Abstract
Identifying substandard tissue-engineered oral mucosa grafts with a poor epithelium before clinical use is critical to ensure quality assurance/control in regenerative medicine, leading to success of grafting. This study investigated the effects of one of the C-xylopyranoside derivatives, β-D-xylopyranoside-n-propane-2-one (XPP), on oral epithelial regeneration. Using a three-dimensional oral mucosa model, we analyzed changes of the epithelial structure, glycosaminoglycan (GAG) synthesis, the expression levels of basement membrane zone markers, and substrates of Akt/mTOR signaling. Compared with the control, 2 mM XPP treatment increased the mean and minimal epithelial thickness, and reduced the variation of epithelial thickness. It also stimulated expressions of decorin and syndecan-1 with change of GAG amount and/or composition, and enhanced the expressions of integrin α6, CD44, and Akt/mTOR signaling substrates. These findings suggest that XPP supplementation contributes to consistent epithelial regeneration. Moreover, upregulation of those markers may play a role in increasing the quality of the oral mucosal epithelium.
Graphical abstract
XPP had an effect on consistent epithelial regeneration in an in vitro 3D oral mucosa model (A: Histologic examination, B: Histomorphometric analysis).
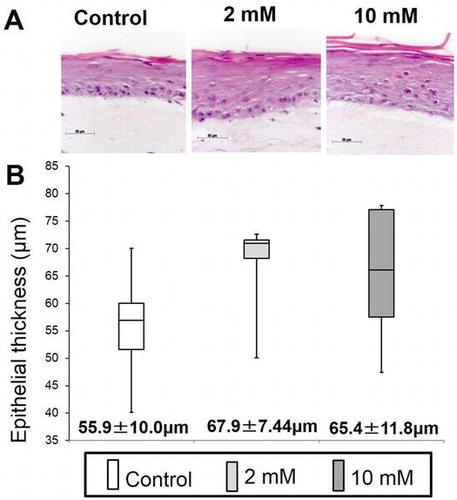
A tissue-engineered oral mucosa (TEOM) for human clinical applications, such as ex vivo produced oral mucosa equivalents (EVPOME), has been manufactured under current good manufacturing practices with multiple processing and testing steps.Citation1–3) The final product testing and release criteria that ensure the quality assurance/control of tissue-engineered products have been challenging in regenerative medicine (RM).Citation4,5) In our case, manufacture of an EVPOME can result in the generation of substandard grafts with a poor/thin epithelium, which is inevitable when manufacturing multiple pieces.Citation3) Transplantation of substandard EVPOME grafts impedes wound healing in vivo due to poor barrier function, insufficient cytokine secretion, and a predisposition to epithelial exfoliation. To avoid it, there should be two ways. One is to develop a non-invasive method for detecting substandard grafts prior to product release, and the other is a pharmacologic manipulation to improve the ability to regenerate epithelium to enhance the epithelial quality of the TEOMs to a level acceptable for clinical use.Citation6,7)
A thin epithelium in native oral mucosa, resulting from atrophic changes, is a characteristic of aging.Citation8–10) This change is consistent with epidermal thinning, implying intrinsic skin aging that involves changes in the extracellular matrix (ECM) and basement membrane zone (BMZ).Citation11) Although the mechanism of age-related epithelial atrophy is not well elucidated, Oh et al., reported that the total content of sulfated glycosaminoglycans (s-GAGs) and the perlecan expression in the BMZ are significantly reduced in older skin tissue samples compared with younger samples.Citation12,13) These findings clearly indicate that GAGs and related proteoglycans (PGs) are involved in various structural and physiologic regulatory functions in skin.
Recent studies demonstrated that enhancing GAG synthesis and its bioactivity in dermal fibroblasts by C-xylopyranoside derivatives, as glycosyltransferase inhibitors, can initiate an increase in s-GAG synthesis.Citation14–17) Furthermore, histologic examinations also demonstrated the biologic effects of C-xylopyranoside derivatives to ameliorate the structural changes of aged skin.Citation18–20) As such compounds appear to be beneficial to oral mucosa aging, we hypothesized that they could enhance GAG production in oral mucosa cells. Consequently, they contribute to providing consistent epithelial thickness within TEOM/EVPOMEs to prevent the generation of substandard grafts.
The primary objective of this study was to assess whether supplementation of one of the C-xylopyranoside derivatives, β-D-xylopyranoside-n-propane-2-one (XPP), facilitates GAG synthesis and epithelial regeneration using an in vitro, three-dimensional, oral mucosa model (3DOMM), in which oral keratinocytes were cultured on top of the fibroblast-populated collagen lattice. To examine the effects of XPP, we performed histomorphometric analysis of the epithelial tissue in the 3DOMMs and GAG analyses of the 3DOMMs as well as the culture supernatants. We, then, assessed the expression level of BMZ markers and substrates of the Akt/mTOR signaling pathway because the basement membrane and PGs in ECM play a pivotal role in epithelial homeostasis/regeneration.Citation21–25) These findings may provide a novel strategy for improving the quality assurance/control of cell–tissue-based products in RM as well as soft tissue engineering (TE).
Materials and methods
Procurement of oral mucosa samples
The protocol for obtaining human oral mucosa samples was approved by the Niigata University Faculty of the Dentistry Internal Review Board (25-R2-04-08). Patients subjected to minor dentoalveolar surgery were provided sufficient information regarding this study, and all participating individuals signed an informed consent form.
Primary oral keratinocyte (OK) and fibroblast (OF) cultures
At the Oral and Maxillofacial Surgery outpatient clinic at the Niigata University Medical & Dental Hospital, an oral mucosa sample was harvested from the area adjacent to the site of the surgical procedure without causing any morbidity. The sample was transported in a 15-mL conical tube containing 5 mL of basal EpiLife® (Life Technologies, Carlsbad, CA, USA) medium. Primary oral keratinocytes (OKs) and oral fibroblasts (OFs) cultures were established as described previously.Citation24) Briefly, a tissue specimen was transferred to a 0.025% trypsin/EDTA solution (Life Technologies) containing 1.5% Antibiotic–Antimycotic (Life Technologies) and soaked overnight at room temperature. OKs were mechanically dissociated from the underlying connective tissue in a 0.0125% defined trypsin inhibitor (DTI; Life Technologies), resuspended in “complete” EpiLife® supplemented with EpiLife Defined Growth Supplements (Life Technologies), 0.06 mM Ca2+, gentamicin (5.0 μg/mL), and amphotericin B (0.375 μg/mL; Life Technologies), and plated at a density of 4.0–5.0 × 104 cells/cm2. When the cells reached 70–80% confluence, they were re-plated at a density of 0.7–1.0 × 104 cells/cm2, and serially subcultured. OKs from passages 3 to 5 were used in the study.
