Abstract
Naringin (Nar) has antioxidant and anti-inflammatory properties. It was recently reported that enzymatic modification of Nar enhanced its functions. Here, we acylated Nar with fatty acids of different sizes (C2–C18) using immobilized lipase from Rhizomucor miehei and investigated the anti-inflammatory effects of these molecules. Treatment of murine macrophage RAW264.7 cells with Nar alkyl esters inhibited lipopolysaccharide (LPS)-induced nitric oxide (NO) production, with Nar lauroyl ester (Nar-C12) showing the strongest effect. Furthermore, Nar-C12 suppressed the LPS-induced expression of inducible NO synthase by blocking the phosphorylation of inhibitor of nuclear factor (NF)-κB-α as well as the nuclear translocation of NF-κB subunit p65 in macrophage cells. Analysis of Nar-C12 uptake in macrophage cells revealed that Nar-C12 ester bond was partially degraded in the cell membrane and free Nar was translocated to the cytosol. These results indicate that Nar released from Nar-C12 exerts anti-inflammatory effects by suppressing NF-κB signaling pathway.
Graphical abstract
Naringin lauroyl ester inhibits LPS-induced activation of NF-κB signaling in macrophages.
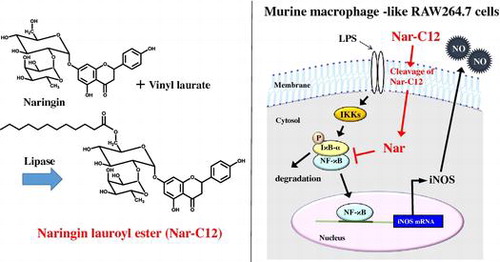
Flavonoids are a group of secondary metabolites that are found in nearly all plants and have various biological activities, including antimicrobial,Citation1) antioxidant,Citation2) anti-inflammatory,Citation3) and anti-tumorCitation4) effects. Naringin (Nar) is one of the most common citrus flavonoids and is mainly found in grapefruitCitation5); it has reported anti-atherosclerotic,Citation6) anti-oxidant,Citation7) and anti-inflammatoryCitation8) properties. As such, Nar has many potential applications in foods, cosmetics, and pharmacology.
Nitric oxide (NO) is a major mediator of inflammation that plays a critical role in bacterial clearance as part of the host defense against bacterial infection, and is produced upon activation of phagocytes such as macrophages.Citation9) In innate immunity, NO production by macrophages is stimulated by inducible NO synthase (iNOS), whose expression is induced by lipopolysaccharide (LPS), interferon-γ, interleukin (IL)-1β, IL-6, and tumor necrosis factor-α.Citation10) On the other hand, hyperactivation of macrophages by excess levels of bacterial endotoxins such as LPS leads to the overproduction of inflammatory mediators including NO, which can result in the generation of reactive nitrogen species that react with macromolecules and cause cellular damage. These reactions can trigger inflammatory diseases including fever, multi-organ failure, and septic shock.Citation11) In addition, iNOS gene expression is transcriptionally regulated by activated nuclear factor (NF)-κB and p38 mitogen-activated protein kinase (MAPK).Citation12,13) Therefore, NF-κB- and p38 MAPK-mediated iNOS expression can serve as a therapeutic target or can potentially be modulated by a diet containing flavonoids.
Naringenin (Nge) and hesperetin exert anti-inflammatory effects by suppressing iNOS expression in RAW264.7 murine macrophage cells. These effects are more potent than those of Nar and hesperidin—from which they are respectively derived—owing to their greater hydrophobicity,Citation14) suggesting that lower penetration through the cell membrane limits the efficacy of these molecules. Acylation by immobilized lipase has been proposed as a means of overcoming this problem and improving the functionality of flavonoids.Citation15–18) It has been reported that prunin alkyl (C4, C12, and C18) esters are more lipophilic than the parental molecule prunin, which is produced when Nar is degraded by α-rhamnosidases.Citation19) Moreover, these esters show antimicrobial activity, which is lacking in prunin.Citation20,21) Acylation of flavonoids improves their hydrophobicity and solubility in lipophilic media, thereby increasing their ability to interact with or even penetrate the cell membrane.Citation22,23) Although the enzymatic acylation of Nar has been previously reported,Citation24–26) only one study described the biological activities of acylated Nar derivatives and reported that their functions were not improved by the modification.Citation16)
We investigated the various biological activities of acylated Nar derivatives and found that they had an anti-inflammatory effect. The present study examined the effects of Nar alkyl (C2–C18) esters on LPS-induced NO production, iNOS expression, and activation of NF-κB and p38 MAPK signaling pathways. Our results indicate that Nar lauroyl ester (Nar-C12) has more potent anti-inflammatory activity than does Nar and its other derivatives.
