Abstract
Bone homeostasis is maintained by balancing bone formation and bone resorption, but an imbalance between them is associated with various bone-related diseases such as osteoporosis and rheumatoid arthritis. We found that 5,6-dehydrokawain (DK) and dihydro-5,6-dehydrokawain (DDK), which were isolated as promising compounds from Alpinia zerumbet rhizomes, promote differentiation of osteoblastic MC3T3-E1 cells. DK and DDK increased the alkaline phosphatase activity and matrix mineralization of MC3T3-E1 cells. DK exerts larger effects than DDK. The gene expression of runt-related transcription factor 2 and osterix, which are essential transcription factors in the early period of osteoblast differentiation, was significantly increased by DK treatment. The mRNA level of distal-less homeobox 5 was also enhanced by DK treatment, and DK activated the p38 mitogen-activated protein kinase pathway. Therefore, DK may have clinical potential for preventing osteoporosis, and could be considered as a potential anabolic therapeutic agent.
Graphical abstract
5,6-Dehydrokawain (DK) and dihydro-5,6-dehydrokawain (DDK) from Alpinia zerumbet rhizomes, promote differentiation of osteoblastic MC3T3-E1 cells.
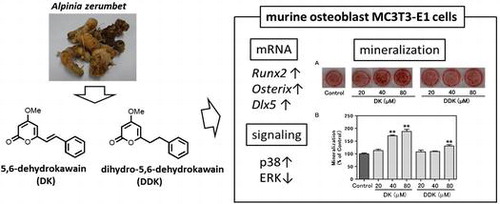
Osteoporosis, characterized by a systemic impairment of bone mass and strength that leads to fragility fractures is a growing medical and socioeconomic issue. Bone formation by osteoblasts and resorption by osteoclasts are tightly coordinated during the remodeling cycle. The loss of bone mass results from an imbalance of these fundamental processes.Citation1,2)
Recent therapies for osteoporosis have mainly focused on the inhibition of bone resorption by osteoclasts.Citation2) For example, bisphosphonates and selective estrogen receptor modulators have been used clinically to prevent bone loss.Citation1,2) However, many osteoporotic patients have already lost a substantial amount of bone. Therefore, it is desirable to develop anabolic treatments that enhance osteoblastic activity.Citation3) Some chemical factors in food and plants have been reported to stimulate osteoblasts.Citation4–7) Beneficial effects against bone loss of isoflavones, such as daidzein and genistein, which are abundant in soybeans have been extensively investigated in various experimental models and in clinical studies.Citation8–10) Several plant-derived isoflavones and the synthetic analogs stimulate osteogenesis and might be potential anabolic agents for the enhancement of bone health.Citation11–13)
Alpinia zerumbet (A.zerumbet), a species of the family zingiberaceae, is abundant in the subtropical and tropical regions. A. zerumbet is known for its human use as a traditional herbal medicine, food,Citation14,15) and dietary supplement especially in Okinawa. Previous reports have shown the α-pyrones, 5,6-dehydrokawain (DK), and dihydro-5,6-dehydrokawain (DDK) are major components in Alpinia leaves and rhizomes.Citation14) These compounds have been shown to have antioxidant properties and to inhibit enzymes that cause skin diseases.Citation16) They also inhibit HIV-1 integrase and neuraminidase activities,Citation17) and prevent the accumulation of advanced glycation end products (AGEs),Citation18) among other beneficial bioactivities.Citation19–21) In the present work, we studied the potential use of DK and DDK as a treatment for osteoporosis by investigating their role in modulating alkaline phosphatase (ALP) activity, mineralization, and osteoblastic gene expression using the osteoblastic cell line, MC3T3-E1. Furthermore, we measured their effect on tartrate-resistant acid phosphatase (TRAP) activity in osteoclast precursor cell line RAW264 cells after stimulation by receptor activator of the nuclear factor kappa B ligand (RANKL).
Materials and methods
Reagents
Alpha-minimum essential medium eagle (α-MEM) and penicillin–streptomycin were purchased from Sigma-Aldrich Japan (Tokyo, Japan). Fetal bovine serum (FBS) and GlutaMAX™ were purchased from Life Technologies Japan (Tokyo, Japan). Ascorbic acid and β-glycerophosphate were purchased from Wako Pure Chemical (Osaka, Japan). Antibodies to p38 mitogen-activated protein kinase (MAPK), phospho-p38 MAPK (p-p38), p44/42 MAPK (ERK1/2), phospho-p44/42 MAPK (Thr202/Tyr204; p-ERK1/2), stress-activated protein kinase/jun-amino-terminal kinase (SPAK/JNK; JNK), and phospho-JNK (p-JNK) were obtained from Cell Signaling Technology (Beverly, MA, USA). Recombinant soluble mouse RANKL was from R&D Systems (Minneapolis, USA). All other reagents used were of analytical grade.