Primary OF cultures were established by an explant culture technique. Small explants were placed in a 60-mm Petri dish (Corning, New York, NY, USA), and incubated in a moist atmosphere of 5% CO2 at 37 °C in Dulbecco’s modified Eagle medium (DMEM; Wako Pure Chemical Industries, Ltd., Osaka, Japan) supplemented with 10% fetal bovine serum (FBS; Nichirei, Tokyo, Japan), gentamicin, and amphotericin B. Cells were fed every three days. Once the cell outgrowth was sufficient, cells were re-plated in another culture vessel (0.8 × 104 cells/cm2), and serially subcultured. OFs from passages 3 to 5 were used in the study.
Pharmacologic reagents
XPP (Fig. ) was purchased from Wako Pure Chemical Industries Ltd., dissolved in EpiLife and DMEM basal media with gentle stirring in a water bath at the indicated final concentrations, and then filtered for cell culture.
Actinase E (protease from Streptomyces griseus) was purchased from Kaken Pharmaceutical Co. (Tokyo, Japan) and cellulase (from Aspergillus. niger) was from Sigma-Aldrich (St. Louis, MO, USA). Chondroitin 4-sulfate (Ch4S, from whale cartilage), chondroitin 6-sulfate (Ch6S, from shark cartilage), chondroitin (Ch, chemically desulfated Ch6S), and unsaturated disaccharide standards, and Ch B lyase (from Flavobacterium heparinum, EC 4.2.2) were from Seikagaku Biobusiness Co. (Tokyo, Japan). Heparin (Hep, from porcine intestinal mucosa) was purchased from Nacalai Tesque, Inc. (Kyoto, Japan). Hyaluronan (HA, from Streptococcus zooepidemicus) was from Food Chemifa (Tokyo, Japan). Ch ABC lyase (from Proteus vulgaris, EC 4.2.2.4), Hep lyase II (from Flavobacterium heparinum, no EC number), Hep lyase III (from Flavobacterium heparinum, EC 4.2.2.8), heparan sulfate (HS, from bovine kidney), and dermatan sulfate (DS, from porcine intestinal mucosa) were from Sigma-Aldrich. HA lyase (from Streptomyces hyalurolyticus, EC 4.2.2.1) was from Wako Pure Chemical Industries, Ltd. Cellulose acetate membrane was from Jookoo (Tokyo, Japan).
Cell viability
Cells (5 × 103 cells) were plated into 96-well microplates with 100 μL of complete EpiLife culture medium containing 0.06 mM Ca2+ (OKs) or DMEM supplemented with 10% FBS (OFs). At the first medium change, the OKs were fed with complete EpiLife containing either 0.06 mM Ca2+ or 1.2 mM Ca2+. Twenty-four hours later, the cells were fed the appropriate media with supplemented with 0 (control), 2, and 10 mM XPP. Using a Cell Counting Kit-8 (Dojindo Molecular Technologies, Osaka, Japan), cell viability was determined every 24 h according to the manufacturer’s instructions. Absorbance at 450 nm with a reference wavelength of 620 nm was measured using a Multiskan FC 96-well plate reader. (Thermo Fisher Scientific, Waltham, MA, USA). All assays were performed in triplicate.
Measurement of sulfated-glycosaminoglycans (s-GAGs)
Cells plated at a density of 1.5 × 105 were grown in six-well microplates with 3 mL complete EpiLife® culture medium containing either 0.06 mM Ca2+ or 1.2 mM Ca2+ (for OKs) and DMEM supplemented with 10% FBS (for OFs). Subsequently, 2 or 10 mM XPP was added to the media. After incubation with the reagents for 24 and 48 h, we measured the amount of s-GAGs released in 100 μL of culture media using a Blyscan assay kit (Biocolor, Carrickfergus, UK) according to the manufacturer’s instructions. Absorbance was measured at 650 nm with a reference wavelength of 570 nm using a Multiskan FC 96-well plate reader (Thermo Fisher Scientific). All assays were performed in triplicate. The amount of s-GAGs in the medium was calculated by the concentrations (μg/100 μL) multiplied by 30. The amount of s-GAGs in supernatants of three-dimensional oral mucosa models was also measured as described above, due to the sensitivity, which was calculated by the concentration multiplied by 180.
Fabrication of a three-dimensional oral mucosa model (3DOMM)
The 3DOMM was fabricated according to a previous study with minor modifications.Citation26) Briefly, after filling a six-well tissue culture insert (Greiner Bio, Roskilde, Denmark) with 1 mL acellular type I collagen matrix (Nitta gelatin, Osaka, Japan), a mixture of 3 mL collagen matrix solution and 5.0 × 105 OFs was placed on top of the solidified acellular collagen matrix. After the mixture solidified, 22 mL DMEM containing 10% FBS was added to create a submerged culture condition. Two days later, the mixture was detached from the tissue culture insert wall using a 100-μL micropipette and the culture was continued for another 5 days to allow the gel to contract completely.
Subsequently, OKs (5.0 × 105/50 μL of complete EpiLife® containing 0.06 mM Ca2+) were seeded onto the surface of the contracted collagen gel. All constructs were submerged in culture for the first 4 days with complete EpiLife® containing 0.06 mM Ca2+, followed by complete EpiLife® containing 1.2 mM Ca2+ for the another 3 days. This resulted in the formation of a continuous epithelial monolayer on the collagen gel. The cells were then raised to an air–liquid interface for epithelial stratification with 18 mL of complete EpiLife® containing 1.2 mM Ca2+ and cultured further for 7 days. The constructs were fed every other day with one of three media containing 0, 2, or 10 mM XPP, which were continuously supplemented during a total period of 21 days in culture.
3DOMM sample collection
After the fabrication of 3DOMMs treated with 0, 2, or 10 mM XPP was completed, the culture supernatants, not changed for the last 48 h, were collected and stored at −80 °C until measurement. Several whole constructs were immediately stored at −80 °C for the GAG analysis, and the others were split into two pieces. One piece was fixed with 4% paraformaldehyde in 0.1 M phosphate-buffered saline and embedded in paraffin for histologic examination and immunostaining. The other piece was lysed with RIPA buffer supplemented with protease and phosphatase inhibitor cocktails (Wako Pure Chemical Industries, Ltd.) using a homogenizer (Fisher Scientific, Waltham, MA, USA) to obtain entire 3DOMM extracts for the immunoblot analysis.
