Abstract
We studied the role of genes encoding the cAMP-dependent protein kinase A catalytic subunit (PKAc) in the ligninolytic system in Pleurotus ostreatus. The wild-type P. ostreatus strain PC9 has two PKAc-encoding genes: PKAc1 and PKAc2 (protein ID 114122 and 85056). In the current study, PKAc1 and PKAc2 were fused with a β-tubulin promoter and introduced into strain PC9 to produce the overexpression strains PKAc1-97 and PKAc2-69. These strains showed significantly higher transcription levels of isozyme genes encoding lignin-modifying enzymes than strain PC9, but the specific gene expression patterns differed between the two recombinant strains. Both recombinants showed 2.05–2.10-fold faster degradation of beechwood lignin than strain PC9. These results indicate that PKAc plays an important role in inducing the wood degradation system in P. ostreatus.
Graphical abstract
PKAc1 and PKAc2 play an important role in inducing the wood degradation system in P. ostreatus. Probably, the effects are mainly mediated by unknown pathways independent of CaM.
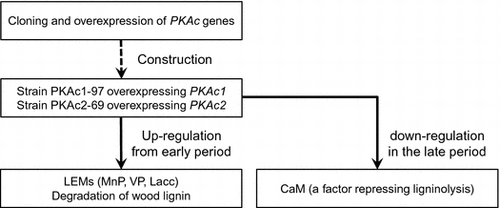
White-rot fungi are the only organisms known to be capable of completely mineralizing wood lignin,Citation1) which makes them key players in the global carbon cycle.Citation2) While a combination of extracellular lignin-modifying enzymes (LMEs), organic acids, H2O2-generating enzymes, and other incompletely characterized components is considered to be important for lignin degradation,Citation3,4) many details remain unclear. There are four major families of LMEs: lignin peroxidases (LiPs), manganese peroxidases (MnPs), versatile peroxidases (VPs), and high-redox potential laccases (Laccs).Citation5,6) Although several controversial examples where none of these enzymes are present have been recently described,Citation7) the existence of at least one of the families, especially LiPs, MnPs, and VPs, is generally considered to be important for white rot. In the context of using these enzymes for delignification and detoxification in industrial applications,Citation8) LME genes are frequently targeted for molecular breeding of white-rot fungi.Citation9) There are several examples of recombination resulting in a marked improvement in the ligninolytic activity of the host white-rot fungus. In Pleurotus ostreatus strain PC9, overexpression of VP1, formerly referred to as mnp4, improved the mineralization rate of [14C]-lignin contained in cotton stalks with and without Mn2+ from 1.4 and 1.0% to 1.69 and 1.28%, respectively.Citation10) The ligninolytic activity of Phanerochaete sordida YK-624 against beechwood powder was improved 1.3-fold by overexpression of a gene encoding manganese peroxidase.Citation11) To find additional potential targets for molecular breeding, we need to elucidate the entire lignin degradation system, especially the overall regulatory pathway.
Previous studies using Phanerochaete chrysosporium, the most widely studied white-rot fungus, have suggested the importance of increased intracellular cAMP concentration for LME expression and lignin degradation.Citation12–16) However, little is known regarding the involvement of genes of the cAMP signal transduction pathway in lignin degradation. In our previous study, another white-rot fungus, P. ostreatus, which produces MnP, VP, and Lacc, but not LiP,Citation5,17–19), was transformed with a mutated gene encoding the alpha subunit of a heterotrimeric G protein, resulting in increases in intracellular cAMP and extracellular Lacc activity.Citation20) However, the cAMP overproduction induced transcription of the gene encoding calmodulin (CaM), which has a negative effect on the expression of all the LMEs in P. ostreatus.Citation21) Moreover, lignin degradation by the P. ostreatus recombinant overexpressing CaM was slightly lower than that by the wild-type strain.Citation21) This suggests there is another pathway mainly regulating the ligninolytic system, which is induced in parallel with an increase of intracellular cAMP, but branches from the minor pathway containing CaM.
In the present study, two genes encoding isoforms of the protein kinase A (PKA) catalytic subunit (PKAc), which is one of the best-described factors activated by cAMP, were overexpressed in P. ostreatus strain PC9. The results suggested that PKAc has an important role in inducing degradation of lignin and holocellulose.