Cell culture and treatment
MC3T3-E1 and RAW264 murine cell lines were obtained from the RIKEN Cell Bank (Tsukuba, Japan). These cells were cultured in α-MEM supplemented with 10% FBS, 1% GlutaMAX™, 100 units/mL of penicillin, and 100 μg/mL of streptomycin (Culture medium). To differentiate osteoblasts, differentiation medium (DM) supplemented with 50 μg/mL ascorbic acid and 10 mM β-glycerophosphate in culture medium was used. DK and DDK were dissolved in dimethyl sulfoxide (DMSO). The DMSO solution was diluted with DM to achieve the desired final concentration of 0–80 μM DK and DDK. The final concentration of DMSO in the DM was less than 0.1% (v/v) and did not affect cell viability. During incubation, the medium was changed every two or three days. The cultures were maintained in a humidified atmosphere of 5% CO2 at 37 °C.
Isolation of compounds
Rhizomes of A. zerumbet were collected from the University of the Ryukyus campus, Okinawa, Japan. Samples (1.50 kg) were cut, dried for one day, and extracted 3 times with 3 L of MeOH for one day. The extract was filtered and evaporated under vacuum at 40 °C. MeOH extract (MeOH ext., 21.30 g) was obtained and partitioned with EtOAc (500 mL × 3) and water. The EtOAc layer was combined and evaporated to residue (EtOAc ext., 4.70 g). The remaining water layer was further extracted with BuOH (300 mL × 3). The BuOH layer was combined and evaporated (BuOH ext., 1.64 g). The resultant water layer was evaporated (Water ext., 12.77 g). A part of the EtOAc ext. was purified by silica gel (Wako) column chromatography and finally isolated with preparative high performance liquid chromatography (HPLC) using a C18 column (Wakosil-II 5C18 RS-Prep, 5 μm, 20.0 × 250 mm; Wako). Two main peaks were separated (Fig. (A)) and the corresponding compounds were identified using nuclear magnetic resonance (NMR) and high-resolution electrospray ionization mass spectrometry (HRESIMS).Citation17) DK (4.4 mg) and DDK (5.5 mg) were isolated from 100.5 mg of EtOAc ext,. respectively. NMR spectra were recorded on a JEOL JNM-ECZ400S in CDCl3. HRMS was recorded on a Thermo scientific Q Exactive™ instrument. DK and DDK were characterized by the following data.
5,6-Dehydrokawain (DK)
1H NMR (400 MHz): δ 3.83 (s, 3H), 5.50 (d, J = 2.0 Hz, 1H), 5.95 (d, J = 2.4 Hz, 1H), 6.59 (d, J = 16.0 Hz, 1H), 7.35–7.52 (m, 6H); 13C NMR (400 MHz): δ 55.9, 88.8, 101.3, 118.6, 127.4, 128.9, 129.4, 135.2, 135.8, 158.6, 164.0, 171.1; HRESIMS calculated for C14H13O3 229.0865; found [M + H] + 229.0866.
Dihydro-5,6-dehydrokawain (DDK)
1H NMR (400 MHz): δ 2.75 (t, J = 8.0 Hz, 2H), 2.97 (t, J = 7.8 Hz, 2H), 3.77 (s, 3H), 5.41 (s, 1H), 5.72 (s, 1H), 7.16–7.31 (m, 5H); 13C NMR (400 MHz): δ 32.8, 35.4, 55.8, 87.7, 100.2, 126.4, 128.3, 128.6, 139.8, 164.3, 164.9, 171.1; HRESIMS calculated for C14H15O3 231.1021; found [M + H] + 231.1024.
