Abstract
In tritrophic interactions, plants recognize herbivore-produced elicitors and release a blend of volatile compounds (VOCs), which work as chemical cues for parasitoids or predators to locate their hosts. From detection of elicitors to VOC emissions, plants utilize sophisticated systems that resemble the plant–microbe interaction system. Fatty acid–amino acid conjugates (FACs), a class of insect elicitors, resemble compounds synthesized by microbes in nature. Recent evidence suggests that the recognition of insect elicitors by an ancestral microbe-associated defense system may be the origin of tritrophic interactions mediated by FACs. Here we discuss our findings in light of how plants have customized this defense to be effective against insect herbivores, and how some insects have successfully adapted to these defenses.
Graphical abstract
Fatty acid–amino acid conjugates (FACs) in caterpillar regurgitant triggers herbivore-specific plant defenses.
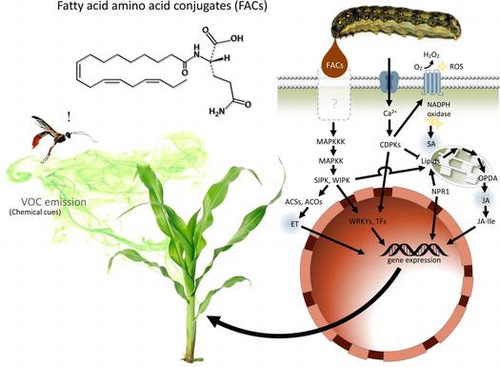
Living organisms, including plants, have developed various sensors to gain vital information for intra- and inter-specific communication and prey–predator interactions. Insects and plants employ surprisingly sophisticated chemical communication systems, mediated by molecules such as pheromones, kairomones, and hormones.Citation1,2) However, the more interactions mediated by compounds the more corresponding receptors are required, highlighting the crucial difference between chemosensory (olfaction and contact chemoreception) and other perceptions, such as visual and auditory. In tritrophic interactions, plants recognize herbivore feeding and release a blend of volatile organic compounds (VOCs) that facilitate parasitoids or predators to locate their host species. This is the so-called “indirect defense” of plants, using the natural enemies of herbivores. Although antennal sensitivity and learning ability of the natural enemies are essential, the plants’ ability to recognize herbivore elicitors and discriminate such signals from mechanical damage or fungal infections is of utmost importance. This review summarizes recent advances in research on tritrophic interactions mediated by herbivore-produced fatty acid–amino acid conjugates (FACs) and discusses the questions (1) what is the basic mechanism from which tritrophic interactions originated, and (2) how has it gained its present-day function.
I. Tritrophic interaction overview
Intensive work over the past decades have revealed that tritrophic interactions are quite common across a broad range of plant species; at least 49 plants originating from 25 families have been reported to be involved in such interactions.Citation3) However, the mechanism of VOC emission differs by plant. Cabbage plants infested by (white) cabbage butterfly, Pieris brassicae, larvae release a larger amount of a blend of VOCs than artificially damaged plants, and the elicitor was identified to be β-glucosidase in the larval saliva.Citation4) A similar model was reported for spider mite-infested lima beans that emit a blend of homoterpenes, which can be mimicked by β-glucosidase treatment of damaged leaves.Citation5,6) In both the cases, β-glucosidase cleaves off the VOCs from constitutive phytochemicals stored as non-volatile glucosides in the leaf tissues. Furthermore, there are plants which release in response to herbivory elicitors de novo-synthesized VOCs.Citation7) These de novo-synthesized VOCs primarily comprise terpenoids, fatty acid derivatives, phenylpropanoids, and nitrogen- and sulfur-containing compounds. The most well-known elicitors are FACs, in the oral secretions of lepidopteran caterpillars (Fig. ).Citation8–11) Detection of the FACs in plants triggers sequential signal transduction resulting in systemic VOC synthesis as well as induction of direct defense substances.Citation12–15) Present knowledge of this complex signal transduction ultimately leading to gene expression has been recently reviewed and summarized.Citation16,17) Furthermore, terpenoids are synthesized in a multistep pathway, and this diurnally-regulated biosynthesis follows the light-dependent opening of the stomata, which are essential for VOC emission.Citation18) If this emission is a “cry for help”, the plant would simply release the volatile signals; indeed, VOC emission due to β-glucosidase is simple and functions well.Citation4)
Fig. 1. Structure of herbivore-produced elicitors of plant VOC emissions.
