Abstract
Tertiary interactions between a new RNA motif and RNA tetraloops were analyzed to determine whether this new motif shows preference for a GCGA tetraloop. In the structural context of a ligase ribozyme, this motif discriminated GCGA loop from 3 other tetraloops. The affinity between the GCGA loop and its receptor is strong enough to carry out the ribozyme activity.
Interactions between RNA hairpins capped by GNRA tetraloops (where N is any of the 4 bases and R is purine) and their receptor motifs (termed L/R interactions) constitute a major family of RNA tertiary interactions.Citation1,2) As L/R interactions allow 2 RNA helices to assemble with a defined orientation, they play important roles in forming and maintaining defined 3-dimensional (3D) RNA structures.Citation1,2) Among the L/R interactions, 3 L/R pairs, which are GAAA loop and R(11nt) motif, Citation3) GGAA loop and R(1) motif,Citation4) and GUAA loop and R(B7.8) motif,Citation5) have been shown to form L/R complexes with excellent binding specificity and high binding affinity. Due to their structural modularity, the 3 L/R motifs have been used as building blocks for artificial design and construction of RNA nanostructures, which are promising scaffolds for nanobiotechnology and synthetic biology.Citation6) To expand the potency of RNA structural design with L/R interactions, further accumulation and characterization of novel L/R interactions are desirable. We are interested in a new RNA motif that was identified recently in a riboswitch controlled by cAMP/GMP. In the 3D structure of the cAMP/GMP riboswitch, this motif, designated as the R(GCGA) motif in this study, recognizes a GCGA tetraloop, whose receptor motif was not identified before the analysis of the cAMP/GMP riboswitch.Citation7) Therefore, we evaluated the binding ability and specificity of the R(GCGA) motif.
For convenient estimation of the binding specificity of modular GNRA receptors, we developed an assay system in which self-splicing reaction of a bimolecular ribozyme can be detected through the production of a fluorescent RNA aptamer. Spinach RNA is an aptamer that captures DFHBI (3,5-difluoro-4-hydroxybenzylidene imidazolinone), and the resulting DFHBI-RNA complex results in strong fluorescence of DFHBI that is non-emissive in the free state.Citation8) We engineered a bimolecular derivative of the Tetrahymena group I self-splicing intron ribozyme (Fig. (B))Citation9) to insert the ΔP5 ribozyme as an intron into the spinach RNA aptamer sequence. Self-splicing of the intron ribozyme yields the spinach RNA as ligated exons, production of which can be monitored by the increase in fluorescence of DFHBI-spinach complex. Self-splicing reaction of the ΔP5 ribozyme requires complex formation with the activator P5abc RNA (Fig. (B)). Tertiary interaction between GNRA tetraloop in P5abc and its receptor in P6 of the ΔP5 ribozyme serves as one of the 3 interactions (P5b/P6, P5c/P2, and P5a/P4) supporting the association of the 2 RNA components.Citation10,11)
Fig. 1. Interactions between tetraloops and their receptors in 2 modular ribozymes.
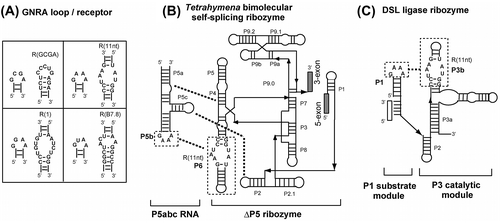
In the bimolecular Tetrahymena ribozyme at low (<10 mM) Mg2+ ion concentration, the stability of GNRA/receptor interaction directly governs the ribozyme activity because the P5b/P6 interaction contributes significantly to the complex formation. Therefore, we examined the target specificity of 3 known receptor motifs with 5 mM Mg2+ ions (Fig. (A)–(C)). The ΔP5 ribozyme with a receptor motif (R(11nt), R(1), or R(B7.8)) in P6 showed no fluorescence without P5abc (Fig. (A)–(C)). Each ribozyme gave distinctly higher fluorescence in the presence of P5abc RNA possessing the matched GNRA loop than those with mismatched GNRA loops (Fig. (A)–(C)). This observation indicated that the self-splicing reaction of the ΔP5 ribozyme occurred in a P5abc-dependent manner to yield spinach RNA.
Fig. 2. Catalytic activities of 2 modular ribozymes.