Materials and methods
Strain and culture conditions
Three P. ostreatus monokaryotic strains, the wild-type strain PC9, PKAc1-overexpressing strain PKAc1-97, and PKAc2-overexpressing strain PKAc2-69, were used throughout this study. The fungal strains were grown and maintained in potato dextrose agar (PDA; Becton, Dickinson and Company, USA) medium. To evaluate enzyme activity and gene transcription, the fungi were pre-cultured on solid PDA medium at 28 °C for 7–10 days. Fungal discs were then cut out from the edge of colonies on the pre-culture plates using a sterilized 6-mm-diameter cork borer. Four disks were inoculated into 20 mL of glucose–peptone liquid (GP) medium (2% w/v glucose, 0.5% w/v polypeptone (Becton Dickinson), 0.2% w/v yeast extract (Becton, Dickinson and Company, USA), 0.1% w/v KH2PO4, and 0.05% w/v MgSO4·7H2O) containing 0.27 mM MnSO4 in a 300-mL Erlenmeyer flask and incubated at 28 °C in the dark.
Determination of LME activity
Mn2+-dependent oxidation activity, which represents the total activity of MnP and VP, was assayed as described below. The enzyme solution was incubated with 400 μM guaiacol, 50 mM Na–lactate buffer (pH 4.5), 200 μM MnSO4, and 100 μM H2O2. The extinction coefficient of oxidized guaiacol at 465 nm is 12,100 M−1 cm−1. Mn2+-dependent oxidation activity was calculated by subtracting the activity in the absence of Mn2+ from that in the presence of 200 μM MnSO4.
Lacc activity was assayed using 2,2′-azino-bis(3-ethylbenzthiazoline-6-sulfonic acid) (ABTS) as the substrate. The enzyme was incubated with 500 μM ABTS and 100 mM citrate buffer (pH 3.0). The extinction coefficient of oxidized ABTS at 436 nm is 29,300 M−1 cm−1.
Cloning and overexpression of PKAc genes
All primers used for cloning and vector construction are listed in Table . The amino acid sequences of Ustilago maydis adr1 and uka1Citation22) were used to perform a BLASTp search against the P. ostreatus strain PC9 genome database to identify PKAc homologs. This search suggested that P. ostreatus has two PKAc-encoding genes, referred to as PKAc1 and PKAc2 (protein IDs 114122 and 85056 in P. ostreatus PC9 v1.0; http://genome.jgi.doe.gov/).
Table 1. Primers used in the present study.
We constructed PKAc1- and PKAc2-overexpression vectors, pB-TUB_Po114122 and pB-TUB_Po85056 (Fig. ), by inserting the PKAc1 and PKAc2 open reading frames (ORFs) downstream of the β-tubulin promoter of the pB-TUB_DEST_GFP vectorCitation21) using the Gateway system (Life Technologies). The β-tubulin promoter has higher expression activity in strain PC9 than other constitutive promoters.Citation23)
Fig. 1. Physical maps of the plasmid vectors.
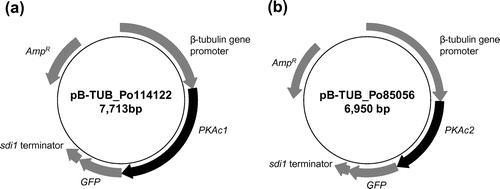
pB-TUB_Po114122 and pB-TUB_Po85056 were co-transformed with pTM1 into P. ostreatus strain PC9 as described previouslyCitation24,25) to construct the PKAc1- and PKAc2-overexpressing strains PKAc1-97 and PKAc2-69, respectively. Full-length insertion of the DNA cassettes into the genomes of transformants was confirmed by genomic PCR.
Quantitative real-time reverse transcription polymerase chain reaction (RT-PCR) assay
Total RNA was isolated from hyphae using an RNeasy Plant Mini Kit (Qiagen, Netherlands) according to the manufacturer’s protocol. Following treatment with RNase-free DNase (Takara), the mRNA was reverse transcribed using a PrimeScript RT Reagent Kit (Takara) according to the manufacturer’s instructions. Quantitative real-time RT-PCR amplification was carried out for all MnP, VP, Lacc, and PKAc isozyme genes (Table ). The glyceraldehyde 3-phosphate dehydrogenase gene (gpd) (protein_id 88005) was used as an endogenous reference gene. Amplification was performed using gene-specific primers (Table ) and SYBR Premix Ex Taq II (Takara). A standard curve for each gene was constructed from real-time PCR results using a dilution series of the PCR product made mostly with the same primer pair template as was used in the real-time RT-PCR. If there was non-specific amplification from the original primers, possibly because of repetition of the same PCR, alternate primer pairs were designed to generate a template for the construction of the standard curve (Table ). The transcription levels of each gene were extrapolated based on the standard curves.