Materials and methods
Materials
Nar was provided by Toyo Sugar Refining Co. (Tokyo, Japan). Nge, nobiletin, 4-Å molecular sieves, vinyl acetate, vinyl butyrate, vinyl hexanoate, vinyl octanoate, vinyl decanoate, vinyl laurate, vinyl palmitate, vinyl stearate, Dulbecco’s modified Eagle’s medium (DMEM), and penicillin-streptomycin solution were from Wako Pure Chemical Industries (Osaka, Japan). Vinyl myristate was from Tokyo Chemical Industry Co. (Tokyo, Japan). Lipozyme RM IM (immobilized lipase from Rhizomucor miehei) was gifted from Novozymes (Bagsvaerd, Denmark). Fetal bovine serum (FBS) was from Thermo Scientific (Waltham, MA, USA). 3-(4,5-Dimethylthiazol-2-yl)-2,5-diphenyl tetrazolium bromide (MTT) was from Dojindo Laboratories (Kumamoto, Japan). LPS (Escherichia coli 026:B6) was from Sigma–Aldrich Co. (St. Louis, MO, USA). Antibodies against the following proteins were used in this study: NF-κB p65 (Santa Cruz Biotechnology, Santa Cruz, CA, USA); glyceraldehyde 3-phosphate dehydrogenase (GAPDH; Millipore, Billerica, MA, USA); histone (Chemicon International, Temecula, CA, USA); and phosphorylated forms of p38 MAPK and IκB-α (Cell Signaling Technology, Danvers, MA, USA). Horseradish peroxidase (HRP)-conjugated iNOS-specific antibody has been previously described.Citation27) Anti-triose-phosphate isomerase (TPI) antibody was provided by Dr R. Yamaji (Osaka Prefecture University, Japan). RAW264.7 murine macrophage-like cells were obtained from the European Collection of Cell Cultures (Salisbury, UK).
Preparation of Nar alkyl esters
Nar alkyl esters were prepared as previously described.Citation19) Briefly, a mixture containing 19 mL of acetone, 500 mg of molecular sieves, 0.4 mmol Nar, 4 mmol vinyl ester, and 100 mg of Lipozyme RM IM in a screw-capped bottle was incubated at 50 °C. When most of the Nar had been converted to the alkyl ester form, the immobilized enzyme and molecular sieves were removed by filtration. After evaporating the acetone under reduced pressure at 40 °C, the residual material was washed three times with hexane and then lyophilized. Purity was verified by high-performance liquid chromatography (HPLC) as described below.
HPLC conditions
Reaction products were analyzed by HPLC using a Cosmosil 10C8-300 column (4.6 × 50 mm; Nacalai Tesque, Kyoto, Japan) and by ultraviolet detection at 280 nm. Elution was carried out in a linear gradient from 55% to 100% solvent A (methanol) for 4 min at a flow rate of 1.2 mL/min and 40 °C; 0.2% acetic acid solution was used as solvent B.
Cell viability assay
RAW264.7 cells (2.5 × 104/well) were pre-cultured in DMEM supplemented with 10% heat-inactivated FBS and 1% penicillin-streptomycin in 96-well microplates in a humidified 5% CO2 atmosphere at 37 °C for 24 h. The cells were incubated with or without Nar alkyl esters as described in the legend for Fig. . Cell viability was determined with the MTT assay based on mitochondrial reduction of MTT to formazan.Citation28) Briefly, 0.5% MTT in phosphate-buffered saline (PBS) was added (10 μL/well) to the culture medium (100 μL/well), followed by incubation for 2 h. Cell suspensions were then mixed with 110 μL MTT stop solution composed of 10% sodium dodecyl sulfate (SDS) in 10 mM HCl and incubated for 12 h. Absorbance was measured at 540 nm on a microplate reader (Bio-Rad, Hercules, CA, USA).