Histologic examinations and quantitative morphometric analysis
Paraffin-embedded sections were cut in sections 4.5 μm thick, and stained with hematoxylin and eosin (HE). To determine the thickness and number of cell layers within the epithelial layer of 3DOMM treated with 0, 2, or 10 mM XPP, the HE-stained sections were photographed using an Axioplan 2 imaging microscope (Carl Zeiss, Göttingen, Germany) through an AxioCam HRc digital camera (Carl Zeiss). Using the AxioVision software, the distance between the basal side of basal cells and the surface of the keratinized layer perpendicular to the interface between the epithelium and the underlying connective tissue as well as the number of cell layers was measured at 10 randomly selected points per section by two examiners in a blinded manner. The mean number of cell layers from the 10 measurements was assigned to represent each sample of 3DOMM.
Preparation of GAGs
3DOMMs and their culture supernatants were dried and treated with actinase E. Then, GAGs were liberated from PGs by treatment with cellulase (from Aspergillus niger) by its endo-β-xylosidase activity.Citation27) Uronic acid content of cell-associated GAGs, not their culture supernatants, was determined by the carbazole sulfuric acid method.Citation28)
Electrophoresis of GAGs on cellulose acetate membrane
GAGs (0.2 μg of uronic acid from a mixture of 2 lots) with or without treatment with lyases were electrophoresed on a cellulose acetate membrane in 0.1 M pyridine-0.47 M formic acid buffer (pH 3.0) at 1 mA/cm for 20 min. The membrane was stained with 0.05% Alcian Blue-20 mM MgCl2-70% ethanol.Citation29)
Unsaturated disaccharide composition analysis of GAGs
Unsaturated disaccharide compositions of GAGs (100 μg each from a mixture of 14 lots) were determined by HPLC after treatment with lyases; Ch ABC lyase, double digestion with Hep lyase II and Hep lyase III, Ch B lyase, or HA lyase.Citation30,31) All enzymatic reactions were performed exhaustively under optimal conditions and reactions stopped by boiling at 100 °C for 3 min. HPLC was performed on a Hitachi ELITE LaChrom system equipped with a UV–Vis detector (model L-2420; Hitachi, Tokyo, Japan) using a YMC-Pack Polyamine II column (4.6 × 250 mm, YMC Co., Kyoto, Japan). Unsaturated disaccharides generated by lyase treatment were eluted with a linear gradient of NaH2PO4 from 16 to 478.5 mM over 60 min at a flow rate of 1.0 ml/min at 30 °C. The eluate was monitored by UV absorbance at 232 nm.
Immunoblot analysis of 3DOMM
Equal amounts (10–30 μg) of protein per lane were resolved by sodium dodecyl-sulfate-polyacrylamide gel electrophoresis and electrophoretically transferred to Immobilon P membranes (Millipore, Darmstadt, Germany). The membranes were then incubated overnight at 4 °C with antibodies against: type IV collagen (1:1000; ab6586, Abcam, Cambridge, UK), laminin-5 (1:1000; ab14509, Abcam), nidogen-2 (1:1000; ab131279, Abcam), integrin α6 (1:200; sc-10730, Santa Cruz Biotechnology, Inc., Dallas, TX, USA), integrin β1 (1:500; #04-1109, Millipore, Billerica, MA, USA), decorin (1:400; ab67449, Abcam), syndecan-1 (1:100; MCA2459GA, AbD serotec, Raleigh, NC, USA), CD44 (1:5000; 04-1123, Millipore), p-Akt (1:1000; #4058, Cell Signaling Technology (CST), Danvers, MA, USA), Akt (1:1000; #9272,CST), p-S6 K (1:1000; #9234, CST), S6 K (1:1000; #2707, CST), p-S6 (1:1000; #4857, CST), S6 (1:1000; #2217, CST), or β-actin (1:2500; #4970, CST), followed by goat-anti mouse or rabbit antibodies (1:5000; GE Healthcare, Milwaukee, WI, USA). The signals were detected using the chemiluminescence reagent (Thermo Fisher Scientific). For semi-quantitative comparison of signals, membranes were scanned and quantified using Chemidoc (Biorad, Hercules, CA, USA).
Immunohistochemistry
Immunostaining using heat-induced epitope retrieval was performed as described previously.Citation32) The primary antibodies used in this study were as follows: type IV collagen (1:500), laminin (1:80; #10765, PROGEN Biotechnik, Heidelberg, Germany), integrin α6 (1:50) and integrin β1 (1:250), syndecan-1 (1:100), CD44 (1:100), p-S6 (1:75), S6 (1:100).
Statistical analysis
Results are presented as mean ± standard deviation (SD) of the mean of the values obtained. Comparisons among the three different groups treated with 0, 2, or 10 mM XPP were examined using a repeated one-way analysis of variance (ANOVA) after determining that the samples were normally distributed using Bartlett’s test. Multiple comparisons among subgroup were adjusted using the Tukey–Kramer post hoc test. A p-value of less than 0.05 was considered statistically significant.
Results
Cell viability
Regardless of the calcium concentrations, the cell viability of OKs treated with 2 or 10 mM XPP increased over time and did not differ significantly from that of untreated cells for up to 48 h (Fig. (A), (B)). Similarly, OFs with increased cell viability over time exhibited no significant differences between the treated and untreated groups, either (Fig. (C)). This finding suggested that the concentrations of XPP used in this study did not decrease OK or OF cell viability.
Fig. 2. Viability measurement of oral keratinocytes and fibroblasts in a monolayer culture supplemented with 0 (control), 2, or 10 mM XPP.
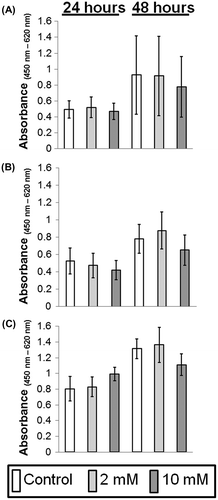
s-GAGs production by OKs and OFs cultured in monolayer
The s-GAGs production did not differ significantly over time among OKs treated with 0, 2, or 10 mM XPP in 0.06 mM Ca2+ medium (Fig. (A)). In contrast, OKs cultured in 1.2 mM Ca2+ medium secreted a significantly greater amount of s-GAG when incubated with 2 mM XPP for up to 48 h and 10 mM XPP for 48 h, compared with OKs without XPP treatment (Fig. (B)). For OFs, only the 10 mM XPP treatment for 48 h induced a significantly higher production of s-GAGs (Fig. (C)).
