Abstract
Postprandial blood glucose control is the major goal in the treatment of diabetes. Here, we investigated the effect of sea cucumber saponins (SCSs) on postprandial blood glucose levels. SCS inhibited yeast as well as rat intestinal α-glucosidase activity in a dose-dependent manner and showed better inhibition of yeast α-glucosidases compared to the positive control. Further studies were performed using ICR mice treated with SCS and starch or SCS alone by oral gavage. Unexpectedly, SCS increased postprandial blood glucose levels a short time (1 h) after oral gavage. The serum corticosterone (CORT) level showed a consistent correlation with glucose levels. In vitro experiments confirmed that SCS treatment increased the secretion of CORT in the Y1 adrenal cell line. Overall, these studies demonstrated that SCS gavage could inhibit α-glucosidase activity but cannot attenuate postprandial blood glucose level within short time periods. The underlying mechanisms are probably related to increased serum CORT levels.
The research idea of the effect of SCS on postprandial blood glucose.
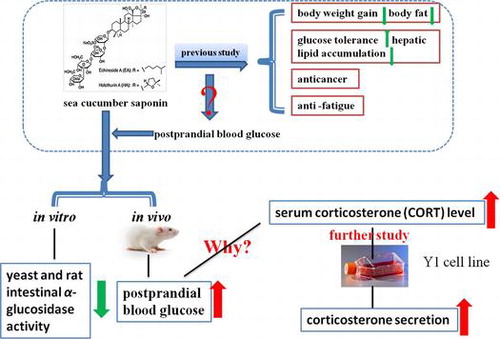
Diabetes, which is caused by compounding genetic and environmental factors, has become an alarming global problem in recent years.Citation1) Type 2 diabetes is characterized by a gradual decline in insulin secretion or insulin resistance in response to nutrient load and is primarily a disorder of postprandial hyperglycemia.Citation2) It is generally accepted that postprandial hyperglycemia is a dominant risk factor for cardiovascular disease and mortality in type 2 diabetes.Citation3) Hence, management of postprandial hyperglycemia is considered to be an important therapeutic strategy for type 2 diabetes.
Mammalian α-glucosidase which is located in the brush border surface membrane of intestinal cells is the key enzyme which catalyzes the final step of carbohydrate digestion. Inhibition of α-glucosidase can retard the liberation of D-glucose from dietary carbohydrates, delay glucose absorption, and therefore, suppress postprandial hyperglycemia.Citation4) Hence, one therapeutic approach for preventing diabetes is to delay glucose absorption via inhibition of α-glucosidase.Citation5) Three α-glucosidase inhibitors, acarbose (Glucobay), voglibose (Basen), and miglitol (Glyset), are widely used in the treatment of patients with type 2 diabetes.Citation6) The side effects of these α-glucosidase inhibitors have not been well established. Many natural products have also been investigated for their inhibitory effects on glucose absorption from the intestine. For instance, unsaturated fatty acids separated from sea cucumber were reported to be effective inhibitors of yeast as well as rat intestinal α-glucosidase.Citation4)
Sea cucumber is an economically important food resource in East Asia due to its high nutritional value.Citation7) Various extracts of sea cucumber have been reported to possess biological properties such as antioxidant, anticancer,Citation8,9) and antifatigue activities.Citation10) Sea cucumber saponins (SCS) are distributed in the body wall, internal organs, and glands.Citation11) Several studies have revealed bioactive properties of SCS, such as antitumor effects and alleviation of orotic acid-induced fatty liver in rats.Citation12) In addition, effects such as improving insulin resistance and obesity in mice fed with a high-fat diet have also been described.Citation13)
Corticosterone (CORT) is a 21-carbon steroid hormone of the corticosteroid type which is produced in the cortex of the adrenal glands in animals. In many species, CORT is a major glucocorticoid, involved in many important physiological activities.Citation14) Alterations in glucocorticoids such as CORT are known to alter energy metabolism and glucose homeostasis. Previous studies have revealed that enhanced activity of glucocorticoid hormones in humans contributes to insulin resistance.Citation15)
The present study aimed to investigate the inhibitory effect of SCS on yeast and mammalian α-glucosidase in vitro, and to further evaluate the influence of SCS on postprandial blood glucose levels in mice. The potential mechanisms were also explored, including serum CORT concentrations in vivo and CORT secretion in vitro.