Nitrite assay
NO production by macrophages was determined by measuring the levels of nitrite, which is a relatively stable metabolite of NO. RAW264.7 cells were pre-cultured in a 96-well plate as described above for the cell viability assay and treated with different concentrations (25, 37.5, or 50 μM) of nobiletin, Nge, Nar, or Nar alkyl (C2–C12) esters for 30 min. LPS (100 ng/mL) was added to the cells followed by incubation at 37 °C for 24 h in a humidified 5% CO2 atmosphere. A 50 μL volume of culture supernatant was dispensed in triplicate into the wells of a 96-well plate and mixed with 25 μL Griess reagent A (1% sulfanilamide in 5% H3PO4) for 5 min before adding 25 μL Griess reagent B [0.1% N-(1-naphthyl)-ethylenediamine] to the mixture. After incubation for 10 min at room temperature, absorbance was measured at 540 nm on a microplate reader (Bio-Rad).
Western blot analysis
Western blotting was performed as previously described,Citation28) with some modifications. To prepare total proteins for immunoblot analysis of iNOS levels, RAW264.7 cells prepared as described in the legend for Fig. were washed with PBS and then lysed with 1 × SDS sample buffer. To obtain the nuclear fraction for an immunoblot analysis of NF-κB p65 and the cytosolic fraction for immunoblot analysis of phosphorylated IκB-α and p38 MAPK, the cell pellet was processed with the CelLytic NuCLEAR Extraction kit (Sigma-Aldrich) according to the manufacturer’s instructions. Soluble proteins from each sample were subjected to SDS-polyacrylamide gel electrophoresis on an 8% or 14% gel; separated proteins were transferred to a nitrocellulose membrane (GE Healthcare Life Science, Buckinghamshire, UK), which was blocked with 5% skim milk (Nacalai Tesque) and incubated at 4 °C overnight with antibodies against the following proteins: GAPDH, NF-κB p65, histone, phosphorylated IκB-α, phosphorylated p38 MAPK, and TPI, as well as HRP-conjugated anti-iNOS antibody. All bound antibodies except for the anti-iNOS antibody were incubated at room temperature for 1 h with HRP-conjugated anti-rabbit, -mouse (GE Healthcare Life Science), or -goat (Santa Cruz Biotechnology) IgG secondary antibody. Protein bands were detected using Luminata Forte Western HRP Substrate (Millipore) with an LAS-1000 mini image analyzer (Fujifilm, Tokyo, Japan).
Detection of iNOS levels by reverse-transcriptional (RT-) PCR
Total RNA was extracted from RAW264.7 cells prepared as described in the legend for Fig. using Sepasol-RNA I (Nacalai Tesque). Single-stranded cDNA was synthesized from total RNA using ReverTraAce and a random primer (Takara Bio, Kusatsu, Shiga, Japan) according to the manufacturer’s instructions. iNOS gene fragments were amplified using Go-Taq Green master mix (Promega, Madison, WI, USA) and the primers shown in Table . After initial denaturation at 95 °C for 2 min, cycling was carried out as follows: 95 °C for 30 s; 56 °C for 30 s (iNOS, 20 cycles) or 54 °C for 30 s (GAPDH, 20 cycles); and 72 °C for 21 s (iNOS) or 27 s (GAPDH). GAPDH expression was used as an internal standard. Conditions were determined by testing a number of cycles ranging from 20 to 35, so that no RNA had reached a plateau at the end of the amplification.
Table 1. Primer sequences used for RT-PCR.
Analysis of Nar derivative uptake in RAW264.7 cells
RAW264.7 cells (5.0 × 105/mL) were cultured in a 100-mm dish for 5 days. Cells were incubated with or without 50 μM Nge, Nar, and Nar-C12 for 3 h, then washed four times with ice-cold PBS, and scraped from the dish in 5 mL PBS. The cells were disrupted using a 23G injection needle followed by ultra-centrifugation at 100,000 × g for 1 h at 4 °C. The supernatant was used as the cytosolic fraction; the pellet was resuspended in 500 μL PBS, sonicated for 30 s using a Handy Sonic model UR-20P (Tomy Seiko Co., Tokyo, Japan) and used as the membrane fraction. Both fractions were lyophilized and dissolved with 500 μL methanol. After filtration through a 0.2-μm membrane, Nar derivative content was determined by HPLC as described above, except that a Mightysil RP-18GP column (4.6 × 250 mm; Kanto Chemical Co., Tokyo, Japan) was used and elution was carried out for 21 min at a flow rate of 0.7 mL/min.