ALP activity and cell proliferation assay of MC3T3-E1 cells
Pre-osteoblastic MC3T3-E1 cells were seeded at a density of 5.0 × 104 cells/well in a 24-well plate and incubated for seven days (pre-culture period). Then the medium was changed to DM with DMSO (control) or sample solution and incubated for an additional seven days. For assessing the ALP activity, the cells were collected, washed with saline, and treated with lysis buffer (0.1 M Tris-HCl pH 7.5 containing 0.1% Triton-X 100). ALP activity in the cell lysate was evaluated using a TRACP and ALP assay kit (Takara Bio, Shiga, Japan) according to the manufacturer’s protocol. Cell proliferation was evaluated by a WST-8 (Dojindo, Kumamoto, Japan) reagent. WST-8 solution was added to each well, and the plate was incubated for 0.5 h. The absorbance of each well was recorded with a microplate reader at 450 nm.
Alizarin red staining
MC3T3-E1 cells were seeded at a density of 5.0 × 104 cells/well in a 24-well plate and incubated for seven days (pre-culture period). Then the medium was changed to DM with DMSO (control) or sample solution and incubated for an additional 13 days. After that cells were fixed with ice-cold 70% ethanol and stained with alizarin red S (Wako) solution. For quantification, cells stained with alizarin red S were solubilized with cetylpyridinium chloride, then the extracted stain was transferred to 96-well plates, and the absorbance at 550 nm was measured using a microplate reader.
Gene expression analysis of MC3T3-E1 cells
MC3T3-E1 cells were seeded at a density of 5.0 × 104 cells/well in a 24-well plate and incubated for seven days (pre-culture period). Then the medium was changed to DM with DMSO (control) or sample solution and incubated for an additional two days. Then, total RNA was extracted from the cells using an RNeasy Mini Kit (Qiagen, Tokyo, Japan). cDNAs were synthesized using random primers and PrimeScript™ Reverse Transcriptase (Takara Bio). The target cDNAs were amplified using Fast SYBR® Green Master Mix (Life Technologies, Japan) together with gene-specific primers (Takara Bio). The primer sequences are listed in Table . PCR conditions were 95 °C for 20 s, 95 °C for 3 s, and 60 °C for 30 s for 40 cycles. PCR products were measured using a StepOnePlus™ Real time PCR System (Life Technologies, Japan). The fold change expression analysis was performed using a comparative Cq method (2-delta-delta Cq). Actb was used as an internal control.
Table 1. Primer pairs used for RT-PCR.
Western blot analysis
MC3T3-E1 cells were seeded at a density of 10.0 × 104 cells/well in a 12-well plate and incubated for seven days (pre-culture period). Then the medium was changed to DM with DMSO (control) or 80 μM DK and incubated for an additional 0, 5, 15, and 30 min. Cells were washed twice with PBS and lysed using cOmplete™ Lysis-M and Phos-STOP™ (Roche Diagnostic, Tokyo, Japan). Lysates were centrifuged at 13,000 rpm for 5 min and supernatants were collected. The protein content was measured with a Pierce BCA Protein Assay Kit (Thermo Scientific, Rockford, USA). Each whole cell lysate was suspended in a 2×Laemmli Sample Buffer (Bio-Rad, Hercules, USA) containing 2% 2-mercaptoethanol and boiled at 100 °C for 5 min. Equal amounts of protein (5 μg) were separated by SDS-PAGE and transferred to a PVDF membrane (Merck Millipore, MA, USA). After blocking for 1 h in 2% ECL Blocking reagent (GE Healthcare, Tokyo, Japan) in TPBS (PBS and 0.1% Tween 20), the membrane was incubated overnight at 4 °C with various primary antibodies. The membrane was then washed four times with TPBS and incubated at room temperature with the secondary antibody (GE Healthcare). After washing four times with TPBS, protein bands were detected with ECL Prime Western Blotting Detection Reagents (GE Healthcare) and imaged on a LAS-4000 Luminescent Image Analyzer (GE Healthcare). Band intensities were measured for statistical analysis using Multi Gauge, version 3.11, software (Fujifilm Life Science, Tokyo, Japan).
Tartrate-resistant acid phosphatase (TRAP) activity assay and cell proliferation assay of RAW264 cells
RAW264 cells (2.5 × 104 cells/well) were cultured in 24-well plates and incubated for 24 h (pre-culture period). Then, the medium was changed to the culture medium containing 0–40 μM DK or DDK and 10 ng/ml RANKL for an additional 48 h. Then, the cells were collected, washed with PBS, and treated with lysis buffer (0.1 M Tris-HCl pH 7.5 containing 0.1% Triton-X 100). TRAP activity in the cell lysate was measured using a TRACP and ALP assay kit (Takara Bio). Cell proliferation was evaluated by a WST-8 (Dojindo) reagent. WST-8 solution was added to each well, and the plate was incubated for 0.5 h. The absorbance of each well was recorded with a microplate reader at 450 nm.