Fig. 3. Measurement of sulfated-glycosaminoglycans (s-GAG) of oral keratinocytes and fibroblasts in a monolayer culture supplemented with 0 (control), 2, or 10 mM XPP.
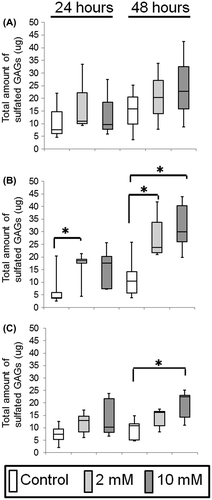
Quantitative analysis of epithelial morphology
Histology of all 3DOMMs revealed a continuous and well-differentiated oral epithelial layer with several viable cell layers and parakeratinization was developed on top of the OFs-populated dermal layer without any rete ridge formation (Fig. (A)). Although there were few distinct differences in the epithelial structures among the experimental groups, we analyzed the morphometric features of the epithelial layer. The epithelial thickness of cell layers quantitated in this study differed only marginally (p = 0.056 and p = 0.078, respectively) among the XPP treated and untreated groups (Fig. (B)). The number of cell layers within the epithelial layer did not differ significantly among groups (data not shown)
Fig. 4. Effect of XPP on epithelial regeneration in an in vitro 3D oral mucosa model (3DOMM).
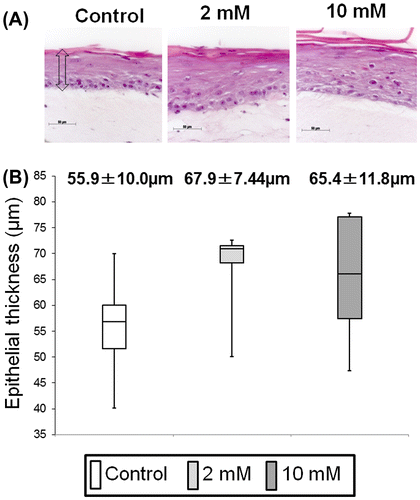
Secreted and cell-associated GAGs production from 3DOMMs
In 3DOMMs, the levels of s-GAGs in the supernatants measured by Blyscan assay treated with 2 or 10 mM XPP were significantly higher than those of the untreated group (Fig. ). However, the GAGs secreted from 3DOMMs were not discriminated from the XPP-derived GAGs. Based on uronic acid contents, cell-associated GAGs prepared from 3DOMMs showed a 20% increase in the presence of 2 mM XPP, compared with the control (812.2 μg versus 678.8 μg), indicating 2 mM XPP did not significantly affect the biosynthesis of cell-associated GAGs.
XPP affects the composition of cell-associated chondroitin sulfates (ChSs)
Cellulose acetate membrane electrophoresis of GAG chains prepared from 3DOMMs revealed that cell-associated GAGs migrated similarly regardless of the dose of XPP and the migration distances were similar to those of DS or HS (Fig. (A)). Electrophoresis patterns of GAGs from 2 mM XPP-treated cells after the treatment with lyases specific for GAG structures indicated that most of the GAGs are ChSs including DS, and a small portion are HS/Hep, but GAGs that are HA are almost undetectable (Fig. (B)). There was a similar pattern in 10 mM XPP-treated cells (data not shown). In the case of secreted GAGs, the detected band at 10 mM XPP was also close to the position of DS or HS (Fig. (C)). At 0, 2 mM XPP, no band was detected because the sample amount was lower than that of 10 mM XPP. We believe the reason for this was that we had to do the calculation for the samples on the basis of the Blyscan assay before GAG preparation as there were insufficient amounts.
Fig. 6. Electrophoresis of GAGs on cellulose acetate membrane.
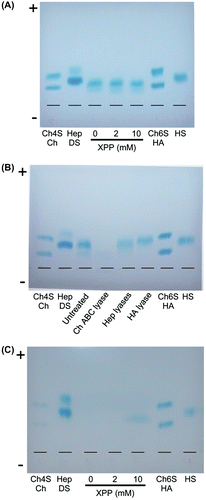
In order to further investigate the cell-associated GAG composition, unsaturated disaccharide composition analysis using HPLC was performed (Fig. ). From the totals of peak areas of detected disaccharides, unsaturated disaccharides derived from ChSs were 4.2 times in the absence of XPP or 3.8 times in the presence of 2 mM XPP more than those from HS/Hep (comparison Fig. (A) and (C), (E) and (G)). Unsaturated disaccharides derived from HA were not detected in this scale (Fig. (D) and (H)). Therefore, ΔDi-0Ss in Fig. A and E were almost all from ChS. The percentages of ΔDi-4Ss derived from DS in total ΔDi-4Ss were 69% and 81% in the absence of XPP and in the presence of 2 mM XPP, respectively (comparison Fig. (A) and (B), (E) and (F)). Disaccharide composition of ChS and HS/Hep are shown in Tables and . In the absence of XPP, ΔDi-4S is rich in ChS, and ΔDi-0S is rich in HS/Hep. At 2 mM XPP, ΔDi-4S decreased 16.9%, on the other hand, ΔDi-0S and ΔDi-6S increased 12.7 and 4.1%, respectively, in ChS composition. The ΔDi-4S from DS is decreased by 13% in the presence of 2 mM XPP (comparison Fig. (B) and (F)). These results suggest the possibility of the decrease of sulfation on ChSs including DS in the presence of XPP. In the case of HS/Hep, no significant composition change was observed.
Fig. 7. HPLC analysis of unsaturated disaccharides derived from cell-associated GAGs after lyase treatment.
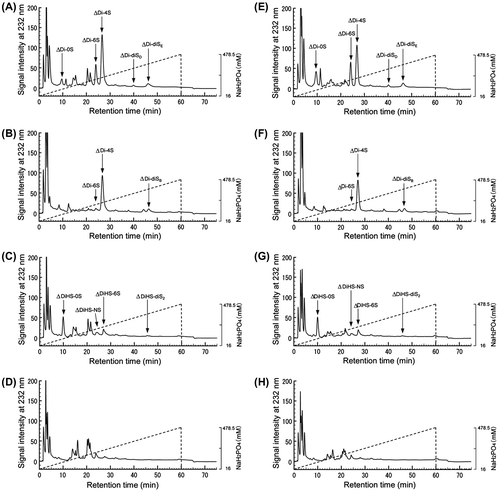
Table 1 Unsaturated disaccharide compositions of cell-associated ChS chains.
Table 2. Unsaturated disaccharide compositions of cell-associated HS/Hep chains.