Statistical analysis
All experiments were performed at least three times. Values are presented as the mean ± SE. Statistical significance was determined by one-way analysis of variance using Prism software (GraphPad, San Diego, CA, USA). A p value < 0.01 was considered significant.
Results and discussion
Effects of Nar alkyl esters on cell viability
Enzymatic acylation of flavonoids by lipases with fatty acids is more regioselective than chemical acylation and may enhance not only their solubility but also their stability in various media.Citation29,30) We therefore acylated Nar with different fatty acids (C2–C18) using immobilized lipase from R. miehei (Fig. ). In agreement with a previous study,Citation24) the nuclear magnetic resonance spectral analysis revealed that fatty acids bonded to the C-6 of the glucose moiety of the Nar molecule (data not shown). To characterize the biological activity of Nar derivatives, we first evaluated the effect of Nar alkyl (C2–C18) esters on the viability of RAW264.7 cells at concentrations of 25 and 50 μM. Nge, Nar, and Nar alkyl (C4–C12) esters did not affect cell viability (Fig. ); however, treatment with Nar alkyl (C14–C18) esters decreased viability in a dose-dependent manner. Prunin and hesperetin glucoside alkyl esters exert toxic effects on Jurkat cellsCitation31) by interacting with the plasma membrane. These results suggest that fatty acid with long chains (C14–C18) undermine the integrity of the plasma membrane. Nar-C2 slightly increased cell viability as determined by the MTT assay; however, it did not affect the release of lactic acid dehydrogenase from cells treated with Nar-C2 or left untreated (data not shown). Acetic acid released by Nar-C2 degradation may have affected mitochondrial dehydrogenase activity in the MTT assay.
Fig. 2. Effect of Nar alkyl esters on cell viability.
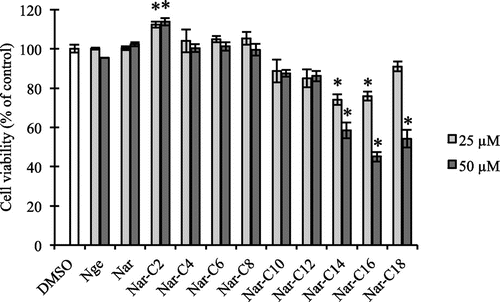
Effects of Nar alkyl esters on LPS-induced NO production
NO production is induced in inflammationCitation32); we therefore investigated the effect of Nar alkyl esters on LPS-induced NO production. Given the cytotoxicity of Nar alkyl (C14–C18) esters, we focused our analysis on Nge, Nar, and Nar alkyl (C2–C12) esters. Nobiletin, which has potent anti-inflammatory effects,Citation3) was used as a positive control. LPS-induced nitrite production, which was inhibited by Nar alkyl (C6–C12) esters in a dose-dependent manner (Fig. ); those with longer fatty acid carbon chains showed greater NO-inhibiting effects. For instance, NO generation was decreased in the presence of Nar-C12 as compared to Nge, Nar, and the other derivatives and was far higher for Nar-C12 than for nobiletin. These results suggest that Nar alkyl esters with long fatty acid carbon chains can penetrate the cell membrane, and that Nar-C12 acts as a potent inhibitor of inflammation by preventing NO accumulation, which can lead to inflammatory diseases.
Fig. 3. Effect of Nar alkyl esters on LPS-induced NO production.