Statistical analysis
All data are expressed as mean ± standard error of the mean (SEM). Comparisons between control and each group were analyzed using one-way analysis of variance followed by Dunnett’s multiple comparison test or Student’s t test using GraphPad Prism V5.02 software for Windows (Graphpad Software, San Diego, California, USA). P-values < 0.05 were considered significant.
Results
Effect of DK and DDK on ALP activity and cell proliferation in MC3T3-E1 cells
In the ALP activity assay, the DM significantly increased the ALP activity compared to culture medium-treated cells (data not shown). DK at 80 μM enhanced the ALP activity by 25% in comparison to the control cells. DDK at 80 μM also enhanced ALP activity by approximately 8% (Fig. (A)). 40 μM DK and DDK did not significantly enhance the ALP activity.
Fig. 2. Effect of DK and DDK on ALP activity and cell proliferation in MC3T3-E1 cells.
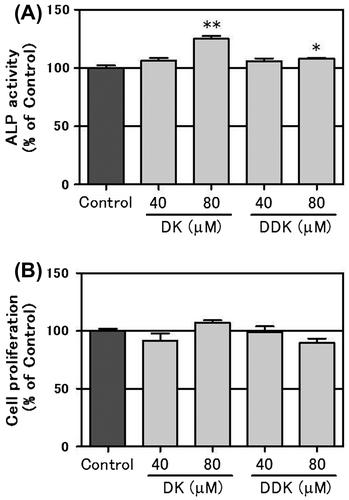
The effect of DK and DDK on cell proliferation was determined by WST-8 assay. Under the test conditions, DK and DDK did not affect cell proliferation at any of the concentrations used (Fig. (B)).
Effect of DK and DDK on matrix mineralization of MC3T3-E1 cells
The effect of DK and DDK on bone matrix mineralization was determined by alizarin red S staining. DK significantly enhanced bone nodule formation in a concentration-dependent manner (Fig. (A) and (B)). Quantification analysis shows DK at 80 and 40 μM increased mineralization by approximately 88 and 71%, respectively, in comparison to control. DDK at 80 μM also significantly augmented matrix mineralization of MC3T3-E1 cells by about 31% in comparison to the control cells.
Fig. 3. Effect of DK and DDK on matrix mineralization of MC3T3-E1 cells.
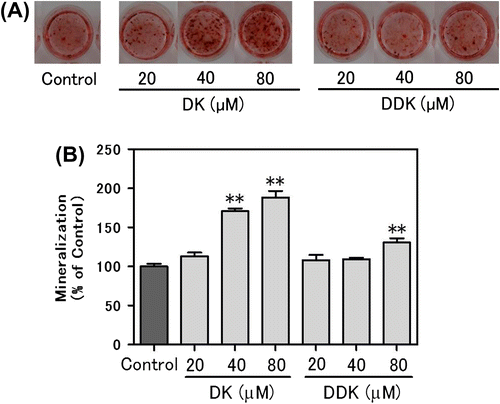
Effect of DK on osteoblastic gene expression
The osteogenic mRNA expression levels of three transcription factors [runt-related transcription factor 2 (Runx2), Osterix (Osx), and distal-less homeobox 5 (Dlx5)], and two differentiation markers [alkaline phosphatase (Alpl) and osteocalcin (Bglap)] in the MC3T3-E1 cells treated with DK for two days were compared with control cells (Fig. ). The Runx2 mRNA expression level was increased by approximately 60% in comparison to the control cells at 80 μM by DK treatment. 40 μM DK treatment also enhanced Runx2 expression level by 29%. The Osx mRNA expression was increased dose dependently by about 141 and 62% in comparison to the control by adding 80 or 40 μM DK, respectively. Dlx5 mRNA also increased by about 48 and 42% of the levels in the control cells by adding 80 or 40 μM DK, respectively. mRNA levels of Alpl and Bglap also were dose dependently increased by DK treatment.
Fig. 4. Effect of DK on osteoblast-related genes in MC3T3-E1 cells.