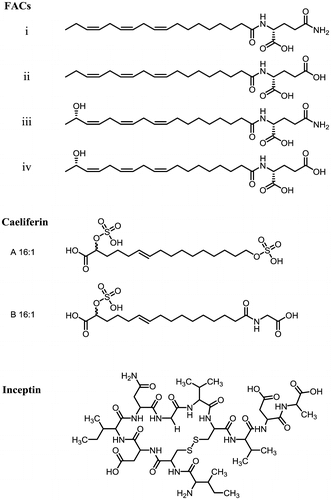
Redefining plant VOCs as intermediates interacting with a broader range of organisms may explain why VOC induction is so complicated. The plant–microbe interactions mediated by VOCs were recently reviewed.Citation19) The surfaces of flowers, leaves, and roots are known habitats of numerous microorganisms that affect plant fitness, either positively or negatively, and interfere with plant-insect interactions. VOCs such as terpenoids, benzenoid compounds, aliphatics, and sulfur-containing compounds emitted by plants strongly affect these colonizing bacteria. In particular, terpenoids have been shown to perform defensive roles in numerous plant species, and their antimicrobial properties have been reported in Zea mays and Arabidopsis.Citation20–22) Not only do they prevent the establishment of detrimental microbes, they can also promote the growth of mutualists by serving as carbon sources.Citation19) Considering that such interaction originated from ancient immune responses in primitive plants and bacteria, and that arthropod herbivory joined the interaction in later periods, it would not be surprising if plants use the same or a similar pathway for elicitor perception, signaling, activation, or suppression in their defense against insects. Interestingly, there are striking similarities in signaling pathways between plant-pathogen and plant-herbivore interactions (Fig. ).Citation16,23–25) Briefly, herbivore infestation induces the generation of reactive oxygen species (ROS) and calcium influx, which sequentially activates calcium-dependent protein kinases and MAPK cascades (including salicylic acid-induced protein kinase, SIPK, and wound-induced protein kinase, WIPK) at the damaged site. These are early events similarly triggered by microbe infections.Citation26–28) Also, phytohormone synthesis and activation of transcription regulators overlap with the early changes caused by microbe-associated molecular patterns.Citation29,30)
Fig. 2. Models of signal transduction and cellular events in plant defense responses triggered by microbes and herbivorous insects.
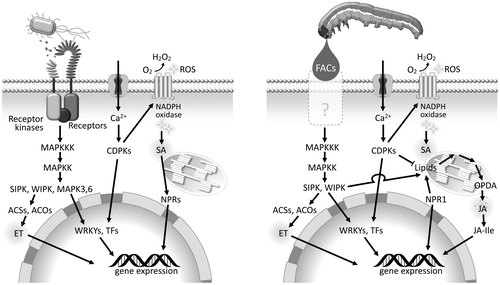
In contrast to the recent progress in understanding the signal cascade, little is known about the first step: the elicitor recognition mechanism in plants. An early study reported that volicitin, a FAC, binds to maize plasma membranes with properties resembling that of a ligand–receptor interaction.Citation31) However, no further data on this interaction has been reported. Another possibility is that FACs directly affect the cell membrane potentials by releasing Ca2+ and trigger signal transduction. Studies in transgenic aequorin expressing soybean cells exposed to FACs demonstrated a concentration-dependent influx of Ca2+, which was comparable with the effects of SDS detergent.Citation32) However, contrary to FAC induction of intact plant, it is worth noting that in that system, the amino acid moiety of FACs (glutamine/glutamate) and its stereochemistry did not affect the influx activity.Citation32) Oral secretions of various lepidopteran species were shown to exhibit ion channel-forming activity on artificial membranes; FACs also interfered with the membrane, but did not form stable channels.Citation33) Whether the first step of FACs elicitation is caused by a ligand–receptor interaction or direct interference with the function of the cell membrane remains to be determined.
Two more classes of herbivore-associated VOC elicitors have been described: caeliferins and inceptins (Fig. ). Caeliferins were identified from the oral secretions of the herbivorous grasshopper Schistocerca americana and its related species in the suborder Caelifera.Citation40) Interestingly, caeliferins share a similar structural property with FACs, with both hydrophobic (long chain hydrocarbon moiety) and hydrophilic (sulfated hydroxyls and glycine) moieties. Its elicitor activity has been demonstrated in Z. maysCitation40) and Arabidopsis thaliana,Citation29), but there is no available data on its reception mechanism. On the other hand, inceptins in the oral secretions of the fall armyworm Spodoptera frugiperda are disulfide-bridged proteolytic fragments of the chloroplastic ATP synthase γ-subunit (Fig. ).Citation41) This peptide is released from plants that larvae have fed on, and is similar to systemin, a damage-associated plant hormone identified in solanaceous plants. However, systemin can be released by wounding alone, while inceptin release is dependent on herbivores. Furthermore, the modification (cleavage site) for inceptin generation differs by insect species, suggesting that the elicitor may convey more specific information of the attacker to the plants. In this regard, inceptin is more similar to flg22, a pathogen-associated peptide elicitor.Citation42)
II. FAC analogs and their function in insects
Several FAC analogs have been identified from lepidopteran insects.Citation8,43–48) These are classified into four types, represented by the compounds shown in Fig. : (i) glutamine conjugates, which are putative evolutionary ancestors of FACs; (ii) glutamate type, which are less common but are the major FAC components in some species; (iii) hydroxylated fatty acids conjugated with glutamine; and (iv) hybrids of (ii) and (iii). Since the fatty acid moiety is directly obtained from the diet, linolenic acid can be replaced with linoleic acid, oleic acid, or any other saturated/unsaturated fatty acid of the respective host plants, and the composition ratio depends on the ratio of the diet.Citation10,49) The amino acid moiety on the other hand, appears to be obtained from pools within the insect herbivoresCitation10) and the glutamine/glutamate ratio is very likely species-specific.Citation50)