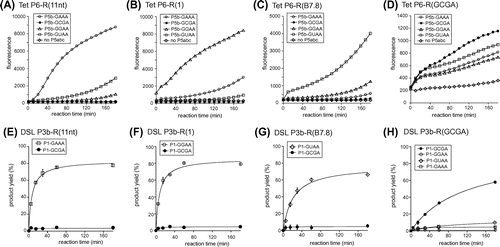
RNA–RNA tertiary interactions maintaining RNA 3D structures require Mg2+ ions.Citation1,2) Among the 3 bimolecular ribozyme complexes with cognate P5b/P6 pair, the pair of R(11nt)-ribozyme and the GAAA-P5abc showed faster increases in emission than the other 2 pairs. The order of increase in fluorescence was consistent with the order of binding affinities between the GNRA loop and its cognate receptor (GAAA/R(11) > GGAA/R(1) > GUAA/R(B7.8)) determined in other assay systems based on the catalytic activity of the DSL ribozyme and the dimerizing ability of tectoRNA.Citation2) These observations indicated that the fluorescent monitoring system can be used to evaluate RNA–RNA interactions.
The target specificity of each of the 3 receptor motifs was also tested by adding P5abc RNA with a mismatched GNRA loop in P5b. Although splicing reactions of the ΔP5 ribozyme were much less efficient with mismatched P5abc activator, ribozymes with R(11nt), R(1), and R(B7.8) were weakly promoted by P5abc with GUAA, GAAA, and GGAA in P5b, respectively (Fig. (A)–(C)). In the presence of 5 mM Mg2+ ions, 3 ΔP5 ribozymes were not activated by P5abc RNA possessing the P5b GCGA loop.
We then tested the P5abc-dependent splicing of the ΔP5 ribozyme with the R(GCGA) motif in the P6 region. In the presence of 5 mM Mg2+, however, the reaction solution showed no fluorescence even in the presence of a matched pair of ribozyme and activator with the R(GCGA) motif and GCGA loop (data not shown). This result suggested that the interaction between the GCGA loop and R(GCGA) motif may require a higher concentration of Mg2+ ions than the other 3 pairs. Therefore, we examined the bimolecular splicing reaction in the presence of 15 mM Mg2+ ions, where P5abc-dependent reactions of the ΔP5 ribozyme were still observed. In the presence of 15 mM Mg2+ ions, however, binding between the ΔP5 ribozyme and P5abc was considerably less specific than the reaction in the presence of 5 mM Mg2+ ions. Mismatched P5abc RNA also conducted splicing reactions, although the fluorescence was lower than that of matched complex with P6-R(GCGA) and P5b-GCGA loop (Fig. (D)). The results shown in Fig. (A)–(C) indicate that the bimolecular splicing system producing spinach RNA is convenient when the target RNA–RNA interaction is established at a low Mg2+ ion concentration. On the other hand, Fig. (D) shows that this system has a drawback in the presence of a higher (>10 mM) Mg2+ ion concentration. In the presence of 15 mM Mg2+ ions, the bimolecular ribozyme was shown to retain partial catalytic ability even without P5b/P6 interactionCitation11) because the remaining 2 tertiary interactions (P5a/P4 and P5c/P2) were strengthened by 15 mM Mg2+ ions and considerably supported complex formation. Therefore, the binding specificity of P5b/P6 interaction was partially dispensable.Citation11)
We next examined an alternative assay, which enabled us to evaluate the binding properties of the GCGA loop and its receptor motif even in the presence of a high Mg2+ concentration. As a system suitable for analyzing L/R interaction without indirect contribution of Mg2+ ions, we employed an artificial ribozyme termed the DSL ligase ribozyme.Citation12) The P3 catalytic domain of the DSL ribozyme holds its P1 substrate helix via GNRA/receptor interaction. As no other interaction participates in the substrate/catalytic domain association, Mg2+ ions are incapable of assisting in substrate/catalytic domain association indirectly through enhancement of other tertiary interactions.Citation13) Thus, Mg2+ ions can contribute only directly to the association of P1/P3 domains through GNRA/receptor interaction.
We prepared 4 catalytic domain variants with distinct receptor motifs. In each variant, we prepared 2 (or 4) substrate domains with matched and mismatched GNRA tetraloops (Fig. (E)–(G)). In the presence of 50 mM Mg2+ ions, 3 DSL ribozymes with R(11nt), R(1), and R(B7.8) receptor motifs in P3b exhibited RNA–RNA ligation activity depending on their cognate tetraloops in the P1 substrate domain. In contrast, variants with GCGA loops were virtually inactive even with 50 mM Mg2+ ions (Figs. (E)–(G)). The fourth variant with R(GCGA) in the catalytic domain showed ribozyme activity with its cognate P1 GCGA loop although its activity was slightly lower than those of the 3 variants with matched loop/receptor pairs (Fig. (H)). On the other hand, the R(GCGA) variants were hardly active with 3 mismatched P1 loops. These results indicated that the R(GCGA) receptor serves as a receptor motif that can discriminate the GCGA loop from GAAA, GGAA, and GUAA loops, and the GCGA/R(GCGA) pair requires higher Mg2+ concentrations than the other 3 pairs for stable association of the tetraloop and receptor motif. In comparison of DSL ribozyme activities of 4 variants with distinct receptor motifs, the P1-GCGA/P3b-R(GCGA) pair showed less efficient reaction than the remaining 3 pairs (Figs. (E)–(H)). On the other hand, the GCGA/R(GCGA) interaction was shown to be markedly stronger than a primitive loop/receptor pair composed of a GUGA loop and 5′-CU-3′:5′-AG-3′ base pairsCitation2) because this primitive pair was incapable of supporting DSL ribozyme activity even with 60 mM Mg2+ ions (data not shown).