Immunoblotting analysis of 3DOMMs
Although there were slight changes in the expression of the majority of BMZ markers between the 3DOMMs treated with 0 or 2 mM XPP, only the expression level of α6 integrin in the 2 mM XPP-treated 3DOMM was more than two fold greater (Fig. ). In addition, the expression levels of BMZ markers, such as type IV collagen, nidogen-2, and integrin α6 had a greater than 50% increase in the 3DOMMs treated with 10 mM XPP, compared with the control (Fig. ). The expression levels of decorin (CS/DSPG) and syndecan-1(HSPG) by the XPP treatment increased in a dose-dependent manner, however, both 2 and 10 mM XPP treatment groups exhibited a conspicuous increase in CD44 expression (Fig. ). Furthermore, Akt and S6 kinase were more phosphorylated in XPP-treated 3DOMMs than untreated ones while S6 was slightly phosphorylated in only 2 mM XPP-treated 3DOMMs (Fig. ).
Fig. 8. Expression of BMZ marker proteins. Immunoblotting detection of type IV collagen, laminin-5, nidogen-2, integrin α6, and integrin β1 in 3DOMMs treated with 0, 2, or 10 mM XPP.
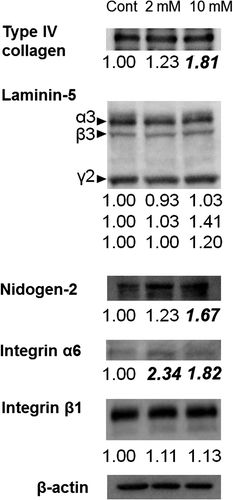
Fig. 9. Expression of PGs. Immunoblotting detection of decorin, syndecan-1, and CD44 in 3DOMMs treated with 0, 2, or 10 mM XPP.
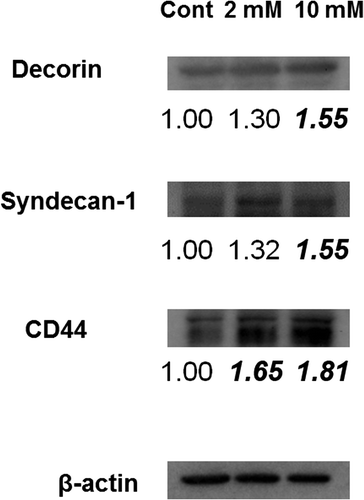
Fig. 10. Expression of Akt/mTOR signaling substrates. Immunoblotting detection of p-Akt, Akt, p-S6K, S6K, p-S6 and S6 in 3DOMMs treated with 0, 2, or 10 mM XPP.
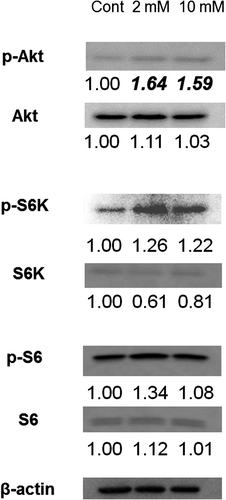
Immunohistochemistry
To confirm the immunoblotting analysis for 3DOMMs, we assessed the immunolocalization of the BMZ markers. The immunoreaction of type IV collagen and laminin, which were more intense in the XPP treatment groups, was localized in basal layer cells and the underlying extracellular matrix, while there was no clear linear expression pattern (Fig. (A), (B)). Integrin α6 was exclusively detected on the basal side of the basal cells, and the expression level was enhanced by the XPP supplementation, compared to the control group (Fig. (C)). Integrin β1 was immunolocalized in the basal layer cells and the underlying extracellular matrix, whereas the expression level of the XPP treatment group did not differ from that of the untreated control (Fig. (D)). The membrane PG syndecan-1 was expressed in the more cell layers within suprabasal layers in the XPP-treated 3DOMM than the control one (Fig. (E)). In addition, CD44 expression was confined to the cell membrane of the basal cell layers in the XPP-untreated 3DOMM, but more CD44-immunopositive cell layers were present in the XPP-treated 3DOMM (Fig. (F)). The immunoreactions of p-S6 localized in the upper suprabasal cell layer were more intense in the XPP-treated 3DOMMs than in the control, whereas the S6 expression was detected in almost the entire epithelium (Fig. (G), (H))
Fig. 11. Expression and immunolocalization of proteins used in immunoblot analysis in 3DOMMs treated with 0, 2, or 10 mM XPP.
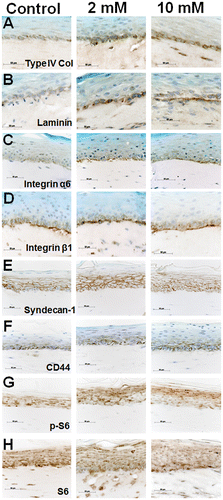
Discussion
GAGs/PGs, major ECM constituents, are bioactive macromolecules present in every mammalian tissue, and play important roles in intracellular signaling, tissue functions, and properties, including epithelial tissues.Citation33,34) TE/RM approaches have been suggested as promising strategies to (re)generate biologically functional tissues.Citation35) Thus, it is important to delineate the interactions of GAGs/PGs with cells. Diverse approaches integrating GAGs/PGs into the design of engineered constructs for soft tissues have been successfully applied.Citation36–41) However, further strategies are required to control and advance TE/RM.Citation36,42) Here, we highlighted the feasibility of C-xyloside to stimulate cells to synthesize GAGs/PGs and improve the epithelial regeneration in tissue-engineered constructs. This pharmacologic manipulation, for the first time, allows for its potential use for quality assurance/control of a cell-based product, leading to a novel insight into the regulatory framework in TE/RM.