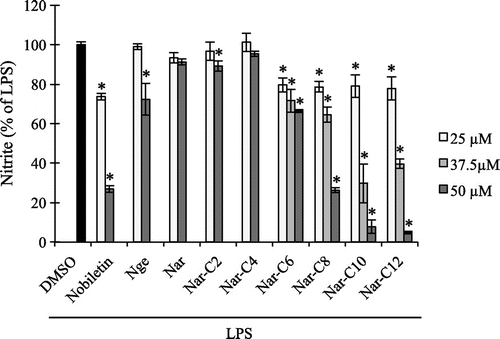
Effects of Nar-C12 on iNOS expression
To investigate whether the inhibition of NO production by Nar-C12 is due to suppression of iNOS expression, the LPS-induced expression of iNOS protein, and mRNA in the presence or absence of Nar derivatives (Nge, Nar, or Nar-C12) was analyzed by western blotting and RT-PCR, respectively. Both iNOS protein and transcript levels were negligible in unstimulated cells, but increased markedly 24 h after LPS (100 ng/mL) treatment (Fig. (A, B)). Pretreatment with Nge moderately inhibited iNOS protein and transcript expression in cells treated with LPS. As expected, Nar-C12, but not Nge or Nar, had a potent inhibitory effect on both iNOS protein and mRNA expression. These results confirm that Nar-C12 entered cells more readily than Nge or Nar as a result of its fatty acid moiety and was thereby able to modulate iNOS expression.
Fig. 4. Effect of Nar-C12 on iNOS protein and mRNA expression in RAW264.7 cells.
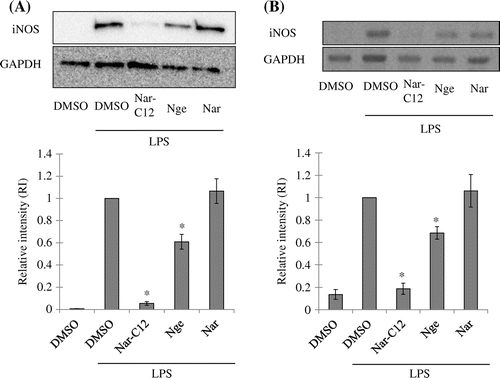
Effect of Nar-C12 on signaling pathways involved in iNOS induction
NF-κB regulates iNOS expression via an LPS-dependent pathway. The NF-κB dimer (p65/50) in resting cells is maintained in the cytosol by interaction with inhibitor of NF-κB (IκB). Upon exposure to pro-inflammatory stimuli, IκB is phosphorylated by IκB kinase, then ubiquitinated and degraded; free NF-κB dimers are then translocated to the nucleus and induce the transcription of target genes.Citation33) To determine whether NF-κB signaling is involved in the inhibition of iNOS expression by Nar-C12, LPS-induced IκB-α phosphorylation and NF-κB p65 nuclear translocation in the presence and absence of Nar-C12 were analyzed by western blotting. Nar-C12 decreased the levels of phosphorylated IκB-α in the cytosol (Fig. (A)) and of NF-κB p65 in the nucleus (Fig. (B)) of RAW264.7 cells.
Fig. 5. Effect of Nar-C12 on IκB-α phosphorylation and NF-κB activation.
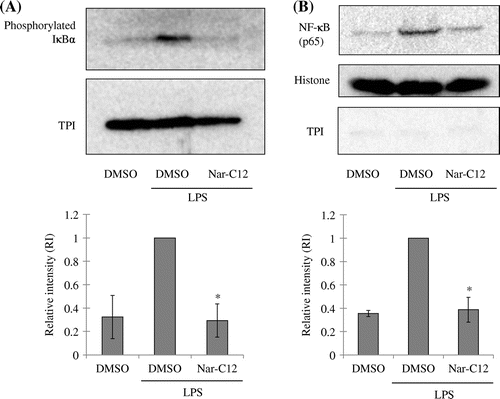
p38-MAPK phosphorylation is also important in LPS-induced iNOS expression. To determine whether p38-MAPK signaling plays a role in the inhibition of iNOS expression by Nar-C12, LPS-induced phosphorylation of p38 MAPK in the presence and absence of Nar-C12 was analyzed by western blotting. p38 MAPK phosphorylation was increased by LPS stimulation, but this was unaltered by Nar-C12 treatment under the experimental conditions (Fig. ). These results indicate that Nar-C12 may suppress iNOS expression via modulation of the NF-κB pathway. Nge and Nar have been shown to block NF-κB activation by a similar mechanism in murine macrophage RAW264.7 cells,Citation8,34) suggesting that while these related compounds all exhibit anti-inflammatory effects, their ability to permeate the cell membrane and exert these effects is determined by their hydrophobicity. A metabolite of rutin has also been reported to suppress inflammation by inhibiting iNOS expression via the NF-κB pathway.Citation35)
Fig. 6. Effect of Nar-C12 on p38 MAPK activation.
