Abstract
We analyzed DNA replication in early zebrafish embryos. The replicating DNA of whole embryos was labeled with the thymidine analog 5-ethynyl-2ʹ-deoxyuridine (EdU), and spatial regulation of replication sites was visualized in single embryo-derived cells. The results unveiled uncharacterized replication dynamics during zebrafish early embryogenesis.
Nuclear distribution of DNA replication sites in adult somatic cells of various species changes dynamically as S-phase progresses.Citation1–3) Cells in the early mouse embryo have an S-phase length similar to that of adult somatic cells (~8 h) and exhibit spatiotemporal regulation of replication patterns.Citation4,5) However, little is known about the spatiotemporal regulation of DNA replication in species where embryonic cells proceed through replication much faster than somatic cells. Through labeling of replicating DNA with thymidine analogs and subsequent fluorescence-mediated detection, sites of DNA replication can be visualized in the nucleus as granule-like structures called “replication foci.”Citation6–8) Recently, we reported five distinct distribution patterns of replication foci in the nucleus of AB9 cells derived from the caudal fin of adult zebrafish.Citation9) S-phase in zebrafish embryonic cells is thought to be much shorter than in somatic cells (~8-h-long S-phase in somatic cells),Citation10,11) which led us to investigate replication processes during zebrafish early embryogenesis to examine the relationship between S-phase length and spatial organization of replication sites.
Wild-type zebrafish were obtained from a commercial supplier (Meitosuien, Aichi, Japan). Fish were maintained at 28.0 ± 0.5 °C under light conditions of 14-h light and 10-h dark. To collect embryos, two female and three male zebrafish adults were mated. Spawning was induced by switching from dark to light. We used a water tank system (HASHIMOTO ELECTRONIC INDUSTRY CO., LTD, Mie, Japan) for automatically collecting zebrafish embryos.Citation12) Obtained embryos were cultured in breeding water maintained at 28.0 °C for 2–6 h prior to replication labeling.
We developed an experimental system for replication labeling of zebrafish embryos and visualization of labeled DNA in embryo-derived cells. To perform replication labeling, embryos were incubated in 1×Danieau’s buffer containing 10 mM EdU (Life Technologies, Carlsbad, CA, USA) for 1 min at 28.0 °C. After replication labeling, embryos were immediately immersed in ice-cold PBS to stop DNA replication. To quickly transfer embryos from an EdU-containing to an EdU-free environment, we used a fine mesh strainer as shown in Fig. (A). Egg membranes were removed with needles (26 G, Terumo, Tokyo, Japan), and the cells inside were collected using a micropipette. Collected cells were then mixed with SmearGell (Genostaff, Tokyo, Japan), and the resultant cell suspension was spread on 3-aminopropyltrimethoxysilane-coated coverslips. After 2 mins of air-drying, cells were fixed with 4% paraformaldehyde in PBS and then permeabilized with 0.5% Triton X-100 in PBS. Blocking before EdU detection was performed by incubation in 3% bovine serum albumin/0.1% Tween 20/Tris-buffered saline for 30 min at 37.0 °C. EdU-labeled DNA was detected using a Click-iT EdU imaging kit (Life Technologies) according to the manufacturer’s protocol. Recently, EdU has been frequently used as an alternative for BrdU to visualized sites of DNA synthesis.Citation13) Unlike other thymidine analogs, EdU incorporated into genomic DNA does not require a DNA denaturation step for detection. Immunohistochemical staining for proliferating cell nuclear antigen (PCNA) was performed as described previously.Citation14) In brief, cells were pre-treated with ice-cold cytoskeleton buffer (100 mM NaCl, 300 mM sucrose, 3 mM MgCl2, 10 mM Pipes, pH 6.8) containing 0.5% Triton X-100 for 30 s. Cells were incubated with anti-PCNA antibody (1:100; clone PC10, Mouse IgG2a; Biolegend, San Diego, USA) for 1 h at 37.0 °C. After washing with TBS, cells were incubated with Alexa Fluor 555-conjugated goat anti-mouse IgG (1:100; Life Technologies) for 30 min at 37.0 °C. Nuclear DNA was stained with 4ʹ6-Diamidino-2-phenylindole (DAPI). Samples were mounted with an anti-fade reagent containing DAPI (SlowFade® Gold antifade reagent with DAPI, Life Technologies) before imaging. DNA fibers from EdU-labeled cells were prepared as described previously.Citation9) A fluorescence microscope (Axioplan 2 MOT; Carl Zeiss, Inc. Jena, Germany) equipped with a 63×objective lens and an ORCA R2 camera (Hamamatsu; Shizuoka, Japan) was used for imaging. This experimental system allows us to collect single embryo-derived cells, thus enabling analysis of the dynamic nature of DNA replication within a single embryo.