Lignin and holocellulose measurement
To evaluate their ability to degrade lignin and holocellulose, the strains were cultured on PDA and disks were prepared as above. Four disks were then inoculated into wood flour medium containing 2 g of dried beech (Fagus crenata) wood powder (35–60 mesh) and 10 mL of 0.2%(w/v) yeast extract and then incubated at 28 °C and > 60% humidity in the dark for 5 weeks. Following culture, the wood powder was collected on cylindrical filter paper and placed into a Soxhlet extractor and refluxed for 6 h with 150 mL of solvent (benzene:ethanol = 2:1 (v/v)). The defatted wood flour was used for measurement of lignin and holocellulose.
The lignin content was measured by Klason lignin quantification.Citation26) Briefly, 200 mg of the dried wood flour was placed into a glass beaker, pre-swollen with 3 mL of 72% H2SO4, and stirred every hour for 4 h. The mixture was diluted with 112 mL of ddH2O and boiled for 4 h under reflux to maintain a constant volume. After cooling, the Klason lignin was collected by vacuum filtration using a glass filter (G4). The weight of acid-soluble lignin was calculated by the measurement of maximum absorbance of the filtrate at 200–205 nm (105 (g/L)−1 cm−1 was used as the absorbance coefficient of beech lignin). After drying at 105 °C for 5 h, the weight of Klason lignin was calculated by subtracting the weight of the glass filter from the total weight of the glass filter and Klason lignin.
Chlorite holocelluloseCitation27) was measured as holocellulose remaining in the culture. Briefly, 500 mg of the dried wood flour, 30 mL of ddH2O, 50 μL of acetic acid, and 0.2 g of sodium chlorite were placed into a 300-mL Erlenmeyer flask. A glass bead (30 mm in diameter) was put on top of the flask. The mixture was heated in a water bath at 70 °C. After 60 min, 50 μL of acetic acid and 0.2 g of sodium chlorite were added, the flask was shaken well and incubation at 70 °C was continued. The addition of 50 μL of acetic acid and 0.2 g of sodium chlorite was repeated every hour until the lignin was completely separated from the sample. The total time for the reaction was 7 h. The sample was cooled, collected by vacuum filtration using a glass filter (G2) and washed with cold water and acetone. After drying at 105 °C for 5 h, the weight of chlorite holocellulose was calculated by subtracting the weight of the glass filter from the total weight of the glass filter and chlorite holocellulose.
Statistical analyses
The enzyme activity data were analyzed using two-way repeated-measures analysis of variance (ANOVA). The transcript level and mycelial growth data were analyzed using two-way factorial ANOVA. The lignin and holocellulose degradation data were analyzed using one-way ANOVA. Sphericity assumptions were confirmed using Mauchly’s sphericity test (p > 0.05). Significant differences between the strains were determined by the Holm–Bonferroni method using R (http://www.r-project.org).
Results
Construction of strains PKAc1-97 and PKAc2-69
To understand the effect of the PKAc1 and PKAc2 genes in P. ostreatus, we constructed the PKAc1-overexpressing strain PKAc1-97 and PKAc2-overexpressing strain PKAc2-69 by introducing pB-TUB_Po114122 and pB-TUB_Po85056, respectively, into the P. ostreatus strain PC9 (Fig. ). Real-time RT-PCR analysis was performed to determine the effects of PKAc overexpression during stationary culture using GP medium containing 0.27 mM MnSO4. The PKAc1-97 and PKAc2-69 strains showed higher levels of PKAc1 and PKAc2 transcription, respectively, than strain PC9 (Fig. ). The dry weights of mycelia from the PKAc1- and PKAc2-overexpressing strains in stationary cultures grown in GP liquid medium containing 0.27 mM MnSO4 were slightly less than in the wild-type strain (Fig. ).
Fig. 2. Time course studies of absolute PKAc1 (a) and PKAc2 (b) transcript levels.
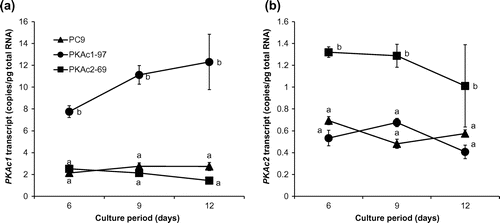
Fig. 3. Time course of dry weight of mycelia, which were cultured in GP liquid medium containing 0.27 mM MnSO4.