Materials and methods
Preparation of SCS
Fresh sea cucumbers (Pearsonothuria graeffei) species were purchased from Nanshan aquatic market of Qingdao (Qingdao, China). SCS was prepared according to the method of HuCitation13). The final purification of holothurin A (HA) and echinoside A (EA) from SCS was conducted according to the method of DongCitation16). High-performance liquid chromatography (HPLC) analysis of SCS was conducted according to the method of WangCitation17). An Agilent 1100 HPLC system, equipped with a variable-wavelength detector at 205 nm, and a reverse-phase C-18 column (150 × 4.6 mm) (Agilent, Santa Clara, CA, USA) was used to determine the concentration of various triterpene glycosides. The mobile phase was a linear gradient starting at 30% acetonitrile in 0.1% aqueous trifluoroacetic acid increasing to 60% acetonitrile over 15 min. The flow rate was 1.0 mL/min. The retention times of all single triterpene glycosides were confirmed by comparison with standard (Sigma, St. Louis, MO, USA).
Extraction of rat intestinal α-glucosidases
Male Wistar rats were purchased from Qingdao medicine inspecting institute (Qingdao, China). Brush border membranes prepared from rat intestine according to the methods of Kessler et al.Citation18) were used as the source of rat intestinal α-glucosidase.
SCS α-glucosidase inhibitory activity kinetics
Yeast α-glucosidases and p-nitrophenyl-α-d-glucopyranoside (pNPG) were purchased from Sigma Chemical Co. (St. Louis, MO, USA). Yeast or rat α-glucosidase was premixed with SCS at various concentrations and 20 mM pNPG in phosphate buffer was added to the mixture to start the reaction. The reaction was incubated at 37 °C for 5 min and stopped by adding 1 mL of 0.1 M Na2CO3. α-Glucosidase activity was determined by measuring release of p-nitrophenol from pNPG at 405 nm.
The inhibitory effects of SCS on yeast α-glucosidase activity were examined with increasing concentrations of pNPG as a substrate, in the absence and presence of SCS at 0.05 mg/mL or 0.1 mg/mL. The enzyme reaction was performed by incubating the mixture at 37 °C for 30 min. The mode of inhibition was determined by Lineweaver–Burk plot analysis of the data, which were calculated from the result according to Michaelis–Menten kinetics.
Animals
The experiments were carried out according to the guidelines of the ethical committee of experimental animal care at Ocean University of China (Approval No. 2009-0007). Male ICR mice aged 4–5 weeks were provided by Vital River Laboratories (Beijing, China). Mice were maintained in pathogen-free conditions at constant humidity of 65 ± 15% and a temperature of 23 ± 2 °C with a 12 h light/dark cycle. Mice were adaptively fed for 1 week.
Blood glucose and serum CORT measurement
To test the effect of SCS on starch absorption, mice were randomly assigned to three groups of eight animals: control, SCS, and acarbose groups. Mice were deprived of food for 12 h but allowed free access to water before the test. Oral gavage was conducted at 8:00 am. All mice were fed 2 g/kg body weight soluble starch by gavage. The control group was treated by gavage with saline; the treatment groups were treated by gavage with either SCS (150 mg/kg body weight) or acarbose (10 mg/kg body weight).
For testing the effects of single oral gavage with SCS, mice were randomly assigned to two groups of eight. Mice were allowed free access to food and water. Mice in the control group were administered saline orally, and SCS treatment group mice were treated by gavage with SCS (150 mg/kg body weight) alone. The SCS gavage concentration was at the safe dose as tested before.