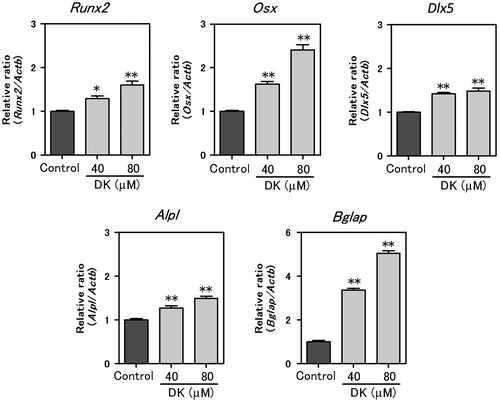
Effect of DK on MAPKs phosphorylation in MC3T3-E1 cells
Using western blot analysis, phosphorylation of p38, ERK1/2, and JNK was elevated by stimulation of the DM. DK additively enhanced phosphorylation of p38 and suppressed phosphorylation of ERK1/2. There was no significant effect on JNK activation (Fig. ).
Effect of DK and DDK on RANKL-induced osteoclast activity in RAW 264 cells
The effect of DK and DDK on differentiation of RAW264 cells to osteoclasts was investigated. TRAP activity was elevated significantly by RANKL stimulation (Fig. ). DK and DDK did not affect TRAP activity of RANKL-stimulated RAW 264 cells. Cell proliferation was significantly decreased by DK and DDK at 40 μM (Fig. ).
Fig. 6. Effect of DK and DDK on osteoclastogenesis.
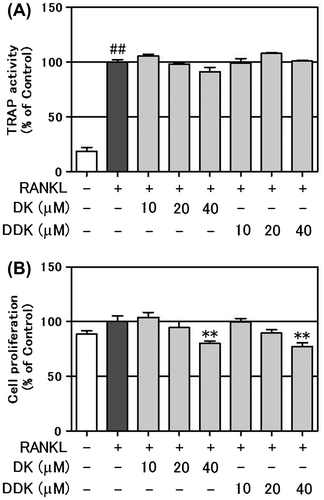
Discussion
ALP activity is one of the most frequently used biochemical markers of early osteoblastic differentiation.Citation22,23) We first examined the effects of each extract of A.zerumbet rhizomes on ALP activity. The results showed EtOAc ext. increased ALP activity by 30% at a concentration of 50 μg/ml, but BuOH ext. and Water ext. did not exhibit any increased ALP activity (data not shown). HPLC analysis revealed there were two main peaks in the EtOAc ext. (Fig. (A)), and these two peaks were identified as DK and DDK. So we concluded that DK and DDK were the main active components of the A.zerumbet rhizomes that promoted osteoblast differentiation. DK and DDK significantly increased ALP activity of the MC3T3-E1 cells, but cell proliferation was not affected by their treatment (Fig. ). Compared with DK and DDK, DK displayed greater effects than DDK on ALP activity.
Extracellular matrix mineralization occurs in the later period of osteoblast differentiation.Citation23) Therefore, we investigated whether DK or DDK treatment of MC3T3-E1 cells increased the deposition of mineralized matrix. Extracellular calcium deposits in mineralizing nodules were stained with alizarin red S. Mineralization was observed in the matrix of cells treated with DK and DDK after 13 days of differentiation (Fig. (A)). DK and DDK therefore had an acceleration effect on matrix mineralization by the cells. Interestingly, quantification revealed that DK had a greater effect on accelerating mineralization than did DDK (Fig. (B)).
In past studies, DK and DDK showed different effects in response to various biological assays. For example, DK and DDK demonstrate the same level of inhibition against HIV-1 integrase and neuraminidase,Citation17) but DK showed greater anti-oxidative activity than DDK and inhibited collagenase, elastase, hyaluronidase, and tyrosinase to a greater extent than DDK.Citation16) DK also demonstrated stronger anti-AGEs activity than DDK.Citation18) On the other hand, DDK exhibited significantly greater inhibition than DK on pancreatic lipase activity.Citation19) The difference in structure between DK and DDK is the presence of a double bond in the linker part of α-pyrone and the phenyl groups (Fig. (B)). In the recent years, it has been reported that covalent binding of the α,β-unsaturated carbonyl group in phytochemicals to various proteins via the Michael addition are important to the molecule’s bioactivity.Citation24–26) The extension of the conjugated double bond from the carbonyl group in DK may stimulate osteoblasts.