Fig. 1. Visualization of replication dynamics in Zebrafish embryo.
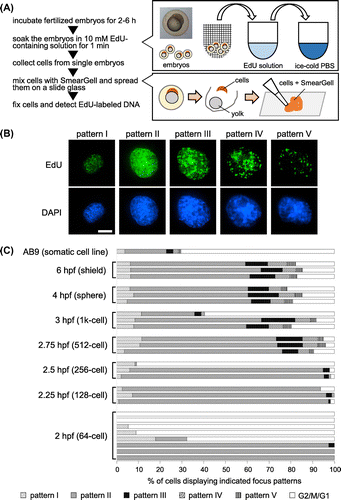
We first performed replication labeling of 6-h post-fertilization (hpf) embryos (early gastrula stage) where cell division takes between 78 and 151 min to analyze replication processes in single embryo-derived cells.Citation15) We identified five distinct distribution patterns of replication foci in the nucleus (Fig. (B) and Supplemental Fig. 1): pattern I, small foci distributed throughout the nucleoplasm; pattern II, increased number of foci in the nucleoplasm; pattern III, larger foci observed in both the nucleoplasm and nuclear periphery; pattern IV, relatively large foci localized exclusively at the nuclear periphery; and pattern V, a small number of replication foci. These foci colocalized with a replication protein PCNA which is known to accumulate at the site of newly synthesized DNA (Supplemental Fig. 2). Together with the data showing incorporation of EdU into DNA fiber molecules extended from the zebrafish embryo-derived cell nucleus (Supplemental Fig. 3), we concluded that observed EdU foci accurately reflect the sites of DNA synthesis in the cell nucleus.
Surprisingly, the distribution patterns of replication foci in 6 hpf embryo-derived cells were indistinguishable from those observed in somatic AB9 cells.Citation9) Furthermore, the frequency of each distribution pattern in S-phase was similar to those in somatic cells (Fig. (C)). Although the temporal order of these patterns was determined from our previous study,Citation9) the frequency of pattern II was particularly high, which was also observed in somatic cells. Similar results were obtained when we used embryos at 2.75–4 hpf (blastula stage) where cell division takes between 15 and 54 min.Citation15) These results strongly suggest that spatiotemporal regulation of replication sites in early developmental stages is equivalent to that observed in somatic cells. Thus, we conclude that spatiotemporal regulation of replication is organized regardless of the length of S-phase. It should be noted that the percentage of embryonic cells in S-phase (~90%) was greater than that of somatic cells (~30%), likely reflecting the extremely high rate of proliferation of embryonic cells (Fig. (C)).
Interestingly, we found striking differences in the frequency of each replication focus pattern and the percentage of S-phase cells between 2–2.5 and 2.75–6 hpf embryonic stages. For instance, some embryos at 2–2.5 hpf contain only pattern II nuclei, while others contain only non-labeled (G2/M/G1) nuclei. Observed differences are probably due to the synchronized cell division cycle of 2–2.5 hpf embryos rather than structural remodeling of chromatin that might occur during this period. The cell division cycle within zebrafish embryos is highly synchronous until the 2.5 hpf embryonic stage (256-cell stage) and loss of synchrony begins in the 2.75 hpf embryonic stage (512-cell stage) which corresponds to the midblastula transition.Citation10) During the highly synchronous division cycle, even slight differences in the timing of embryo collection and replication labeling between experiments could lead to inconsistent results and the overrepresentation of specific replication focus patterns within single embryos. As the frequency of pattern II nuclei is much higher than that of other patterns, it is not surprising that cells displaying pattern II were overrepresented in 2–2.5 hpf embryos.
Our study revealed replication dynamics during zebrafish early embryogenesis for the first time. We show the existence of spatiotemporal regulation of replication sites in embryo-derived cells undergoing fast cycles of replication, clearly demonstrating that a prolonged S-phase is not required for organizing replication focus patterns similar to those observed in somatic cells. This study also provides a basis for further experimentation using zebrafish embryos. Fish embryos can be used to assess various genotoxic stresses in aquatic environmentsCitation16, 17) and monitoring DNA replication processes is a sensitive method to quantify the effects of such stresses.Citation18, 19) Given the marked variation in the outcomes of replication labeling among individual 2–2.75 hpf embryos, timing of exposure to genotoxic stresses should be carefully considered to evaluate the effects on replication processes.
Author contributions
K.K., S.T., and K.O. designed the study. K.K., E.H., N.O., K.H., and S.O. performed the experiments and analyzed the results. E.A. and Y.T. maintained and provided zebrafish. M.O. discussed data and helped preparing the manuscript. K.K., S.T., and K.O. prepared the manuscript. All authors discussed the results and the manuscript.