Blood samples were collected from the tail vein prior to oral gavage (baseline) and at selected times after oral gavage, and blood glucose concentration was determined using a blood glucose assay kit purchased from Nanjing Jiancheng Bioengineering Institute (Nanjing, China).
For serum CORT assessment, blood samples taken from the SCS single oral gavage test were centrifuged at 7500 rpm at 4 °C for 20 min and the serum was collected and stored at −80 °C until assayed. CORT was detected using a specific ELISA assay kit from Assaypro LLC (Saint Charles, MO, USA).
Cell culture and treatment
Y1 mouse adrenal tumor cells were purchased from Shanghai institute of biochemistry and cell biology (Shanghai, China), and grown in RPMI 1640 medium and fetal bovine serum (FBS) purchased from Gibco (Grand Island, NY) and 100 U/mL penicillin and 100 μg/mL streptomycin in a humidified atmosphere of 95% air and 5% CO2 at 37 °C.
Determination of CORT levels in cell culture supernatant
Y1 cells were plated at a density of 105 cells/mL in 12-well plates and cultured for 24 h. Cells were then incubated with 40 ng/mL EA or HA and at 0.5, 1, 4, 16, and 18 h after SCS treatment, 10 μL of cell culture supernatant was collected for CORT measurement by ELISA.
Statistical analysis
Data were expressed as mean ± SEM. Statistical significance between groups was determined by one-way ANOVA test using SPSS (version 18.0; SPSS Inc., Chicago, IL, USA), with p < 0.05 considered statistically significant.
Results
Components of SCS
HPLC analysis of total saponins from the sea cucumber P. graeffei showed that SCS contained 91.2 mg total triterpene glycosides/100 mg extract (wt/wt) (Fig. ), suggesting that triterpene glycosides are the main components of the extracts. Holothurin A (HA) and echinoside A (EA) were shown to be the major triterpene glycosides present, with others also present at low concentrations.
Fig. 1. HPLC analysis of total saponins extracted from P. graeffei.
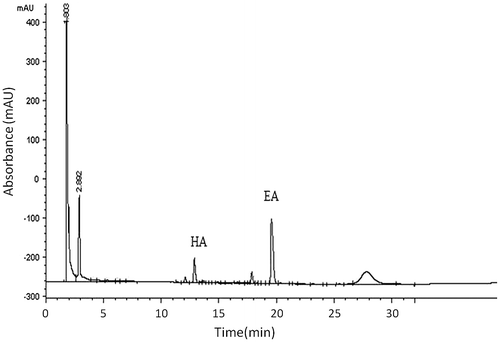
SCSs are primarily triterpene glycosides consisting of lanosterol-type aglycone with a saccharide moiety attached at the C-3 position. As shown in Fig. , both of these components have similar structures, and only differ in the terminal side chain in aglycone.Citation17)
Effects of SCS on α-glucosidase activity
Yeast α-glucosidases and rat intestinal α-glucosidase were used to study the inhibitory activity of SCS. As shown in Fig. (A) and (B), SCS significantly inhibited yeast α-glucosidases with an IC50 value of 0.04 mg/mL compared to positive control acarbose (IC50 = 1.06 mg/mL). The inhibitory activity of SCS against rat intestinal α-glucosidase was less pronounced than on yeast α-glucosidases, with an IC50 value of 0.086 mg/mL, while that of acarbose was 0.02 mg/mL, as shown in Fig. (C) and (D). These data show that yeast α-glucosidase activity was significantly reduced by SCS compared with acarbose in a dose-dependent manner, while the inhibitory effect of SCS on rat intestinal α-glucosidase was less than that of acarbose.