An additional goal was to reveal the molecular mechanisms by which DK causes the potent enhancement of mineralization. Runx2 is an essential transcription factor that controls the expression of genes related to osteogenic differentiation.Citation27) Osterix also is essential for osteoblast differentiation and bone formation.Citation28) These two transcription factors govern the critical regulation of osteoblast differentiation.Citation29) DK treatment significantly increased Runx2 and Osx expression in MC3T3-E1 cells (Fig. ). We conclude it is involved in the DK-induced osteoblast stimulation. Alpl and Bglap are osteoblastic marker genes whose expression is regulated by Runx2 and Osterix.Citation30) Alpl and Bglap were significantly enhanced by DK treatment as well (Fig. ). These results show that DK treatment of MC3T3-E1 cells enhances the transcription factors Runx2 and Osterix, and induces the subsequent expression of Alpl and Bglap.
In this study, the DM induced activation of MAPKs (Fig. ). The MAPK signaling pathway plays an important role during osteoblast differentiation,Citation31) and especially, activation of p38 MAPK is essential for osteoblast differentiation.Citation32,33) Some recent studies show that plant-derived compounds or extracts enhance osteoblast differentiation through p38 MAPK activation.Citation11,34) Ulsamer et al. reported bone morphological protein-2-induced activation of Osterix through upregulation of Dlx5 and p38 MAPK activation.Citation35) Kawane et al. reported Dlx5 directly binds to the 343-bp enhancer and plays an important role in directing Runx2 expression.Citation36) In the DK treatment of MC3T3-E1 cells, mRNA levels of Dlx5, Osx, and Runx2 were increased (Fig. ) and phosphorylation of p38 MAPK also was elevated significantly (Fig. ). From these results, we speculated that DK-induced p38 MAPK activation is involved in the elevation of Dlx5, Osx, and Runx2. However, further studies are needed to clarify these molecular mechanisms.
Kono et al. showed that the ERK pathway was involved in negative regulation of skeletal mineralization.Citation37) The phosphorylation of ERK protein treated with DK was significantly decreased (Fig. ). ERK suppression may allow matrix mineralization by DK treatment.
The importance of the JNK cascade for osteoblast differentiation also has been reported.Citation38) We examined JNK activation by DK treatment, but did not observe a significant change (Fig. ).
Osteoclasts resorb bone, and skeletal disease is induced through excess osteoclastic activity.Citation39) To determine the effect of DK and DDK on bone resorption, RAW264 cells were stimulated with RANKL, and TRAP activity was assessed. DK and DDK did not decrease TRAP activity in RANKL-stimulated RAW 264 cells at the tested concentrations, but cell proliferation was suppressed at a concentration of 40 μM (Fig. ). This result indicates that DK and DDK do not affect osteoclast differentiation in vitro.
In this study, we revealed the osteoblast stimulating activity of DK and DDK isolated from rhizomes of A. zerumbet. DK exerted greater effects on ALP activity and mineralization of MC3T3-E1 cells than DDK. Therefore, DK may have clinical potential for treating osteoporosis, and could be considered as a potential anabolic therapeutic agent. However, further research is necessary to define in more detail the mechanisms of action of DK, and pre-clinical studies will be required to evaluate its effects in vivo.
Authors contribution
M.K., T.M., A.W., I.Y., K.F., M.W., and S.T. conceived and designed the experiments. M.K., T.M., A.W., and T.H. performed the experiments. M.K., K.N., and Y.M. wrote the manuscript. All authors discussed the results and commented on the manuscript.
Acknowledgment
The authors would like to thank Dr. Yoshimitsu Tachi at the Osaka City University and Ms. Keiko Koizumi and Mr. Masahiro Ide at the Japan Food Research Laboratories for their useful comments and suggestions. The authors also thank Dr. Takahiro Ishii and Mr. Nozomi Taira at the University of the Ryukyus for collection of A. zerumbet.
Disclosure statement
No potential conflict of interest was reported by the authors.