As is expected by the chemical structure, the initially predicted function of FACs was as a biosurfactant in the caterpillar gut tract,Citation48,51) and indeed, the critical micellar concentration of N-linolenoyl-L-glutamine (i) was reported to be low enough for this function.Citation52) However, this cannot explain why only glutamine or glutamic acid is used for FACs synthesis, while other amino acids are just as capable of serving as the hydrophilic domain of the surfactant. Our recent study demonstrated that in Spodoptera litura larvae FACs were synthesized glutamine-dominantly.Citation53,54) Here FACs function most likely as a sink for glutamine by depleting glutamine in midgut cells, changing the equilibrium and favoring glutamine synthesis. Consequently FACs may serve as a primary storage of glutamine synthesized by glutamine synthetase (Fig. ).Citation55) Although this hypothesis has not been clearly addressed, it offers a partial explanation as to why only glutamine/glutamate are used for FAC synthesis. The FAC conjugase embedded in the membrane of the crop and midgut tissues of Manduca sexta is sufficiently fast to catalyze the FAC synthesis observed in insects.Citation56,57) Although the identity of the conjugase has not yet been determined, a FACs hydrolase has been identified as aminoacylase (L-ACY-1) in the Heliothis virescens gut lumen, and its specific expression has been suggested to explain the species-specific FAC patterns.Citation44,58,59) Concerning the hydroxylation of the 17th position of the fatty acids, neither the function nor the enzyme have been determined. An efficient incorporation of free oxygen into volicitin was demonstrated in vitro by an incubation experiment using 18O2, suggesting that a monooxygenase may be involved in the biosynthesis.Citation60)
Fig. 3. A model summarizing nitrogen assimilation and FAC metabolism in the S. litura caterpillar.
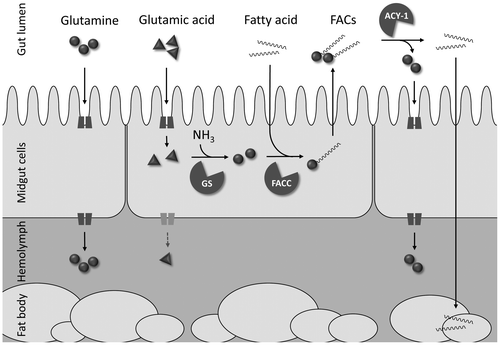
Of 29 lepidopteran species screened, regardless of tritrophic interactions, FACs were found in 19 of these species,Citation50) strongly suggesting that FACs are more commonly synthesized in a broad range of lepidopteran caterpillars than was previously known (Table ). The constraint of only glutamine and glutamate moieties was also observed to be consistent among the FACs found in lepidopteran caterpillars. Furthermore, glutamine conjugates, type (i), appeared to be the orthodox FACs structure, and are found in all FAC-producing species. Comparing the diversity of FACs with lepidopteran phylogeny indicates that glutamic acid conjugates (ii) can be synthesized by relatively primitive species, while hydroxylation of fatty acids (iii, iv) is limited to mostly larger and more developed macrolepidopteran species. Citation50)
Table 1. FACs composition in insects.
As shown in Table , FACs have been reported to be major components in the gut tracts of Drosophila melanogaster and the cricket Teleogryllus taiwanemma, although the physiological function of FACs in insects outside of Lepidoptera remains completely unknown.Citation61) The profile of FAC analogs in these two insects was similar to that of the tobacco hornworm M. sexta, predominantly utilizing glutamic acid conjugates over glutamine conjugates. However, a clear substrate preference toward glutamine was commonly displayed in an in vitro biosynthesis assay using crude enzyme extract from the midgut tissues of these tested insects.Citation62) The determination of the FAC glutamine/glutamate ratio likely differs by insect species. Moreover, in crickets, an additional unique biosynthetic pathway for glutamic acid conjugates was demonstrated. T. taiwanemma converts glutamine conjugates to glutamic acid conjugates by deaminating the side chain of the glutamine moiety. Such a pathway is apparently absent in other insects.Citation62) At this point, we are unable to rule out the possibility that FACs in these insects are a result of convergent evolution, but it is more likely that the ancestral insects utilized glutamine conjugates and crickets, and other insects developed glutamic acid conjugates in a different manner.
III. Development of tritrophic interactions
It is known that in general, higher plants, even those that do not respond to known elicitors, can recognize attack by pests and show defense responses. The concept of “damaged-self recognition” was proposed to explain this universal phenomenon by suggesting that plants recognize fragmented motifs of plant-origin as a sign of being destroyed.Citation63) Systemin represents this case in that the peptide contains plant-derived motifs, and cell wall fragments such as pectins, oligosaccharides, and glycopeptides form a large group among the known plant defense elicitors.Citation64–66) Herbivore-associated elicitors such as FACs, caeliferins, and inceptins also contain plant-derived units, but modifications by insects are of more importance. None of these elicitors can arise without being first processed by insect metabolic processes, and this assures the plant of being under herbivore attack. This allows plants to boost the specific defense response that is more critically effective against the insects.