Our results indicate that incubation with 2 or 10 mM XPP, a simple compound of C-xyloside, selected for use in this study due to the cost-effectiveness,Citation43) did not have any cytotoxic effects on OKs as well as OFs grown in a monolayer culture. We also showed higher s-GAGs levels in the culture media secreted from XPP-treated cells, basically, in both time- and dose-dependent manners, which is consistent with previous studies examining the effect of C-xyloside.Citation17,19,44)
The principal and significant finding of this study is that pharmacologic manipulation during 3DOMM fabrication in the presence of 2 or 10 mM XPP increased the mean and minimal epithelial thickness without disturbing the epithelial structure and differentiation. Of note, when incubated with 2 mM XPP, the epithelial thickness was more consistent as confirmed by its smaller interquartile range, compared with the other groups. Thus, 2 mM XPP treatment could be efficacious for preventing the development of a thin/poor epithelial layer that would not qualify for grafting and minimizing differences among individuals. This allows us to utilize this compound to decrease the variation of epithelial thickness of 3DOMMs that is beneficial to the quality assurance/control of cell-based products. Nonetheless, further studies are required to implement XPP supplementation to the current EVPOME manufacturing protocol because OFs are not included in the EVPOME grafts. OKs would behave differently in the culture condition without interactions with OFs.Citation45) In addition, it is important to assess the barrier functions and mechanical properties of the engineered epithelial layer by pharmacologic manipulation.Citation46–48)
We speculated elongated GAGs from xylose of supplemented XPP mainly elevated GAGs in the culture media, rather than increase in the secreted GAGs from 3DOMMs as stated by previous studies using monolayer cultures of epidermal cells.Citation16,17) Although an effect of free GAGs in the culture medium on the epithelial regeneration of 3DOMM needs to be elucidated, an increase in the epithelial thickness is highly likely to be due to the finding that the cell-associated GAGs are increased with an increase in the decorin and syndecan-1 expressions, in XPP-treated 3DOMMs, compared with untreated ones. In addition, not only the GAGs production, the change of GAGs profiles could affect the epithelial regeneration although the mechanism of how 2 mM XPP treatment was more efficacious to enhance the epithelial thickness and provide consistent epithelial thickness than 10 mM treatment needs to be further elucidated. Our HPLC analysis showed that 2 mM XPP treatment increased the ratio of ΔDi-0S within 3DOMMs. A relative increase in ΔDi-0S may regulate microenvironment and epithelial–stromal cell interactions in the 3DOMM, as indicated in various tissues.Citation49–51) In addition, the ratios of unsaturated disaccharide compositions derived from ChS and HS/Hep between 2 mM XPP treatment and a control group were similar to the previous study.Citation16) In contrast, this study showed a slight decrease in DS production, which is different from the previous report in which skin keratinocytes were cultured in a monolayer.Citation44) Thus, OFs, rather than OKs, appear to contribute to the current data using the OKs and OFs co-culture model.
Apparently, XPP treatment of the 3DOMMs upregulated the expression levels of major BMZ markers such as type IV collagen, laminin, integrin α6, and integrin β1. The data are consistent with a prior study showing an increase in the deposition of BMZ proteins induced by a different redox form of XPP, via stimulating GAGs/PGs synthesis.Citation18) Therefore, these findings also imply that XPP treatment induces the development of a well-formed epithelial layer because the basement membrane has a central role in epithelial homeostasis/regeneration.Citation21–25)
Our analyses also indicate the XPP-treated 3DOMMs expressed more decorin (DS/CSPG), a small leucine rich PG, and syndecan-1 (HSPG), a membrane PG, than the control, which was supported by an increase in the GAG synthesis by XPP supplementation. According to the previous studies, the reduction of decorin and syndecan-1 production in skin is closely related to aging process.Citation13,52,53) In addition, a relative increase in ΔDi-0S by XPP obtained from this study is also consistent with the previous report showing age-related differences in the catabolism of PGs in human skin.Citation54) Thus, these findings suggest an increase in the expression of decorin and syndecan-1 by the XPP treatment also plays a pivotal role in preventing the atrophic changes in the 3DOMMs. Furthermore, the expression level of CD44, another membrane PG serving as a major receptor of hyaluronate, is involved in both skin atrophy and hyperplasia.Citation55–57) In addition to the remarkable increase detected by the immunoblots analysis, CD44 was expressed more in the XPP-treated 3DOMM cell layers in the present study, consistent with the previous in vivo study.Citation19,20) Although XPP treatment did not increase the amount of HA in the culture supernatant and cell-associated GAG (data not shown), the upregulation of CD44 might also influence the quality of the epithelium.
We further assessed Akt/mTOR signaling activity because its downstream kinase and substrate are required for cell growth and increases in protein synthesis, and phosphorylation of those substrates may have an impact on the increase in epithelial thickness.Citation58) Immunoreaction of p-S6 suggested activation of the Akt/mTOR pathway in the upper suprabasal layers of the XPP-treated 3DOMM, which may also contribute to the consistent epithelial regeneration. However, in contrast to the 2 mM XPP treatment, the interquartile range of epithelial thickness by 10 mM XPP was larger. This may be due to slight decrease of mTOR activity detected by immunoblots. In addition, integrin α6 and CD44 expression may have a greater impact on the epithelial regeneration. However, this disparity needs to be clarified because the expression levels of type IV collagen, nidogen-2, decorin, and syndecan-1 were the highest among the three groups.
In summary, incubation with 2 or 10 mM XPP when manufacturing 3DOMMs led to an increase in mean epithelial thickness without affecting the epithelial structure or differentiation process, accompanied by an increase in the minimal epithelial thickness formed in 3DOMMs. Importantly, the 2 mM XPP treatment provided a smaller variation in the epithelial thickness, indicating a possible pharmacologic manipulation to improve the epithelial quality. The results may be due to the increase in the cell-associated GAGs as the expressions of decorin and syndecan-1 increased with the change of GAGs profiles, the upregulation of major BMZ markers expression in 3DOMM as well as the activation of the Akt/mTOR pathway. Although utilization of C-xylosides might improve the quality assurance/control of EVPOME fabrication by generating a consistent epithelial layer, further studies are necessary to develop a potent system for non-invasive detection of suboptimal EVPOME grafts.
Authors contributions
K. I. and A. U. designed the research plan with assistance from R.T. and T. M. A. U. and I. K. performed research and analyzed data. H. K. assisted the histologic examination and the statistical analysis. A. S., N. S., Y. H., T. S., and H. O. assisted assay experiments. A. U., I. K., and K. I. prepared manuscript with assistance from all authors. All authors revised and approved final manuscript.
Funding
This work was supported, in part, by the Regional Innovation Strategy Support Program (City Area Type) from the Ministry of Education, Culture, Sports, Science and Technology of Japan.
Acknowledgments
Authors would like to thank Drs. Masaru Kaku and Kazunari Higa for their technical assistance. I also thank the Oral Anatomy Department staff for their technical assistance.
Disclosure statement
No potential conflict of interest was reported by the authors.