Kinetics of enzyme inhibition
The inhibition mode of SCS against yeast α-glucosidases was analyzed by performing enzyme assays at different concentrations of pNPG and SCS. Data are presented as a Lineweaver–Burk (double-reciprocal) plot (Fig. ). SCS showed a non-competitive type of inhibition against α-glucosidases, as proved by the decrease in Vmax value with increasing SCS concentration, while the Km value remained constant. The Ki value of SCS was 0.04 mg/mL.
Effects of SCS on blood glucose levels
Since SCS was shown to inhibit rat intestinal α-glucosidase, the effect of SCS on blood glucose level was examined after starch loading in vivo. As shown in Fig. (A), blood glucose concentration in control mice increased to 8.4 mmol/L at 1 h, and decreased to baseline levels at 2 h, while the acarbose group showed a lower glucose concentration from 0.5 to 2 h compared with controls. However, mice in the SCS group showed a higher glucose level from 0.5 to 2 h compared with controls, as illustrated by the increase in glucose level from 4.8 to 10.5 mmol/L 2 h after starch administration.
Fig. 5. Effect of SCS administration on blood glucose level.
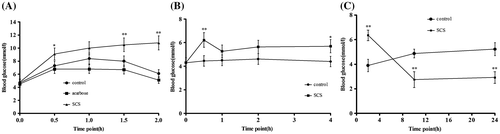
In order to explore the effect of SCS alone on blood glucose, the effect of SCS gavage was examined without starch loading. It was observed that postprandial blood glucose concentration increased in both groups within 0.5 h (Fig. (B)), while mice treated with SCS exhibited higher blood glucose concentrations compared with controls at 0.5 h (p < 0.01). At the 1 and 2 h time points, SCS group mice still showed higher glucose levels than controls, but this was not statistically significant. Further studies were then performed over a longer time course. Consistent with the results from the short time points, the glucose level of the SCS group was significantly higher at 2 h compared with controls, with a concentration of 6.35 mmol/L (p < 0.01). At the 10 and 24 h time points, the SCS group blood glucose decreased to baseline levels and was significantly lower than controls (p < 0.01), as shown in Fig. (C).
Effects of SCS on serum CORT concentration
It is well known that CORT alters energy metabolism and glucose homeostasis. To investigate this, serum CORT levels were determined after SCS administration. As shown in Fig. (A), serum CORT levels were induced by SCS compared with control mice from 0 to 4 h and reached a peak at 0.5 h in both groups, with a concentration of 233.95 and 411.37 ng/mL in control and SCS groups, respectively (p < 0.01). SCS group mice showed a significantly higher concentration level than controls from 1 to 4 h (p < 0.01).
Fig. 6. Effects of SCS administration on serum CORT level.
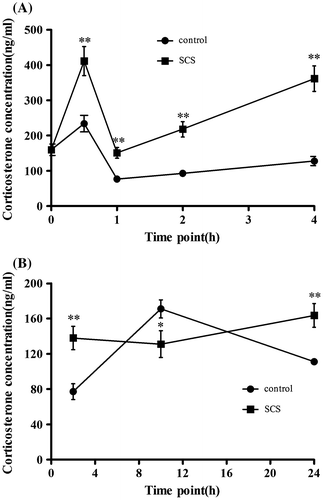
Long-term experiments were conducted simultaneously. SCS treatment showed enhanced serum CORT levels at 2 h compared with controls (p < 0.01). Subsequently, mice treated with SCS exhibited lower CORT levels compared with controls at 10 h (p < 0.05). In contrast, the results at 24 h showed higher serum CORT levels in the SCS group than controls (p < 0.01).
Effects of SCS on CORT secretion in Y1 cells
Based on in vivo experiments, it could be possible that SCS may directly affect CORT secretion. Therefore, CORT production was measured using Y1 mouse adrenal tumor cells after SCS treatment in vitro. Given that both EA and HA showed no toxic effects on the growth of Y1 cells below 40 ng/mL within 24 h (data not shown), this concentration was therefore selected for further experiment. Treatment of Y1 cells with EA and HA over 18 h induced the accumulation of CORT in cell culture supernatants in a time-dependent manner compared with controls (p < 0.05). Compared with HA treatment, exposure of Y1 cells to EA caused significantly greater CORT secretion over the time course (p < 0.05, Fig. ).