How were plants able to recognize an elicitor in the beginning, if the compound was completely new? Inceptins are an understandable case because they originate from plant proteins, therefore, the receptor could have been derived from a damaged self recognition system. However, FACs appear to be quite alien in plants. One possibility is that the interaction mediated by FACs originated in an ancestral interaction between certain plants and microbes. N-Acyl amino acids are quite commonly distributed in microorganisms and are similar in structure to FACs.Citation67,68) Although some of them have been reported to function as biosurfactants, their functions remain mostly unknown. As is expected, several microbes that can synthesize FACs were identified in the gut lumen of Spodoptera exigua, and recently, the hydrolase of FACs was identified to be a novel Dps protein (DNA binding protein from starved cell).Citation51,69) This enzyme can also catalyze synthesis, but did not show amino acid specificity, conjugating most proteinogenic amino acids, while naturally occurring FACs are synthesized glutamine-specific in this lepidopteran species.Citation69) Microbial proteins may play a role at least in the homeostasis of FACs in insects,Citation70) but the finding is rather interesting in that it provides a technical justification for the involvement of ancestral microbes in biosynthesis of a predecessor of FACs. As described in the first chapter, plants are involved in various interactions with microbes and have receptors to recognize elicitors, and there are similarities between the signal transduction components plants utilize to respond to insects and microbes (Fig. ).Citation23,37) These observations may partially support the bold hypothesis that an ancestral receptor of microbial N-acyl amino acids had an affinity for insect FACs, accidentally leading to plants releasing VOCs that serve as exploitable information for foraging parasitoids.
Aside from this speculation, it is well known that plants have the ability to discriminate among insect FACs. Artificial FACs synthesized with amino acids other than glutamine or glutamate showed no elicitor activity in a corn seedling assay.Citation71) This result is consistent with the fact that the amino acid moiety of the known insect FACs is limited to glutamine/glutamate, and no exception has been discovered thus far.Citation50,61) Furthermore, different plant species have slight differences in the recognition of the structural variation of FACs. Corn plants do not react as strongly to N-linolenoyl-L-glutamic acid as they do to the corresponding glutamine analog, while eggplants react to both amino acid analogs, and release equal amounts of VOCs.Citation72) Hydroxylation on the 17th position of the fatty acid moiety is known to significantly affect elicitor activity,Citation71,72) and this may explain why volicitin (N-[17-hydroxylinolenoyl]-L-glutamine) was first identified as a VOC-inducing elicitor.Citation11) The enhanced response to the hydroxylation was not only observed in corn plants but also in solanaceous plants, such as tobacco and eggplants.Citation72) Interestingly, hydroxylated FACs have been found only in Macrolepidoptera, which is considered to be the most advanced lepidopteran group.Citation50) Whether plants discriminate hydroxylated FACs as an indicator of a special threat remains to be clarified. An important related question is whether a plant species could have customized this detection and response system fit to its pests of interest. Identification of N-(18-hydroxylinolenoyl)-L-glutamine (18OH-volicitin), a novel and unique FAC from M. sexta, enabled examination of this hypothesis.Citation72) M. sexta is a pest of various plants in the family Solanaceae, but does not feed on corn plants. Assuming 18OH-volicitin is an elicitor that corn plants have never experienced (adapted to), the bioassay was conducted to see how the plants react to the novel FAC. In the tobacco and eggplant assay, there was no significant difference in the elicitor activity of 17OH- and 18OH-volicitin, while the corn plants clearly “ignored” the hydroxylation on the 18th position, and released the same amount of VOCs when treated with normal N-linolenoyl-L-glutamine.Citation72) The observed results should be carefully examined with a variety of cultivars because domestication and bleeding process may have affected the total amounts and profiles of emitted VOCs.Citation73–75)
Heil et al. pointed out that although sensing insect-derived molecules may enable plants to detect herbivores at the species level, these molecules would be under the full evolutionary control of the herbivore. And if resistance induction is based only on specific recognition, the herbivore can change the molecular structure to avoid being sensed, or simply avoid applying the chemical on the wounded plant tissues.Citation63,76) As expected, some herbivorous species have actually gained this advantage in the arms race. Larvae of the specialist herbivore Heliothis subflexa avoid the production of active FAC elicitors by feeding on the fruits of their host plant, Physalis angulata, which lacks linolenic acid.Citation46) Because linolenic acid is required for the development of most insects, overcoming this nutritional deficiency requires a costly metabolic alteration. In other words, having FACs was a strategic option worth the cost for this species. Other studies showed some insects have additional chemicals (effectors) to disturb the plant defense mechanism triggered by FACs.Citation77,78) Furthermore, quite a few lepidopteran species do not utilize FACs. One-third of the species so far tested have no FACs, and these species were not phylogenetically related.Citation50) Those lepidopteran insects with no FACs tend to have tactics in defending themselves against enemies. Some species sequester or synthesize toxins, while others make nests to hide themselves although there are always exceptions. Considering that the putative function of FACs is to enhance nitrogen assimilation,Citation55) it is no wonder that insects employing the FACs strategy may be able to “grow fast and escape”. This strategy is successful only when the nutrition/deterrent ratio in the host plants are generous enough to the insect. Thus, whether or not an insect may have FACs seems partly dependent on the nutritional value of the host plants.