Notes
Abbreviations: GAG, glycosaminoglycan; s-GAG, sulfated-GAG; PG, proteoglycan; ChS, chondroitin sulfate; Ch4S, chondroitin 4-sulfate; Ch6S, chondroitin 6-sulfate; Ch, chondroitin; DS, dermatan sulfate, Hep, heparin; HS, heparan sulfate; HA, hyaluronan; GlcUA, glucuronic acid; GalNAc, N-acetylgalactosamine; GlcNAc, N-acetylglucosamine; GlcN, glucosamine; TEOM, tissue-engineered oral mucosa; EVPOME, ex vivo produced oral mucosa equivalent; TE, tissue engineering; RM, regenerative medicine; BMZ, basement membrane zone; XPP, β-D-xylopyranoside-n-propane-2-one; 3DOMM, three-dimensional oral mucosa model; OK, oral keratinocyte; OF, oral fibroblast; HE, hematoxylin and eosin; ANOVA, analysis of variance; ECM, extracellular matrix.
References
- Izumi K, Song J, Feinberg SE. Development of a tissue-engineered human oral mucosa: from the bench to the bed side. Cells Tissues Organs. 2004;176:134–152.10.1159/000075034
- Ali RR, Hollander AP, Kemp P, et al. Regulating cell-based regenerative medicine: the challenges ahead. Regen. Med. 2014;9:81–87.10.2217/rme.13.78
- Izumi K, Neiva RF, Feinberg SE. Intraoral grafting of tissue-engineered human oral mucosa. Int. J. Oral Maxillofac. Implants. 2013;28:e295–e303.10.11607/jomi.te11
- Stroncek DF, Jin P, Wang E, et al. Potency analysis of cellular therapies: the emerging role of molecular assays. J. Transl. Med. 2007;5:24–33.10.1186/1479-5876-5-24
- Bieback K, Kinzebach S, Karagianni M. Translating research into clinical scale manufacturing of mesenchymal stromal cells. Stem Cells Int. 2010;2010:193519-193529.
- Kuo S, Zhou Y, Kim HM, et al. Biochemical indicators of implantation success of tissue-engineered. Oral Mucosa. J. Dent. Res. 2014;94:78–84.
- Izumi K, Inoki K, Fujimori Y, et al. Pharmacological retention of oral mucosa progenitor/stem cells. J. Dent. Res. 2009;88:1113–1118.10.1177/0022034509350559
- Andreescu CF, Mihai LL, Răescu M, et al. Age influence on periodontal tissues: a histological study. Rom. J. Morphol. Embryol. 2013;54:811–815.
- Abu Eid R., Sawair F, Landini G, et al. Age and the architecture of oral mucosa. Age (Dordr). 2012;34:651–658.10.1007/s11357-011-9261-1
- Mancini M, Lena AM, Saintigny G, et al. MicroRNAs in human skin ageing. Ageing Res. Rev. 2014;17:9–15.10.1016/j.arr.2014.04.003
- Farage MA, Miller KW, Elsner P, et al. Characteristics of the aging skin. Adv. Wound Care. 2013;2:5–10.10.1089/wound.2011.0356
- Oh JH, Kim YK, Jung JY, et al. Intrinsic aging- and photoaging-dependent level changes of glycosaminoglycans and their correlation with water content in human skin. J. Dermatol. Sci. 2011;62:192–201.10.1016/j.jdermsci.2011.02.007
- Oh JH, Kim YK, Jung JY, et al. Changes in glycosaminoglycans and related proteoglycans in intrinsically aged human skin in vivo. Exp. Dermatol. 2011;20:454–456.10.1111/exd.2011.20.issue-5
- Fritz TA, Esko JD. Xyloside priming of glycosaminoglycan biosynthesis and inhibition of proteoglycan assembly. Methods Mol. Biol. 2001;171:317–323.
- Compain P, Martin OR. Carbohydrate mimetics-based glycosyltransferase inhibitors. Bioorg. Med. Chem. 2001;9:3077–3092.10.1016/S0968-0896(01)00176-6
- Vassal-Stermann E, Duranton A, Black AF, et al. A new C-Xyloside induces modifications of GAG expression, structure and functional properties. PLoS ONE. 2012;7:e47933.10.1371/journal.pone.0047933
- Pineau N, Carrino DA, Caplan AI, et al. Biological evaluation of a new C-xylopyranoside derivative (C-Xyloside) and its role in glycosaminoglycan biosynthesis. Eur. J. Dermatol. 2011;21:359–370.
- Sok J, Pineau N, Dalko-Csiba M, et al. Improvement of the dermal epidermal junction in human reconstructed skin by a new c-xylopyranoside derivative. Eur. J. Dermatol. 2008;18:297–302.
- Pineau N, Bernerd F, Cavezza A, et al. A new C-xylopyranoside derivative induces skin expression of glycosaminoglycans and heparan sulphate proteoglycans. Eur. J. Dermatol. 2008;18:36–41.
- Deloche C, Minondo AM, Bernard BA, et al. Effect of C-xyloside on morphogenesis of the dermal epidermal junction in aged female skin. An ultrastuctural pilot study. Eur. J. Dermatol. 2011;21:191–196.
- Yurchenco PD. Basement membranes : cell scaffoldings and signaling platforms. Cold Spring Harb Perspect Biol. 2011;3:1–28.
- Breitkreutz D, Mirancea N, Nischt R. Basement membranes in skin: unique matrix structures with diverse functions? Histochem. Cell Biol. 2009;132:1–10.10.1007/s00418-009-0586-0
- Hartwell R, Lai A, Ghahary A. Modulation of extracellular matrix through keratinocyte—fibroblast crosstalk. Expert Rev. Dermatol. 2009;4:623–635.10.1586/edm.09.55
- El Ghalbzouri A, Ponec M. Diffusible factors released by fibroblasts support epidermal morphogenesis and deposition of basement membrane components. Wound Repair Regen. 2004;12:359–367.10.1111/wrr.2004.12.issue-3
- Segal N, Andriani F, Pfeiffer L, et al. The basement membrane microenvironment directs the normalization and survival of bioengineered human skin equivalents. Matrix Biol. 2008;27:163–170.10.1016/j.matbio.2007.09.002
- Shiomi A, Izumi K, Uenoyama A, et al. Cyclic mechanical pressure-loading alters epithelial homeostasis in a three-dimensional in vitro oral mucosa model: clinical implications for denture-wearers. J. Oral Rehabil. 2015;42:192–201.10.1111/joor.2015.42.issue-3
- Takagaki K, Iwafune M, Kakizaki I, et al. Cleavage of the xylosyl serine linkage between a core peptide and a glycosaminoglycan chain by cellulases. J. Biol. Chem. 2002;277:18397–18403.10.1074/jbc.M111985200
- Bitter T, Muir HM. A modified uronic acid carbazole reaction. Anal. Biochem. 1962;4:330–334.10.1016/0003-2697(62)90095-7
- Mathews MB. Acid strength of carboxyl groups in isomeric chondroitin sulfates. Biochim. Biophys. Acta. 1961;48:402–403.10.1016/0006-3002(61)90494-2
- Sugahara K, Okumura Y, Yamashina I. The engelbreth-holm-swarm mouse tumor produces undersulfated heparan sulfate and oversulfated galactosaminoglycans. Biochem. Biophys. Res. Commun. 1989;162:189–197.10.1016/0006-291X(89)91980-3
- Yoshida K, Miyauchi S, Kikuchi H, et al. Analysis of unsaturated disaccharides from glycosaminoglycuronan by high-performance liquid chromatography. Anal. Biochem. 1989;177:327–332.10.1016/0003-2697(89)90061-4
- Saito T, Izumi K, Shiomi A, et al. Zoledronic acid impairs re-epithelialization through down-regulation of integrin αvβ6 and transforming growth factor beta signalling in a three-dimensional in vitro wound healing model. Int. J. Oral Maxillofac. Surg. 2014;43:373–380.10.1016/j.ijom.2013.06.016
- Tanzer ML. Current concepts of extracellular matrix. J. Orthop. Sci. 2006;11:326–331.10.1007/s00776-006-1012-2
- Gallo RL. Proteoglycans and cutaneous vascular defense and repair. J. Investig. Dermatol. Symp. Proc. 2000;5:55–60.10.1046/j.1087-0024.2000.00008.x
- Vacanti CA, Kim W, Upton J, et al. Tissue-engineered growth of bone and cartilage. Transplant Proc. 1993;25:1019–1021.