Discussion
Type 2 diabetes is the most common form of diabetes and is usually characterized by postprandial hyperglycemia. In recent years, many studies have reported that postprandial hyperglycemia is the main factor causing diabetes complications such as retinopathy, nephropathy, and cardiovascular disease.Citation19) Thus, the control of postprandial hyperglycemia is one of the major goals for prevention and treatment of diabetes.
The control of postprandial blood glucose levels has been studied in the context of several types of food. For instance, saponins from Solanum anguivi fruit showed hypoglycemic effects in alloxan-induced diabetic rats.Citation20) Plant saponins including daidzein were reported to be effective in reducing postprandial blood glucose in normal and type 2 diabetic rats by suppressing intestinal α-glucosidase.Citation21) Similar to the structure of plant saponins, SCSs are primarily triterpene glycosides of lanosterol-type aglycone with a saccharide moiety attached at the C-3 position. However, the effect of these saponins on postprandial hyperglycemia blood glucose has not been well established.
In the present study, the effect of SCS on α-glucosidase inhibition in vitro was investigated. SCS was shown to be effective inhibitor of α-glucosidase, exhibiting effective inhibitory activity against both yeast and rat intestinal α-glucosidase, suggesting that SCS is likely to be effective in reducing postprandial blood glucose levels. The effect of SCS on rat intestinal α-glucosidase inhibition was less than that of acarbose. The structures of various sources α-glucosidase are different, which affects substrate specificity and the binding of inhibitors. It has been previously reported that acarbose has high inhibitory effects on mammalian α-glucosidase, which is probably due to the substrate specificities that depend on the α-glucosidase source,Citation22) suggesting that the binding of SCS is less specific to the enzyme than acarbose. Our previous study found that SCS treatment improved oral glucose tolerance in high-fat diet-fed mice.Citation13) The inhibitory effect of SCS on α-glucosidase is likely to contribute to this effect. Furthermore, a non-competitive inhibition mode was identified from the study of inhibitory effects of SCS on yeast α-glucosidase, the Ki value was 0.04 mg/mL. Several plant-derived saponins have been proved to be beneficial inhibitor of yeast α-glucosidase and showed better effect than acarbose,Citation21,23) it was identified that soy saponins were non-competitive inhibitors of α-glucosidase.Citation24) The similar inhibitory effect between plant saponins and SCS was probably due to their triterpene glycosides structures.
Given that SCS was effective in suppressing α-glucosidase activity, the effect of SCS on postprandial blood glucose level by oral gavage was further examined, with acarbose as a positive control. Consistent with the in vitro study, acarbose inhibited the increase in postprandial blood glucose level after gavage with starch. However, the SCS-treated mice showed a higher postprandial blood glucose concentration than controls, which further illustrated that the increased blood glucose level is probably due to SCS treatment, especially over a short time course.
Glucocorticoid hormones are produced in the adrenal cortex under the control of the hypothalamic–pituitary–adrenal axisCitation25) and play an important role in regulating glucose homeostasis. It has been proposed that ginseng saponin acts primarily on the hypothalamus or hypophysis, and stimulates corticotrophin (ACTH) secretion resulting in increased CORT secretion in the adrenal cortex.Citation26) The present study assessed serum CORT concentrations to examine whether the increased glucose levels over a short time after SCS treatment were connected with serum CORT. In line with variations in blood glucose variation, results of in vivo studies showed a rise in the level of serum CORT induced by SCS over short time points, which confirmed that the increased blood glucose level may be attributed to the effect of SCS on serum CORT levels.