References
- Rachner TD, Khosla S, Hofbauer LC. Osteoporosis: now and the future. Lancet. 2011;377:1276–1287.10.1016/S0140-6736(10)62349-5
- Rodan GA, Martin TJ. Therapeutic approaches to bone diseases. Science. 2000;289:1508–1514.10.1126/science.289.5484.1508
- Mohan S, Kutilek S, Zhang C, et al. Comparison of bone formation responses to parathyroid hormone(1-34), (1-31), and (2-34) in mice. Bone. 2000;27:471–478.10.1016/S8756-3282(00)00355-0
- Kono R, Okuno Y, Inada K, et al. A Prunu mume extract stimulated the proliferation and differentiation of osteoblastic MC3T3-E1 cells. Biosci. Biotechnol. Biochem. 2011;75:1907–1911.10.1271/bbb.110264
- Narita Y, Nomura J, Ohta S, et al. Royal jelly stimulates bone formation: physiologic and nutrigenomic studies with mice and cell lines. Biosci. Biotechnol. Biochem. 2006;70:2508–2514.10.1271/bbb.60240
- Ji MY, Leem KH, Kim M, et al. Egg yolk protein stimulates the proliferation and differentiation of osteoblastic MC3T3-E1 cells. Biosci. Biotechnol. Biochem. 2007;71:1327–1329.10.1271/bbb.60217
- Yamaguchi M. β-Cryptoxanthin and bone metabolism: the preventive role in osteoporosis. J. Health. Sci. 2008;54:356–369.10.1248/jhs.54.356
- Sugimoto E, Yamaguchi M. Stimulatory effect of daidzein in osteoblastic MC3T3-E1 cells. Biochem. Pharmacol. 2000;59:471–475.10.1016/S0006-2952(99)00351-2
- Yamaguchi M, Weitzmann MN. The estrogen 17β-estradiol and phytoestrogen genistein mediate differential effects on osteoblastic NF-κB activity. Int. J. Mol. Med. 2009;23:297–301.
- Sirotkin AV, Harrath AH. Phytoestrogens and their effects. Eur. J. Pharmacol. 2014;741:230–236.10.1016/j.ejphar.2014.07.057
- Don MJ, Lin LC, Chiou WF. Neobavaisoflavone stimulates osteogenesis via p38-mediated up-regulation of transcription factors and osteoid genes expression in MC3T3-E1 cells. Phytomedicine. 2012;19:551–561.10.1016/j.phymed.2012.01.006
- Wang W, Olson D, Cheng B, et al. Sanguis Draconis resin stimulates osteoblast alkaline phosphatase activity and mineralization in MC3T3-E1 cells. J. Ethnopharmacol. 2012;142:168–174.10.1016/j.jep.2012.04.033
- Strong AL, Jiang Q, Zhang Q, et al. Design, synthesis, and osteogenic activity of daidzein analogs on human mesenchymal stem cells. ACS Med. Chem. Lett. 2014;5:143–148.10.1021/ml400397k
- Xuan TD, Teschke R. Dihydro-5,6-dehydrokawain (DDK) from Alpinia zerumbet: its isolation, synthesis, and characterization. Molecules. 2015;20:16306–16319.10.3390/molecules200916306
- Upadhyay A, Chompoo J, Taira N, et al. Significant longevity-extending effects of Alpinia zerumbet leaf extract on the life span of Caenorhabditis elegans. Biosci. Biotechnol. Biochem. 2013;77:217–223.10.1271/bbb.120351
- Chompoo J, Upadhyay A, Fukuta M, et al. Effect of Alpinia zerumbet components on antioxidant and skin diseases-related enzymes. BMC Comp. Alt. Med. 2012;12:106.10.1186/1472-6882-12-106
- Upadhyay A, Chompoo J, Kishimoto W, et al. HIV-1 integrase and neuraminidase inhibitors from Alpinia zerumbet. J. Agric. Food. Chem. 2011;59:2857–2862.10.1021/jf104813k
- Chompoo J, Upadhyay A, Kishimoto W, et al. Advanced glycation end products inhibitors from Alpinia zerumbet rhizomes. Food Chem. 2011;129:709–715.10.1016/j.foodchem.2011.04.034
- Be Tu PT, Tawata S. Anti-Obesity effects of hispidin and Alpinia zerumbet Bioactives in 3T3-L1 Adipocytes. Molecules. 2014;19:16656–16671.