- Ferdous Z, Grande-Allen KJ. Utility and control of proteoglycans in tissue engineering. Tissue Eng. 2007;13:1893–1904.10.1089/ten.2006.0056
- Kinikoglu B, Hemar J, Hasirci V, et al. Feasibility of a porcine oral mucosa equivalent: a preclinical study. Artif. Cells. Blood Substit. Immobil. Biotechnol. 2012;40:271–274.10.3109/10731199.2011.644293
- Kinikoglu B, Rovere MR, Haftek M, et al. Influence of the mesenchymal cell source on oral epithelial development. J. Tissue Eng. Regen. Med. 2012;6:245–252.10.1002/term.v6.3
- Wang T, Sun J, Wu H, et al. Evaluation and biological characterization of bilayer gelatin/chondroitin-6-sulphate/hyaluronic acid membrane. J. Biomed. Mater. Res. B. Appl. Biomater. 2007;82B:390–399.10.1002/(ISSN)1552-4981
- Kim SH, Turnbull J, Guimond S. Extracellular matrix and cell signalling: the dynamic cooperation of integrin, proteoglycan and growth factor receptor. J. Endocrinol. 2011;209:139–151.10.1530/JOE-10-0377
- Macri L, Silverstein D, Clark R. Growth factor binding to the pericellular matrix and its importance in tissue engineering☆. Adv. Drug Deliv. Rev. 2007;59:1366–1381.10.1016/j.addr.2007.08.015
- Rosso F, Giordano A, Barbarisi M, et al. From Cell-ECM interactions to tissue engineering. J. Cell. Physiol. 2004;199:174–180.10.1002/(ISSN)1097-4652
- Cavezza A, Boulle C, Guéguiniat A, et al. Synthesis of Pro-XylaneTM: a new biologically active C-glycoside in aqueous media. Bioorg. Med. Chem. Lett. 2009;19:845–849.10.1016/j.bmcl.2008.12.037
- Muto J, Naidu NN, Yamasaki K, et al. Exogenous addition of a C-xylopyranoside derivative stimulates keratinocyte dermatan sulfate synthesis and promotes migration. PLoS ONE. 2011;6:e25480.10.1371/journal.pone.0025480
- Andriani F, Margulis A, Lin N, et al. Analysis of microenvironmental factors contributing to basement membrane assembly and normalized epidermal phenotype. J. Invest. Dermatol. 2003;120:923–931.10.1046/j.1523-1747.2003.12235.x
- Varkey M, Ding J, Tredget EE. Superficial dermal fibroblasts enhance basement membrane and epidermal barrier formation in tissue-engineered skin: implications for treatment of skin basement membrane disorders. Tissue Eng. Part A. 2014;20:540–552.
- Beard JD, Guy RH, Gordeev SN. Mechanical tomography of human corneocytes with a nanoneedle. J. Invest. Dermatol. 2013;133:1565–1571.10.1038/jid.2012.465
- Conneely MJ, Campbell PA. What lies beneath? Scanning probe tomography may have the answer. J. Invest. Dermatol. 2013;133:1458–1460.10.1038/jid.2013.30
- Sorrell JM, Carrino DA, Baber MA, et al. Versican in human fetal skin development. Anat. Embryol(Berl). 1999;199:45–56.10.1007/s004290050208
- Zhang F, Zhang Z, Thistle R, et al. Structural characterization of glycosaminoglycans from zebrafish in different ages. Glycoconj. J. 2009;26:211–218.10.1007/s10719-008-9177-x
- Uchisawa H, Okuzaki B, Ichita J, et al. Binding between calcium ions and chondroitin sulfate chains of salmon nasal cartilage glycosaminoglycan. Int. Congre. Ser. 2001;1223:205–220.10.1016/S0531-5131(01)00458-7
- Li Y, Liu Y, Xia W, et al. Age-dependent alterations of decorin glycosaminoglycans in human skin. Sci. Rep. 2013;3:2422–2429.
- Reed CC, Iozzo RV. The role of decorin in collagen fibrillogenesis and skin homeostasis. Glycoconj. J. 2003;19:249–255.
- Carrino DA, Calabro AD, Aniq B, et al. Age-related differences in human skin proteoglycans. 2011;21:257–268.
- Kaya G, Tran C, Sorg O, et al. Hyaluronate fragments reverse skin atrophy by a CD44-dependent mechanism. PLoS Med. 2006;3:e493.10.1371/journal.pmed.0030493
- Gu H, Huang L, Wong YP, et al. HA modulation of epidermal morphogenesis in an organotypic keratinocyte-fibroblast co-cultured model. Exp Dermatol. 2010;19:e336–e339.
- Kaya G, Saurat JH. Dermatoporosis: a new concept in skin aging. Eur. Geriatr. Med. 2010;1:216–219.10.1016/j.eurger.2010.06.002
- Clark R, Pavlis M. Dysregulation of the mTOR pathway secondary to mutations or a hostile microenvironment contributes to cancer and poor wound healing. J. Invest. Dermatol. 2009;129:529–531.10.1038/jid.2008.441