IV. Perspectives
Only a handful of plant species are reported to release VOCs in response to FACs,Citation16,23) in contrast to our finding that two-thirds of lepidopteran species so far tested had FACs.Citation50) Whether other host plants lost the recognition system for FACs, or such a system was obtained by only a limited number of plant species, will be investigated once the putative FACs receptor is identified.
The FAC conjugase in insects is another key player that needs to be determined. Identification, phylogenetic comparison, and regulation of gene expression of these two proteins will provide further insights into the network of plant and FAC having insects as well as the evolutionary aspects of the sophisticated tritrophic interactions.
Funding
This work was supported by JSPS KAKENHI [grant number 26850063], [grant number 23880014].
Acknowledgments
The author thanks Professor Naoki Mori (Kyoto University) and Professor James H. Tumlinson (Penn State University) for their guidance and encouragement throughout the course of my research. I also thank my collaborators and colleagues, Dr Irmgard Seidl-Adams for kind support, helpful comments, and discussions.
Disclosure statement
No potential conflict of interest was reported by the author.
Notes
† This review was written in response to the author’s receipt of the JSBBA Award for Young Scientists in 2015.
References
- Stowe MK, Turlings TCJ, Loughrin JH, et al. The chemistry of eavesdropping, alarm, and deceit. Proc. Natl. Acad. Sci. USA. 1995;92:23–28.10.1073/pnas.92.1.23
- Hansson BS, Stensmyr MC. Evolution of insect olfaction. Neuron. 2011;72:698–711.10.1016/j.neuron.2011.11.003
- Mumm R, Dicke M. Variation in natural plant products and the attraction of bodyguards involved in indirect plant defense. Can. J. Zool. 2010;88:628–667.10.1139/Z10-032
- Mattiacci L, Dicke M, Posthumus MA. β-Glucosidase: an elicitor of herbivore-induced plant odor that attracts host-searching parasitic wasps. Proc. Natl. Acad. Sci. USA. 1995;92:2036–2040.10.1073/pnas.92.6.2036
- Dicke M, Van Beek TA, Posthumus MA, et al. Isolation and identification of volatile kairomone that affects acarine predatorprey interactions involvement of host plant in its production. J. Chem. Ecol. 1990;16:381–396.10.1007/BF01021772
- Boland W, Feng Z, Donath J, et al. Are acyclic C11 and C16 homoterpenes plant volatiles indicating herbivory? Naturwissenschaften. 1992;79:368–371.10.1007/BF01140183
- Pare PW, Tumlinson JH. De novo biosynthesis of volatiles induced by insect herbivory in cotton plants. Plant Physiol. 1997;114:1161–1167.
- Alborn HT, Turlings TCJ, Jones TH, et al. An elicitor of plant volatiles from beet armyworm oral secretion. Science. 1997;276:945–949.10.1126/science.276.5314.945
- Spiteller D, Pohnert G, Boland W. Absolute configuration of volicitin, an elicitor of plant volatile biosynthesis from lepidopteran larvae. Tetrahedron Lett. 2001;42:1483–1485.10.1016/S0040-4039(00)02290-5
- Pare PW, Alborn HT, Tumlinson JH. Concerted biosynthesis of an insect elicitor of plant volatiles. Proc. Natl. Acad. Sci. USA. 1998;95:13971–13975.10.1073/pnas.95.23.13971
- Alborn HT, Jones TH, Stenhagen GS, et al. Identification and synthesis of volicitin and related components from beet armyworm oral secretion. J. Chem. Ecol. 2000;26:203–220.10.1023/A:1005401814122
- Roda A, Halitschke R, Steppuhn A, et al. Individual variability in herbivore-specific elicitors from the plant’s perspective. Mol. Ecol. 2004;13:2421–2433.10.1111/j.1365-294X.2004.02260.x
- Kaur H, Heinzel N, Schottner M, et al. R2R3-NaMYB8 regulates the accumulation of phenylpropanoid-polyamine conjugates, which are essential for local and systemic defense against insect herbivores in Nicotiana attenuata. Plant Physiol. 2010;152:1731–1747.10.1104/pp.109.151738
- Dinh ST, Baldwin IT, Galis I. The HERBIVORE ELICITOR-REGULATED1 gene enhances abscisic acid levels and defenses against herbivores in Nicotiana attenuata plants. Plant Physiol. 2013;162:2106–2124.10.1104/pp.113.221150
- Huffaker A, Pearce G, Veyrat N, et al. Plant elicitor peptides are conserved signals regulating direct and indirect antiherbivore defense. Proc. Natl. Acad. Sci. USA. 2013;110:5707–5712.10.1073/pnas.1214668110
- Schmelz EA. Impacts of insect oral secretions on defoliation-induced plant defense. Curr. Opin. Insect Sci. 2015;9:7–15.10.1016/j.cois.2015.04.002
- Bonaventure G. Perception of insect feeding by plants. Plant Biol. 2012;14:872–880.10.1111/plb.2012.14.issue-6
