–683.
- Homoki-Farkas P, Örsi F, Kroh LW. Methylglyoxal determination from different carbohydrates during heat processing. Food Chem. 1997;59:157–163.10.1016/S0308-8146(96)00273-7
- Usui T, Yanagisawa S, Ohguchi M, et al. Identification and determination of α-dicarbonyl compounds formed in the degradation of sugars. Biosci. Biotechnol. Biochem. 2007;71:2465–2472.10.1271/bbb.70229
- Bjeldanes LF, Chew H. Mutagenicity of 1,2-dicarbonyl compounds: maltol, kojic acid, diacetyl and related substances. Mutation Res. 1979;67:367–371.10.1016/0165-1218(79)90034-X
- Yahagi T, Degawa M, Seino Y, et al. Mutagenicity of carcinogenic azo dyes and their derivatives. Cancer Lett. 1975;1:91–96.10.1016/S0304-3835(75)95563-9
- Revel G, Pripis-Nicolau L, Barbe JC, et al. The detection of α-dicarbonyl compounds in wine by formation of quinoxaline derivatives. J. Sci. Food Agric. 2000;80:102–108.10.1002/(ISSN)1097-0010
- Daglia M, Papetti A, Aceti C, et al. Isolation and determination of α-dicarbonyl compounds by RP-HPLC-DAD in green and roasted coffee. J. Agric. Food Chem. 2007;55:8877–8882.10.1021/jf071917l
- Arribas-Lorenzo G, Morales FJ. Analysis, distribution, and dietary exposure of glyoxal and methylglyoxal in cookies and their relationship with other heat-induced contaminants. J. Agric. Food Chem. 2010;58:2966–2972.10.1021/jf902815p
- Akakabe Y, Tamura Y, Iwamoto S, et al. Volatile organic compounds with characteristic odor in bamboo vinegar. Biosci. Biotechnol. Biochem. 2006;70:2797–2799.10.1271/bbb.60317
- Dorado L, Montoya MR, Mellado JMR. A contribution to the study of the structure-mutagenicity relationship for α-dicarbonyl compounds using the Ames test. Mutation Res. 1992;269:301–306.10.1016/0027-5107(92)90212-K