Funding
This work was supported partly by the Grant-in-Aid for Scientific Research from the Ministry of Education, Science, Sports, and Culture of Japan, Kieikai Research Foundation, The Public Foundation of Elizabeth Arnold-Fuji, and Yamazaki Spice Promotion Foundation (to K. O.).
Supplemental material
The supplemental material for this paper is available at http://dx.doi.org/10.1080/09168451.2016.1141039
Disclosure statement
No potential conflict of interest was reported by the authors.
Supplementary_material.zip
Download Zip (924.8 KB)References
- Berezney R, Dubey DD, Huberman JA. Heterogeneity of eukaryotic replicons, replicon clusters, and replication foci. Chromosoma. 2000;108:471–484.10.1007/s004120050399
- Dimitrova DS, Berezney R. The spatio-temporal organization of DNA replication sites is identical in primary, immortalized and transformed mammalian cells. J. Cell Sci. 2002;115:4037–4051.10.1242/jcs.00087
- Takebayashi S, Sugimura K, Saito T, et al. Regulation of replication at the R/G chromosomal band boundary and pericentromeric heterochromatin of mammalian cells. Exp. Cell Res. 2005;304:162–174.10.1016/j.yexcr.2004.10.024
- Ferreira J, Carmo-Fonseca M. Genome replication in early mouse embryos follows a defined temporal and spatial order. J. Cell Sci. 1997;110:889–897.
- Bouniol-Baly C, Nguyen E, Besombes D, et al. Dynamic organization of DNA replication in one-cell mouse embryos: relationship to transcriptional activation. Exp. Cell Res. 1997;236:201–211.10.1006/excr.1997.3708
- Manders EM, Stap J, Brakenhoff GJ, et al. Dynamics of three-dimensional replication patterns during the S-phase, analysed by double labelling of DNA and confocal microscopy. J. Cell Sci. 1992;103:857–862.
- O’Keefe RT, Henderson SC, Spector DL. Dynamic organization of DNA replication in mammalian cell nuclei: spatially and temporally defined replication of chromosome-specific alpha-satellite DNA sequences. J. Cell Biol. 1992;116:1095–1110.10.1083/jcb.116.5.1095
- Ma H, Samarabandu J, Devdhar RS, et al. Spatial and temporal dynamics of DNA replication sites in mammalian cells. J. Cell Biol. 1998;143:1415–1425.10.1083/jcb.143.6.1415
- Kuriya K, Higashiyama E, Avşar-Ban E, et al. Direct visualization of DNA replication dynamics in zebrafish cells. Zebrafish. 2015;12:432–439.10.1089/zeb.2015.1151
- Kane DA, Kimmel CB. The zebrafish midblastula transition. Development. 1993;119:447–456.
- Kimmel CB, Ballard WW, Kimmel SR, et al. Stages of embryonic development of the zebrafish. Dev. Dyn. 1995;203:253–310.10.1002/aja.1002030302
- Avsar-Ban E, Miyake H, Obata M, et al. High-throughput injection system for zebrafish fertilized eggs. J. Data Mining Genomics Proteomics. 2013;4:1000137.
- Liboska R, Ligasová A, Strunin D, et al. Most anti-BrdU antibodies react with 2′-deoxy-5-ethynyluridine — the method for the effective suppression of this cross-reactivity. PLoS ONE. 2012;7:e51679.10.1371/journal.pone.0051679
- Takebayashi S, Tamura T, Matsuoka C, et al. Major and essential role for the DNA methylation mark in mouse embryogenesis and stable association of DNMT1 with newly replicated regions. Mol. Cell Biol. 2007;27:8243–8258.10.1128/MCB.00899-07
- Kane DA. Cell cycles and development in the embryonic zebrafish. Methods Cell Biol. 1999;59:11–26.
- Kosmehl T, Hallare AV, Reifferscheid G, et al. A novel contact assay for testing genotoxicity of chemicals and whole sediments in zebrafish embryos. Environ. Toxicol. Chem. 2006;25:2097–2106.10.1897/05-460R.1
- Yang F, Zhang Q, Guo H, et al. Evaluation of cytotoxicity, genotoxicity and teratogenicity of marine sediments from Qingdao coastal areas using in vitro fish cell assay, comet assay and zebrafish embryo test. Toxicol. In Vitro. 2010;24:2003–2011.10.1016/j.tiv.2010.07.019
- Sharma A, Kar A, Kaur M, et al. Specific replication factors are targeted by different genotoxic agents to inhibit replication. IUBMB Life. 2010;62:764–775.10.1002/iub.v62:10
- Zellweger R, Dalcher D, Mutreja K, et al. Rad51-mediated replication fork reversal is a global response to genotoxic treatments in human cells. J. Cell Biol. 2015;208:563–579.10.1083/jcb.201406099