- Seidl-Adams I, Richter A, Boomer KB, et al. Emission of herbivore elicitor-induced sesquiterpenes is regulated by stomatal aperture in maize (Zea mays) seedlings. Plant Cell Environ. 2015;38:23–34.10.1111/pce.2015.38.issue-1
- Junker RR, Tholl D. Volatile organic compound mediated interactions at the plant-microbe interface. J. Chem. Ecol. 2013;39:810–825.10.1007/s10886-013-0325-9
- Gershenzon J, Dudareva N. The function of terpene natural products in the natural world. Nat. Chem. Biol. 2007;3:408–414.10.1038/nchembio.2007.5
- Huffaker A, Kaplan F, Vaughan MM, et al. Novel acidic sesquiterpenoids constitute a dominant class of pathogen-induced phytoalexins in maize. Plant Physiol. 2011;156:2082–2097.10.1104/pp.111.179457
- Huang M, Sanchez-Moreiras AM, Abel C, et al. The major volatile organic compound emitted from Arabidopsis thaliana flowers, the sesquiterpene (E)-β-caryophyllene, is a defense against a bacterial pathogen. New Phytol. 2012;193:997–1008.10.1111/j.1469-8137.2011.04001.x
- Bonaventure G, VanDoorn A, Baldwin IT. Herbivore-associated elicitors: FAC signaling and metabolism. Trends Plant Sci. 2011;16:294–299.10.1016/j.tplants.2011.01.006
- Zipfel C. Plant pattern-recognition receptors. Trends Immunol. 2014;35:345–351.10.1016/j.it.2014.05.004
- Mithofer A, Boland W. Recognition of herbivory-associated molecular patterns. Plant Physiol. 2008;146:825–831.10.1104/pp.107.113118
- Maffei ME, Mithöfer A, Boland W. Before gene expression: early events in plant-insect interaction. Trends Plant Sci. 2007;12:310–316.10.1016/j.tplants.2007.06.001
- Kanchiswamy CN, Takahashi H, Quadro S, et al. Regulation of Arabidopsis defense responses against Spodoptera littoralis by CPK-mediated calcium signaling. BMC Plant Biol. 2010;10:97.10.1186/1471-2229-10-97
- Wu J, Hettenhausen C, Meldau S, Baldwin IT. Herbivory rapidly activates MAPK signaling in attacked and unattacked leaf regions but not between leaves of Nicotiana attenuata. Plant Cell. 2007;19:1096–1122.10.1105/tpc.106.049353
- Schmelz EA, Engelberth J, Alborn HT, et al. Phytohormone-based activity mapping of insect herbivore-produced elicitors. Proc. Natl. Acad. Sci. USA. 2009;106:653–657.10.1073/pnas.0811861106
- Skibbe M, Qu N, Galis I, et al. Induced plant defenses in the natural environment: Nicotiana attenuata WRKY3 and WRKY6 coordinate responses to herbivory. Plant Cell. 2008;20:1984–2000.10.1105/tpc.108.058594
- Truitt CL, Wei HX, Paré PW. A plasma membrane protein from Zea mays binds with the herbivore elicitor volicitin. Plant Cell. 2004;16:523–532.10.1105/tpc.017723
- Maffei M, Bossi S, Spiteller D, et al. Effects of feeding Spodoptera littoralis on lima bean leaves. I. Membrane potentials, intracellular calcium variations, oral secretions, and regurgitate components. Plant Physiol. 2004;134:1752–1762.10.1104/pp.103.034165
- Maischak H, Grigoriev PA, Vogel H, et al. Oral secretions from herbivorous lepidopteran larvae exhibit ion channel-forming activities. FEBS Lett. 2007;581:898–904.10.1016/j.febslet.2007.01.067
- Wu J, Wang L, Wünsche H, et al. Narboh D, a respiratory burst oxidase homolog in Nicotiana attenuata, is required for late defense responses after herbivore attack. J. Integr. Plant Biol. 2013;55:187–198.10.1111/jipb.2013.55.issue-2
- Kallenbach M, Alagna F, Baldwin IT, et al. Nicotiana attenuata SIPK, WIPK, NPR1, and fatty acid-amino acid conjugates participate in the induction of jasmonic acid biosynthesis by affecting early enzymatic steps in the pathway. Plant Physiol. 2010;152:96–106.10.1104/pp.109.149013
- von Dahl CC, Winz RA, Halitschke R, et al. Tuning the herbivore-induced ethylene burst: the role of transcript accumulation and ethylene perception in Nicotiana attenuata. Plant J. 2007;51:293–307.10.1111/j.1365-313X.2007.03142.x
- Wu J, Baldwin IT. Herbivory-induced signalling in plants: perception and action. Plant Cell Environ. 2009;32:1161–1174.10.1111/pce.2009.32.issue-9
- Boudsocq M, Sheen J. CDPKs in immune and stress signaling. Trends Plant Sci. 2013;18:30–40.10.1016/j.tplants.2012.08.008
- Bonaventure G, Baldwin IT. Transduction of wound and herbivory signals in plastids. Commun. Integr. Biol. 2010;3:313–317.10.4161/cib.3.4.11834
- Alborn HT, Hansen TV, Jones TH, et al. Disulfooxy fatty acids from the American bird grasshopper Schistocerca americana, elicitors of plant volatiles. Proc. Natl. Acad. Sci. USA. 2007;104:12976–12981.10.1073/pnas.0705947104
- Schmelz EA, Carroll MJ, LeClere S, et al. Fragments of ATP synthase mediate plant perception of insect attack. Proc. Natl. Acad. Sci. USA. 2006;103:8894–8899.10.1073/pnas.0602328103