Previous studies found that glucocorticoids appeared to reduce insulin receptor substrate 1 protein content and therefore suppressed intracellular insulin signaling.Citation27) Others have also proposed that glucocorticoids increase blood glucose by mobilizing substrates for hepatic gluconeogenesis, and increase gluconeogenic enzyme expression, enhancing gluconeogenesis in the liver.Citation28) In addition, peripheral glucose uptake and utilization can be inhibited by glucocorticoids, partly due to the decreased translocation of glucose transporters (e.g. GLUT4) to the cell surface.Citation29,30) Moreover, it has been suggested that glucocorticoids could inhibit glucose-stimulated insulin secretion from pancreatic β-cells.Citation31) In consequence, although intestinal α-glucosidase was inhibited by SCS, excess CORT secreted by the adrenal cortex subsequently influenced glycometabolism and led to the increased blood glucose observed thereafter.
In line with the experiments at early time points, serum CORT concentration was increased by SCS treatment 2 h after oral gavage. It can therefore be speculated that the variation of CORT in both groups at 10 h (6:00 pm) and 24 h (8:00 am) was connected with circadian rhythms. Our previous study demonstrated that control and SCS groups exhibited daily CORT peak phase at ZT20 (light/night transition).Citation32) Compared with controls, the previous study found that the SCS group showed a higher CORT level at 8:00 am and a lower level at 8:00 pm, which is consistent with our present data.
Results of in vitro studies provided evidence that SCS directly induced CORT secretion in Y1 cells within 18 h, as indicated by the observation that CORT secretion was significantly increased at 0.5 h after HA and EA treatment, and later showed a mild increase, suggesting that the effect of SCS on CORT secretion within a short time was more pronounced. A much more obvious effect of EA than HA was observed from 1 to 18 h after SCS treatment. This difference is likely due to the differences in the structures of these compounds. It has been proved in our previous study that SCS appeared rapidly in serum after oral gavage and the concentration ranged from 0.24 to 0.83 μg/mL within 24 h.Citation33)
In summary, the biological effect of SCS on postprandial glucose levels was evaluated in our present study. Powerful evidence was obtained to support the idea that SCS is effective in inhibiting α-glucosidase. However, the postprandial glucose level within a short time after SCS oral gavage increased dramatically, and the underlying mechanisms were probably due to the promotion of CORT secretion in a direct or indirect way, it was indicated in vitro that CORT was directly induced by SCS and it needs to be further researched whether SCS affect CORT in an indirect way. The beneficial effect that α-glucosidase activity was inhibited by SCS cannot offset the adverse effect of the rising CORT, which led to the rise in postprandial blood glucose level. Traditional Chinese medicine believes that sea cucumber has an antifatigue effect, which may contribute to increased CORT secretion. Long-term SCS treatment has been shown to suppress body weight gain, decrease body fat, improve glucose tolerance, improve hyperlipidemia, and suppress hepatic lipid accumulation by preventing adipokine imbalance in high-fat diet-fed mice in previous work,Citation13) suggesting the distinction between short-term and long-term effects of SCS treatment. The specific underlying mechanisms by which SCS affects serum CORT concentration require further studies.
Authors contributions
Changhu Xue and Yuming Wang designed the study and interpreted the results. Xueyuan Fu did the experiment and wrote this paper, Min Wen did the animal experiment. Xiuqing Han collected data and drafted the manuscript, Yong Xue and Jingfeng Wang modified this paper. Teruyoshi Yanagita played an important role in interpreting the results.
Disclosure statement
No potential conflict of interest was reported by the authors.