- Be Tu PT, Chompoo J, Tawata S. Hispidin and related herbal compounds from Alpinia zerumbet inhibit both PAK1-dependent melanogenesis in melanocytes and reactive oxygen species (ROS) production in adipocytes. Drug. Discov. Ther. 2015;9:197–204.10.5582/ddt.2015.01038
- Nguyen BCQ, Taira N, Tawata S. Several herbal compounds in Okinawa plants directly inhibit the oncogenic/aging kinase PAK1. Drug. Discov. Ther. 2014;8:238–244.10.5582/ddt.2014.01045
- Millán JL. The role of phosphatases in the initiation of skeletal mineralization. Calcif. Tissue. Int. 2013;93:299–306.10.1007/s00223-012-9672-8
- Owen TA, Holthuis J, Markose E, et al. Modifications of protein-DNA interactions in the proximal promoter of a cell-growth-regulated histone gene during onset and progression of osteoblast differentiation. Proc. Natl. Acad. Sci. USA. 1990;87:5129–5133.10.1073/pnas.87.13.5129
- Ohnishi K, Ohkura S, Nakahata E, et al. Non-specific protein modifications by a phytochemical induce heat shock response for self-defense. PLoS One. 2013;8:e58641.10.1371/journal.pone.0058641
- Antony ML, Lee J, Hahm ER, et al. Growth arrest by the antitumor steroidal lactone withaferin A in human breast cancer cells is associated with down-regulation and covalent binding at cysteine 303 of β-tubulin. J. Biol. Chem. 2014;289:1852–1865.10.1074/jbc.M113.496844
- Avonto C, Taglialatela-Scafati O, Pollastro F, et al. An NMR spectroscopic method to identify and classify thiol-trapping agents: revival of michael acceptors for drug discovery? Angew. Chem. Int. Ed. 2011;50:467–471.10.1002/anie.201005959
- Komori T, Yagi H, Nomura S, et al. Target disruption of Cbfa1 results in a complete lack of bone formation owing to maturational arrest of osteoblasts. Cell. 1997;89:755–764.10.1016/S0092-8674(00)80258-5
- Nakashima K, Zhou X, Kunkel G, et al. The novel zinc finger-containing transcription factor osterix is required for osteoblast differentiation and bone formation. Cell. 2002;108:17–29.10.1016/S0092-8674(01)00622-5
- Komori T. Signaling networks in RUNX2-dependent bone development. J. Cell. Biochem. 2011;112:750–755.10.1002/jcb.22994
- Hughes FJ, Turner W, Belibasakis G, et al. Effects of growth factors and cytokines on osteoblast differentiation. Periodontology. 2000;2006:48–72.
- Jadlowiec J, Koch H, Zhang X, et al. Phosphophoryn regulates the gene expression and differentiation of NIH3T3, MC3T3-E1, and human mesenchymal stem cells via the integrin/MAPK signaling pathway. J. Biol. Chem. 2004;279:53323–53330.10.1074/jbc.M404934200
- Suzuki A, Guicheux J, Palmer G, et al. Evidence for a role of p38 MAP kinase in expression of alkaline phosphatase during osteoblastic cell differentiation. Bone. 2002;30:91–98.10.1016/S8756-3282(01)00660-3
- Hu Y, Chan E, Wang SX, et al. Activation of p38 mitogen-activated protein kinase is required for osteoblast differentiation. Endocrinology. 2003;144:2068–2074.10.1210/en.2002-220863
- Itoh T, Nakamura M, Nakamichi H, et al. Regulation of the differentiation of osteoblasts and osteoclasts by hot-water extract of adzuki beans (Vigna angularis). Biosci. Biotech. Biochem. 2014;78:92–99.10.1080/09168451.2014.877182
- Ulsamer A, Ortuno MJ, Ruiz S, et al. BMP-2 induces osterix expression through up-regulation of Dlx5 and its phosphorylation by p38. J. Biol. Chem. 2008;283:3816–3826.10.1074/jbc.M704724200
- Kawane T, Komori H, Liu W, et al. Dlx5 and Mef2 regulate a novel Runx2 enhancer for osteoblast-specific expression. J. Bone. Miner. Res. 2014;29:1960–1969.10.1002/jbmr.v29.9
- Kono S, Oshima Y, Hoshi K, et al. Erk pathways negatively regulate matrix mineralization. Bone. 2007;40:68–74.10.1016/j.bone.2006.07.024
- Matsuguchi T, Chiba N, Bandow K, et al. JNK activity is essential for Atf4 expression and late-stage osteoblast differentiation. J. Bone. Miner. Res. 2009;24:398–410.10.1359/jbmr.081107
- Boyle WJ, Simonet WS, Lacey DL. Osteoclast differentiation and activation. Nature. 2003;423:337–342.10.1038/nature01658