- Schmelz EA, LeClere S, Carroll MJ, et al. Cowpea chloroplastic ATP synthase is the source of multiple plant defense elicitors during insect herbivory. Plant Physiol. 2007;144:793–805.10.1104/pp.107.097154
- Pohnert G, Jung V, Haukioja E, et al. New fatty acid amides from regurgitant of lepidopteran (Noctuidae, Geometridae) caterpillars. Tetrahedron. 1999;55:11275–11280.10.1016/S0040-4020(99)00639-0
- Mori N, Alborn HT, Teal PEA, et al. Enzymatic decomposition of elicitors of plant volatiles in Heliothis virescens and Helicoverpa zea. J. Insect Physiol. 2001;47:749–757.10.1016/S0022-1910(00)00171-2
- Mori N, Yoshinaga N, Sawada Y, et al. Identification of volicitin-related compounds from the regurgitant of lepidopteran caterpillars. Biosci. Biotechnol. Biochem. 2003;67:1168–1171.10.1271/bbb.67.1168
- De Moraes CM, Mescher MC. Biochemical crypsis in the avoidance of natural enemies by an insect herbivore. Proc. Natl. Acad. Sci. USA. 2004;101:8993–8997.10.1073/pnas.0403248101
- Alborn HT, Brennan MM, Tumlinson JH. Differential activity and degradation of plant volatile elicitors in regurgitant of tobacco hornworm (Manduca sexta) larvae. J. Chem. Ecol. 2003;29:1357–1372.10.1023/A:1024209302628
- Halitschke R, Schittko U, Pohnert G, et al. Molecular interactions between the specialist herbivore Manduca sexta (Lepidoptera, Sphingidae) and its natural host Nicotiana attenuata. III. Fatty acid-amino acid conjugates in herbivore oral secretions are necessary and sufficient for herbivore-specific plant responses. Plant Physiol. 2001;125:711–717.10.1104/pp.125.2.711
- Aboshi T, Yoshinaga N, Noge K, et al. Efficient incorporation of unsaturated fatty acids into volicitin-related compounds in Spodoptera litura (Lepidoptera: Noctuidae). Biosci. Biotechnol. Biochem. 2007;71:607–610.10.1271/bbb.60546
- Yoshinaga N, Alborn HT, Nakanishi T, et al. Fatty acid-amino acid conjugates diversification in lepidopteran caterpillars. J. Chem. Ecol. 2010;36:319–325.10.1007/s10886-010-9764-8
- Spiteller D, Dettner K, Boland W. Gut bacteria may be involved in interactions between plants, herbivores and their predators: microbial biosynthesis of N-acylglutamine surfactants as elicitors of plant volatiles. Biol. Chem. 2000;381:755–762.
- Spiteller D, Boland W. N-(17-Acyloxy-acyl)-glutamines: novel surfactants from oral secretions of lepidopteran larvae. J. Org. Chem. 2003;68:8743–8749.10.1021/jo0342525
- Yoshinaga N, Sawada Y, Nishida R, et al. Specific incorporation of L-glutamine into volicitin in the regurgitant of Spodoptera litura. Biosci. Biotechnol. Biochem. 2003;67:2655–2657.10.1271/bbb.67.2655
- Yoshinaga N, Morigaki N, Matsuda F, et al. In vitro biosynthesis of volicitin in Spodoptera litura. Insect Biochem. Mol. Biol. 2005;35:175–184.10.1016/j.ibmb.2004.11.002
- Yoshinaga N, Aboshi T, Abe H, et al. Active role of fatty acid amino acid conjugates in nitrogen metabolism in Spodoptera litura larvae. Proc. Natl. Acad. Sci. USA. 2008;105:18058–18063.10.1073/pnas.0809623105
- Lait CG, Alborn HT, Teal PE, et al. Rapid biosynthesis of N-linolenoyl-L-glutamine, an elicitor of plant volatiles, by membrane-associated enzyme(s) in Manduca sexta. Proc. Natl. Acad. Sci. USA. 2003;100:7027–7032.10.1073/pnas.1232474100
- Tumlinson JH, Lait CG. Biosynthesis of fatty acid amide elicitors of plant volatiles by insect herbivores. Arch. Insect Biochem. Physiol. 2005;58:54–68.10.1002/(ISSN)1520-6327
- Kuhns EH, Seidl-Adams I, Tumlinson JH. A lepidopteran aminoacylase (L-ACY-1) in Heliothis virescens (Lepidoptera: Noctuidae) gut lumen hydrolyzes fatty acid-amino acid conjugates, elicitors of plant defense. Insect Biochem. Mol. Biol. 2012;42:32–40.10.1016/j.ibmb.2011.10.004
- Kuhns EH, Seidl-Adams I, Tumlinson JH. Heliothine caterpillars differ in abundance of a gut lumen aminoacylase (L-ACY-1)-suggesting a relationship between host preference and fatty acid amino acid conjugate metabolism. J. Insect Physiol. 2012;58:408–412.10.1016/j.jinsphys.2012.01.003
- Ishikawa C, Yoshinaga N, Aboshi T, et al. Efficient incorporation of free oxygen into volicitin in Spodoptera litura common cutworm larvae. Biosci. Biotechnol. Biochem. 2009;73:1883–1885.10.1271/bbb.90207
- Yoshinaga N, Aboshi T, Ishikawa C, et al. Fatty acid amides, previously identified in caterpillars, found in the cricket Teleogryllus taiwanemma and fruit fly Drosophila melanogaster larvae. J. Chem. Ecol. 2007;33:1376–1381.10.1007/s10886-007-9321-2
- Yoshinaga N, Abe H, Morita S, et al. Plant volatile eliciting FACs in lepidopteran caterpillars, fruit flies, and crickets: a convergent evolution or phylogenetic inheritance? Front. Physiol. 2014;5:121.