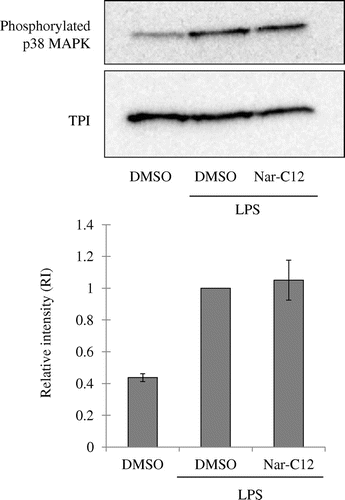
Analysis of Nar derivative uptake in RAW264.7 cells
In Nge-treated cells, Nge was detected in the cytosolic but not in the membrane fraction. In contrast, Nar was detected neither in the cytosol nor in the membrane fraction in Nar-treated cells. In cells treated with Nar-C12, Nar and Nar-C12 were detected in the membrane fraction while Nar was detected in the cytosolic fraction (Table ). These results suggest that Nar-C12 is incorporated into the cell membrane, resulting in the cleavage of the Nar-C12 ester bond by cell membrane esterases; Nar is thus released from Nar-C12 and transferred to the cytosol. Treatment of cells with 50 μM lauric acid had no effect on NO production (data not shown). These results indicate that improvement of anti-inflammatory effects by Nar-C12 depends on Nar uptake in the cytosol.
Table 2. Nar derivative uptake in RAW264.7 cells.
In summary, we synthesized nine Nar alkyl (C2–C18) esters using Lipozyme RM IM. Nar alkyl (C14–C18) esters were cytotoxic to RAW264.7 cells. Nar-C12 more potently inhibited LPS-induced NO production than other Nar derivatives by blocking NF-κB activation.
While hydrophobic flavonoids generally have high membrane permeability, Nar, which has higher water solubility than Nge, exhibits weak anti-inflammatory effects due to its low membrane permeabilityCitation14); conversely, the more potent effects of Nar-C12 against inflammation are likely due to its amphiphilic/lipophilic properties that enable it to penetrate into the cell. Based on the results shown in Table , we speculate that after incorporation of Nar-C12 in the cell membrane, the Nar-C12 ester bond is cleaved by membrane esterases, allowing free Nar to translocate to the cytosol and suppress NF-κB signaling. Alternatively, Nar-C12 may directly engage immune system components in the cell membrane. Nonetheless, the results of the present study indicate that enzymatic acylation can provide a means of enhancing the internalization of Nar by cells and exploiting its anti-inflammatory properties in therapeutics and other products.
Author contributions
H.I. and T.S. conceived and designed the study. H.H. and H.T. carried out most of the experiments. H.T., M.N., M.U., and H.I. analyzed the data. H.H., H.T., and T.S. wrote the manuscript. All authors have read and approved the final manuscript.
Funding
This work was supported by Osaka Prefecture University and in part by Toyo Sugar Refining Co.
Acknowledgments
We would like to thank Editage (www.editage.jp) for English language editing.
Disclosure statement
The authors have no conflict of interest.
Notes
Abbreviations: DMEM, Dulbecco’s modified Eagle’s medium; FBS, fetal bovine serum; GAPDH, glyceraldehyde 3-phosphate dehydrogenase; HPLC, high-performance liquid chromatography; HRP, horseradish peroxidase; IL-1β, interleukin-1β; iNOS, inducible NO synthase; IκB, inhibitor of NF-κB; LPS, lipopolysaccharide; MAPK, mitogen-activated protein kinase; MTT, 3-(4,5-dimethylthiazol-2-yl)-2,5-diphenyl tetrazolium bromide; Nar, naringin; NF-κB, nuclear factor (NF)-κB; Nge, naringenin; NO, nitric oxide; PBS, phosphate-buffered saline; RT-PCR, reverse-transcriptional PCR; SDS, sodium dodecyl sulfate; TPI, triose-phosphate isomerase.