In this study, we analyzed the binding ability and specificity of a receptor motif that recognizes a GCGA tetraloop. This L/R pair may not be suitable as an element for selective formation of the bimolecular complex derived from large ribozymes the assembly of which is supported by multiple tertiary interactions (Figs. (B) and (D)). However, the GCGA/R(GCGA) pair was capable of activating the DSL ribozyme by selective binding between the substrate and catalytic domains (Fig. (H)). The GCGA/R(GCGA) pair can serve as a new molecular part for artificial design and construction of RNA tertiary structures and assemblies including small ribozymes and tectoRNAs, in which their folding and assembly are governed by a very limited number of tertiary interactions.Citation6)
Author contributions
Yoshiya Ikawa and Shigeyoshi Matsumura conceived and designed the experiments. Airi Furukawa and Takaya Maejima contributed reagents/materials/analysis tools and also performed the experiments. Airi Furukawa, Takaya Maejima, Shigeyoshi Matsumura and Yoshiya Ikawa analyzed data and wrote the paper.
Disclosure statement
No potential conflict of interest was reported by the authors.
Funding
This work was supported by the Ministry of Education, Culture, Sports, Science, and Technology (MEXT), Japan under Grants-in-Aid for Scientific Research on Innovative Area “Synthetic Biology” to Y. I. [grant number 23119005]; Scientific Research (C) to Y. I. [grant number 15K05561]; and Research Activity Start-up to S. M. [grant number 26891009].
References
- Butcher SE, Pyle AM. The molecular interactions that stabilize RNA tertiary structure: RNA motifs, patterns, and networks. Acc. Chem. Res. 2011;44:1302–1311.10.1021/ar200098t
- Ishikawa J, Fujita Y, Maeda Y, et al. GNRA/receptor interacting modules: versatile modular units for natural and artificial RNA architectures. Methods. 2011;54:226–238.10.1016/j.ymeth.2010.12.011
- Costa M, Michel F. Frequent use of the same tertiary motif by self-folding RNAs. EMBO J. 1995;14:1276–1285.
- Geary C, Baudrey S, Jaeger L. Comprehensive features of natural and in vitro selected GNRA tetraloop-binding receptors. Nucleic Acids Res. 2008;36:1138–1152.
- Costa M, Michel F. Rules for RNA recognition of GNRA tetraloops deduced by in vitro selection: comparison with in vivo evolution. EMBO J. 1997;16:3289–3302.10.1093/emboj/16.11.3289
- Ishikawa J, Furuta H, Ikawa Y. RNA tectonics (tectoRNA) for RNA nanostructure design and its application in synthetic biology. Wiley Interdiscip. Rev. RNA. 2013;4:651–664.
- Ren A, Wang XC, Kellenberger CA, et al. Structural basis for molecular discrimination by a 3′,3′-cGAMP sensing riboswitch. Cell Rep. 2015;11:1–12.10.1016/j.celrep.2015.03.004
- Warner KD, Chen MC, Song W, et al. Structural basis for activity of highly efficient RNA mimics of green fluorescent protein. Nat. Struct. Mol. Biol. 2014;21:658–663.10.1038/nsmb.2865
- Williams KP, Fujimoto DN, Inoue T. A region of group I introns that contains universally conserved residues but is not essential for self-splicing. Proc. Natl. Acad. Sci. USA. 1992;89:10400–10404.10.1073/pnas.89.21.10400
- Lehnert V, Jaeger L, Michel F, et al. New loop-loop tertiary interactions in self-splicing introns of subgroup IC and ID: a complete 3D model of the Tetrahymena thermophila ribozyme. Chem. Biol. 1996;3:993–1009.10.1016/S1074-5521(96)90166-0
- Naito Y, Shiraishi H, Inoue T. P5abc of the Tetrahymena ribozyme consists of three functionally independent elements. RNA. 1998;4:837–846.10.1017/S1355838298972016
- Ikawa Y, Tsuda K, Matsumura S, et al. De novo synthesis and development of an RNA enzyme. Proc. Natl. Acad. Sci. USA. 2004;101:13750–13755.10.1073/pnas.0405886101
- Fujita Y, Tanaka T, Furuta H, et al. Functional roles of a tetraloop/receptor interacting module in a cyclic di-GMP riboswitch. J. Biosci. Bioeng. 2012;113:141–145.10.1016/j.jbiosc.2011.10.004