- Heil M. Damaged-self recognition in plant herbivore defence. Trends Plant Sci. 2009;14:356–363.10.1016/j.tplants.2009.04.002
- Doares SH, Syrovets T, Weiler EW, et al. Oligogalacturonides and chitosan activate plant defensive genes through the octadecanoid pathway. Proc. Natl. Acad. Sci. USA. 1995;92:4095–4098.10.1073/pnas.92.10.4095
- Bergey DR, Orozco-Cardenas M, de Moura DS, et al. A wound- and systemin-inducible polygalacturonase in tomato leaves. Proc. Natl. Acad. Sci. USA. 1999;96:1756–1760.10.1073/pnas.96.4.1756
- Creelman RA, Mullet JE. Oligosaccharins, brassinolides, and jasmonates: nontraditional regulators of plant growth, development, and gene expression. Plant Cell. 1997;9:1211–1223.10.1105/tpc.9.7.1211
- Brady SF, Clardy J. Long-chain N-acyl amino acid antibiotics isolated from heterologously expressed environmental DNA. J. Am. Chem. Soc. 2000;122:12903–12904.10.1021/ja002990u
- Peypoux F, Laprévote O, Pagadoy M, et al. N-acyl derivatives of Asn, new bacterial N-acyl D-amino acids with surfactant activity. Amino Acids. 2004;26:209–214.
- Ping L, Büchler R, Mithöfer A, et al. A novel Dps-type protein from insect gut bacteria catalyses hydrolysis and synthesis of N-acyl amino acids. Environ. Microbiol. 2007;9:1572–1583.10.1111/emi.2007.9.issue-6
- Funke M, Büchler R, Mahobia V, et al. Rapid hydrolysis of quorum-sensing molecules in the gut of lepidopteran larvae. ChemBioChem. 2008;9:1953–1959.10.1002/cbic.v9:12
- Sawada Y, Yoshinaga N, Fujisaki K, et al. Absolute configuration of volicitin from the regurgitant of lepidopteran caterpillars and biological activity of volicitin-related compounds. Biosci. Biotechnol. Biochem. 2006;70:2185–2190.10.1271/bbb.60133
- Yoshinaga N, Ishikawa C, Seidl-Adams I, et al. N-(18-hydroxylinolenoyl)-L-glutamine: a newly discovered analog of volicitin in Manduca sexta and its elicitor activity in plants. J. Chem. Ecol. 2014;40:484–490.10.1007/s10886-014-0436-y
- Gouinguené S, Degen T, Turlings TCJ. Variability in herbivore-induced odour emissions among maize cultivars and their wild ancestors (teosinte). Chemoecology. 2001;11:9–16.10.1007/PL00001832
- Degen T, Dillmann C, Marion-Poll F, et al. High genetic variability of herbivore-induced volatile emission within a broad range of maize inbred lines. Plant Physiol. 2004;135:1928–1938.10.1104/pp.104.039891
- Maag D, Erb M, Bernal JS, et al. Maize domestication and anti-herbivore defences: leaf-specific dynamics during early ontogeny of maize and its wild ancestors. PLoS One. 2015;10:e0135722.10.1371/journal.pone.0135722
- Heil M, Ibarra-Laclette E, Adame-Álvarez RM, et al. How plants sense wounds: damaged-self recognition is based on plant-derived elicitors and induces octadecanoid signaling. PLoS One. 2012;7:e30537.10.1371/journal.pone.0030537
- Felton GW, Tumlinson JH. Plant-insect dialogs: complex interactions at the plant-insect interface. Curr. Opin. Plant Biol. 2008;11:457–463.10.1016/j.pbi.2008.07.001
- Delphia CM, Mescher MC, Felton GW, et al. The role of insect-derived cues in eliciting indirect plant defenses in tobacco, Nicotiana tabacum. Plant Signal Behav. 2006;1:243–250.10.4161/psb.1.5.3279