References
- Ohemeng KA, Schwender CF, Fu KP, et al. DNA gyrase inhibitory and antibacterial activity of some flavones. Bioorg. Med. Chem. Lett. 1993;3:225–230.10.1016/S0960-894X(01)80881-7
- Chua LS. A review on plant-based rutin extraction methods and its pharmacological activities. J. Ethnopharmacol. 2013;150:805–817.10.1016/j.jep.2013.10.036
- Ihara H, Yamamoto H, Ida T, et al. Inhibition of nitric oxide production and inducible nitric oxide synthase expression by a polymethoxyflavone from young fruits of Citrus unshiu in rat primary astrocytes. Biosci. Biotechnol. Biochem. 2012;76:1843–1848.10.1271/bbb.120215
- Yoshimizu N, Otani Y, Saikawa Y, et al. Anti-tumour effects of nobiletin, a citrus flavonoid, on gastric cancer include: antiproliferative effects, induction of apoptosis and cell cycle deregulation. Aliment. Pharmacol. Ther. 2004;20:95–101.10.1111/apt.2004.20.issue-s1
- Peterson JJ, Beecher GR, Bhagwat SA, et al. Flavanones in grapefruit, lemons, and limes: a compilation and review of the data from the analytical literature. J. Food Compost. Anal. 2006;19:S74–S80.10.1016/j.jfca.2005.12.009
- Chanet A, Milenkovic D, Deval C, et al. Naringin, the major grapefruit flavonoid, specifically affects atherosclerosis development in diet-induced hypercholesterolemia in mice. J. Nutr. Biochem. 2011;23:469–477.
- Gorinstein S, Leontowicz H, Leontowicz M, et al. Changes in plasma lipid and antioxidant activity in rats as a result of naringin and red grapefruit supplementation. J. Agric. Food Chem. 2005;53:3223–3228.10.1021/jf058014h
- Liu Y, Su WW, Wang S, et al. Naringin inhibits chemokine production in an LPS-induced RAW 264.7 macrophage cell line. Mol. Med. Rep. 2012;6:1343–1350.
- Bogdan C. Nitric oxide and the immune response. Nat. Immunol. 2001;2:907–916.10.1038/ni1001-907
- Lowenstein CJ, Snyder SH. Nitric oxide, a novel biologic messenger. Cell. 1992;70:705–707.10.1016/0092-8674(92)90301-R
- Boscá L, Zeini M, Través PG, et al. Nitric oxide and cell viability in inflammatory cells: a role for NO in macrophage function and fate. Toxicology. 2005;208:249–258.10.1016/j.tox.2004.11.035
- Kleinert H, Schwarz PM, Förstermann U. Regulation of the expression of inducible nitric oxide synthase. Biol. Chem. 2003;384:1343–1364.
- Chen BC, Chen YH, Lin WW. Involvement of p38 mitogen-activated protein kinase in lipopolysaccharide-induced iNOS and COX-2 expression in J774 macrophages. Immunology. 1999;97:124–129.10.1046/j.1365-2567.1999.00747.x
- Lin HY, Shen SC, Chen YC. Anti-inflammatory effect of heme oxygenase 1: glycosylation and nitric oxide inhibition in macrophages. J. Cell Physiol. 2005;202:579–590.10.1002/(ISSN)1097-4652
- Gatto MT, Falcocchio S, Grippa E, et al. Antimicrobial and anti-lipase activity of quercetin and its C2-C16 3-O-acyl-esters. Biorg. Med. Chem. 2002;10:269–272.10.1016/S0968-0896(01)00275-9
- Mellou F, Loutrari H, Stamatis H, et al. Enzymatic esterification of flavonoids with unsaturated fatty acids: effect of the novel esters on vascular endothelial growth factor release from K562 cells. Process Biochem. 2006;41:2019–2034.