Additional information
Funding
References
- Lan JR, Zhao YY, Dong FX, et al. Meta-analysis of the effect and safety of berberine in the treatment of type 2 diabetes mellitus, hyperlipemia and hypertension. J. Ethnopharmacol. 2015;161:69–81.10.1016/j.jep.2014.09.049
- Leiter LA, Ceriello A, Davidson JA, et al. Postprandial glucose regulation: new data and new implications. Clin. Ther. 2005;27:S42–S56.10.1016/j.clinthera.2005.11.020
- Miyamoto T, Fukuda K, Kimura T, et al. Effect of percutaneous electrical muscle stimulation on postprandial hyperglycemia in type 2 diabetes. Diabetes Res. Clin. Pract. 2012;96:306–312.10.1016/j.diabres.2012.01.006
- Nguyen TH, Um BH, Kim SM. Two unsaturated fatty acids with potent α-glucosidase inhibitory activity purified from the body wall of sea cucumber (Stichopus japonicus). J. Food Sci. 2011;76:H208–H214.10.1111/jfds.2011.76.issue-9
- Kim YM, Jeong YK, Wang MH, et al. Inhibitory effect of pine extract on α-glucosidase activity and postprandial hyperglycemia. Nutrition. 2005;21:756–761.10.1016/j.nut.2004.10.014
- Kamiyama O, Sanae F, Ikeda K, et al. In vitro inhibition of α-glucosidases and glycogen phosphorylase by catechin gallates in green tea. Food Chem. 2010;122:1061–1066.10.1016/j.foodchem.2010.03.075
- Wu FJ, Xue Y, Tang QJ, et al. The protective effects of cerebrosides from sea cucumber and starfish on the oxidative damage in PC12 cells. J. Oleo Sci. 2013;62:717–727.10.5650/jos.62.717
- Wijesinghe WA, Jeon YJ, Ramasamy P, et al. Anticancer activity and mediation of apoptosis in human HL-60 leukaemia cells by edible sea cucumber (Holothuria edulis) extract. Food Chem. 2013;139:326–331.10.1016/j.foodchem.2013.01.058
- Tian F, Zhang XW, Tong YG, et al. PE, a new sulfated saponin from sea cucumber, exhibits anti-angiogenic and anti-tumor activities in vitro and in vivo. Cancer Biol. Ther. 2005;4:874–882.10.4161/cbt.4.8.1917
- Li B, Wang JF, Fu J, et al. Anti-fatigue effect of sea cucumber Stichopus japonicus in mice. Food Sci. 2010;31:244–247. Chinese.
- Van Dyck S, Gerbaux P, Flammang P. Elucidation of molecular diversity and body distribution of saponins in the sea cucumber Holothuria forskali (Echinodermata) by mass spectrometry. Comp. Biochem. Physiol. B. 2009;152:124–134.10.1016/j.cbpb.2008.10.011
- Hu XQ, Wang YM, Wang JF, et al. Dietary saponins of sea cucumber alleviate orotic acid-induced fatty liver in rats via PPARalpha and SREBP-1c signaling. Lipids Health Dis. 2010;9:1–9.
- Hu XQ, Li ZJ, Xue Y, et al. Dietary saponins of sea cucumber ameliorate obesity, hepatic steatosis, and glucose intolerance in high-fat diet-fed mice. J. Med. Food. 2010;15:909–916.
- Andrews RC, Walker BR. Glucocorticoids and insulin resistance: old hormones, new targets. Clin. Sci. 1999;96:513–523.10.1042/cs0960513
- Reynolds RM, Walker BR. Human insulin resistance: the role of glucocorticoids. Diabetes Obes. Metab. 2003;5:5–12.10.1046/j.1463-1326.2003.00221.x
- Dong P, Xue CH, Yu LF, et al. Determination of triterpene glycosides in sea cucumber (Stichopus japonicus) and its related products by high-performance liquid chromatography. J. Agric. Food Chem. 2008;56:4937–4942.10.1021/jf800893r
- Wang YM, Wang JH, Yanagita RC, et al. Effects of two sulfated triterpene saponins echinoside A and holothurin A on the inhibition of dietary fat absorption and obesity reduction. Biosci. Biotechnol. Biochem. 2014;78:139–146.10.1080/09168451.2014.877830
- Kessler M, Acuto O, Storelli C, et al. A modified procedure for the rapid preparation of efficiently transporting vesicles from small intestinal brush border membranes. Their use in investigating some properties of D-glucose and choline transport systems. BBA-Biomembranes. 1978;506:136–154.