- Viskupicova J, Danihelova M, Ondrejovic M, et al. Lipophilic rutin derivatives for antioxidant protection of oil-based foods. Food Chem. 2010;123:45–50.10.1016/j.foodchem.2010.03.125
- Salem JH, Humeau C, Chevalot I, et al. Effect of acyl donor chain length on isoquercitrin acylation and biological activities of corresponding esters. Process Biochem. 2010;45:382–389.10.1016/j.procbio.2009.10.012
- Céliz G, Daz M. Biocatalytic preparation of alkyl esters of citrus flavanone glucoside prunin in organic media. Process Biochem. 2011;46:94–100.10.1016/j.procbio.2010.07.022
- Céliz G, Audisio MC, Daz M. Antimicrobial properties of prunin, a citric flavanone glucoside, and its prunin 6″-O-lauroyl ester. J. Appl. Microbiol. 2010;109:1450–1457.10.1111/jam.2010.109.issue-4
- Céliz G, Daz M, Audisio MC. Antibacterial activity of naringin derivatives against pathogenic strains. J. Appl. Microbiol. 2011;111:731–738.10.1111/jam.2011.111.issue-3
- Mellou F, Lazari D, Skaltsa H, et al. Biocatalytic preparation of acylated derivatives of flavonoid glycosides enhances their antioxidant and antimicrobial activity. J. Biotechnol. 2005;116:295–304.10.1016/j.jbiotec.2004.12.002
- Kodelia G, Athanasiou K, Kolisis FN. Enzymatic synthesis of butyryl-rutin ester in organic solvents and its cytogenetic effects in mammalian cells in culture. Appl. Biochem. Biotechnol. 1994;44:205–212.10.1007/BF02779657
- Kontogianni A, Skouridou V, Sereti V, et al. Regioselective acylation of flavonoids catalyzed by lipase in low toxicity media. Eur. J. Lipid Sci. Techno. 2001;103:655–660.10.1002/(ISSN)1438-9312
- Kontogianni A, Skouridou V, Sereti V, et al. Lipase-catalyzed esterification of rutin and naringin with fatty acids of medium carbon chain. J. Mol. Catal. B: Enzym. 2003;21:59–62.10.1016/S1381-1177(02)00139-X
- Youn SH, Kim HJ, Kim TH, et al. Lipase-catalyzed acylation of naringin with palmitic acid in highly concentrated homogeneous solutions. J. Mol. Catal. B: Enzym. 2007;46:26–31.10.1016/j.molcatb.2007.02.002
- Nakamura Y, Kitagawa T, Ihara H, et al. Potentiation by high potassium of lipopolysaccharide-induced nitric oxide production from cultured astrocytes. Neurochem. Int. 2006;48:43–49.10.1016/j.neuint.2005.08.002
- Maeda R, Ida T, Ihara H, et al. Immunostimulatory activity of polysaccharides isolated from Caulerpa lentillifera on macrophage cells. Biosci. Biotechnol. Biochem. 2012;76:501–505.10.1271/bbb.110813
- Fossen T, Cabrita L, Andersen OM. Colour and stability of pure anthocyanins influenced by pH including the alkaline region. Food Chem. 1998;63:435–440.10.1016/S0308-8146(98)00065-X
- Ishihara K, Nakajima N. Structural aspects of acylated plant pigments: stabilization of flavonoid glucosides and interpretation of their functions. J. Mol. Catal. B: Enzym. 2003;23:411–417.10.1016/S1381-1177(03)00106-1
- Céliz G, Alfaro FF, Cappellini C, et al. Prunin- and hesperetin glucoside-alkyl (C4–C18) esters interaction with Jurkat cells plasma membrane: consequences on membrane physical properties and antioxidant capacity. Food Chem. Toxicol. 2013;55:411–423.10.1016/j.fct.2013.01.011
- Nathan CF, Hibbs JB Jr. Role of nitric oxide synthesis in macrophage antimicrobial activity. Curr. Opin. Immunol. 1991;3:65–70.10.1016/0952-7915(91)90079-G
- Ghosh S, May MJ, Kopp EB. NF-κB and Rel proteins: evolutionarily conserved mediators of immune responses. Annu. Rev. Immunol. 1998;16:225–260.10.1146/annurev.immunol.16.1.225
- Tsai SH, Lin-Shiau SY, Lin JK. Suppression of nitric oxide synthase and the down-regulation of the activation of NFκB in macrophages by resveratrol. Br. J. Pharmacol. 1999;126:673–680.10.1038/sj.bjp.0702357
- Su KY, Yu CY, Chen YP, et al. 3,4-Dihydroxytoluene, a metabolite of rutin, inhibits inflammatory responses in lipopolysaccharide-activated macrophages by reducing the activation of NF-κB signaling. BMC Complement. Altern. Med. 2014;14:21.10.1186/1472-6882-14-21