- Davis SN. Postprandial physiology and the pathogenesis of type 2 diabetes mellitus. Insulin. 2008;3:132–140.10.1016/S1557-0843(08)80029-2
- Elekofehinti OO, Kamdem JP, Kade IJ, et al. Hypoglycemic, antiperoxidative and antihyperlipidemic effects of saponins from Solanum anguivi Lam. fruits in alloxan-induced diabetic rats. S. Afr. J. Bot. 2013;88:56–61.10.1016/j.sajb.2013.04.010
- Park MH, Ju JW. Daidzein inhibits carbohydrate digestive enzymes in vitro and alleviates postprandial hyperglycemia in diabetic mice. Eur. J. Pharmacol. 2013;712:48–52.10.1016/j.ejphar.2013.04.047
- Calder PC, Geddes R. Acarbose is a competitive inhibitor of mammalian lysosomal acid α-d-glucosidases. Carbohydr. Res. 1989;191:71–78.10.1016/0008-6215(89)85047-5
- Lee JS, Choi MS, Seo KI, et al. Platycodi radix saponin inhibits α-glucosidase in vitro and modulates hepatic glucose-regulating enzyme activities in C57BL/KsJ-db/db mice. Arch. Pharm. Res. 2014;37:773–782.10.1007/s12272-013-0259-3
- Quan J, Yin X, Jin M, et al. Study on the inhibition of alpha-glucosidase by soyasaponins. J. Chin. Med. Mater. 2003;26:654–656.
- Feng B, He Q, Xu HY. FOXO1-dependent up-regulation of MAP kinase phosphatase 3 (MKP-3) mediates glucocorticoid-induced hepatic lipid accumulation in mice. Mol. Cell. Endocrinol. 2014;393:46–55.10.1016/j.mce.2014.06.001
- Hiai S, Yokoyama H, Oura H, et al. Stimulation of pituitary-adrenocortical system by ginseng saponin. Endocrinol. Jpn. 1979;26:661–665.10.1507/endocrj1954.26.661
- Motta K, Barbosa AM, Bobinski F, et al. JNK and IKKβ phosphorylation is reduced by glucocorticoids in adipose tissue from insulin-resistant rats. J. Sreroid. Biochem. 2015;145:1–12.
- Hanson RW, Reshef L. Regulation of phosphoenolpyruvate carboxykinase (GTP) gene expression. Annu. Rev. Biochem. 1997;66:581–611.10.1146/annurev.biochem.66.1.581
- Dimitriadis G, Leighton B, Parry-Billings M, et al. Effects of glucocorticoid excess on the sensitivity of glucose transport and metabolism to insulin in rat skeletal muscle. Biochem. J. 1997;321:707–712.10.1042/bj3210707
- Virgin CE, Ha TPT, Packan DR, et al. Glucocorticoids inhibit glucose transport and glutamate uptake in hippocampal astrocytes: implications for glucocorticoid neurotoxicity. J. Neurochem. 1991;57:1422–1428.10.1111/jnc.1991.57.issue-4
- Delaunay F, Khan A, Cintra A, et al. Pancreatic beta cells are important targets for the diabetogenic effects of glucocorticoids. J. Clin. Invest. 1997;100:2094–2098.10.1172/JCI119743
- Wen M, Cui J, Xu J, et al. Effects of dietary sea cucumber saponin on the gene expression rhythm involved in circadian clock and lipid metabolism in mice during nighttime-feeding. J. Physiol. Biochem. 2014;70:801–808.10.1007/s13105-014-0349-9
- Song SS, Zhang LY, Liu XF, et al. The preliminary study on the digestion and obsortion characteristics of sea cucumber saponins Echinoside A in rats. Chin. J. Mar. Drugs. 2015;34:41–46. Chinese.