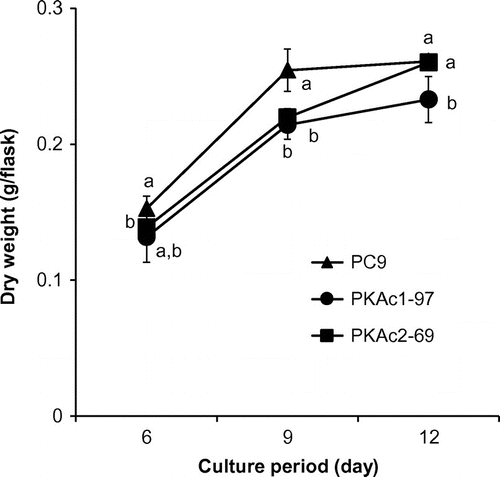
Effect of PKAc gene overexpression on enzyme activities
Lacc- and Mn2+-dependent oxidation activity levels were measured in a time course experiment. Strains PKAc1-97 and PKAc2-69 showed up to two times and four times greater Lacc activity than strain PC9, respectively (Fig. (a)). During the early period (days 6–10), PKAc1-97 showed higher Mn2+-dependent oxidation activity than the other strains (Fig. (b)). A late increase in Mn2+-dependent oxidation activity was observed for PKAc2-69.
Fig. 4. Time course studies of Lacc (a) and Mn2+-dependent oxidation (b) activity.
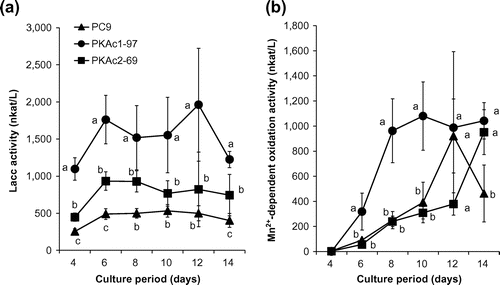
Transcription of LME isoenzyme genes
Previous whole-genome sequencing and annotation revealed that the P. ostreatus wild-type strain PC9 has 10 Lacc isozyme genes, namely PoLacc1–4, 6, 7, and 9–12 (personally annotated and denominated by Kües; http://genome.jgi.doe.gov/), 6 MnP isozyme genes, MnP1–6, and 3 VP isozyme genes, VP1–3. We performed qRT-PCR to measure the absolute quantities of transcripts of these isozyme genes. The transcript levels of all isozyme genes, with the exception of mnp5, were higher in strain PKAc1-97 than in strain PC9, though the extent and day on which a significant difference was detected between the two strains varied depending on the gene (Figs. and ). In strain PKAc2-69, about half of the isozyme genes showed a similar transcription tendency to strain PKAc1-97, whereas the transcription levels of the remaining genes were equal to or lower than in strain PC9 (Figs. and ).
Fig. 5. Time course studies of Lacc isozyme gene transcript levels.
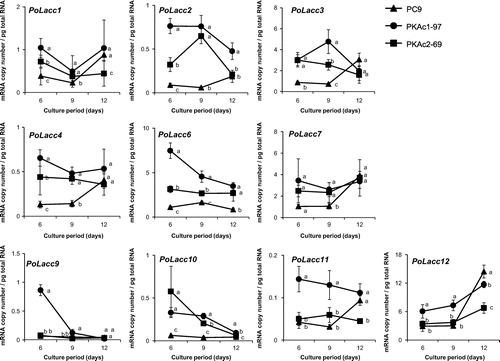
Fig. 6. Time course studies of MnP and VP isozyme gene transcript levels.
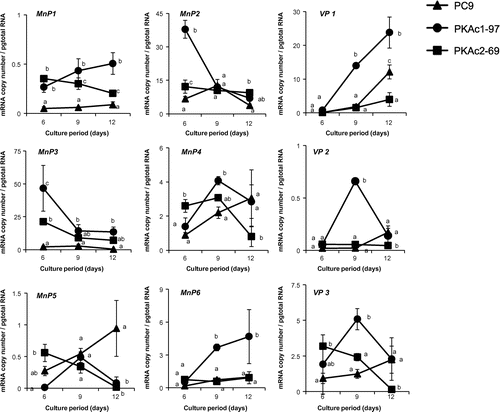
Degradability of lignin and cellulose
Beechwood degradation experiments showed that strain PC9 degraded about 18% of lignin in the flask during the culture period (Fig. (a)). PKAc1-97 and PKAc2-69 showed about 2.05- and 2.10-fold faster lignin degradation rates than strain PC9, respectively (Fig. (a)). PKAc2 overexpression seems to increase the degradability not only of lignin, but also of holocellulose. PKAc2-69 showed 2.24-fold faster holocellulose degradation rates than PC9, though it was not statistically significant (Fig. (b)).
Fig. 7. Digestibility of beech-wood powder.
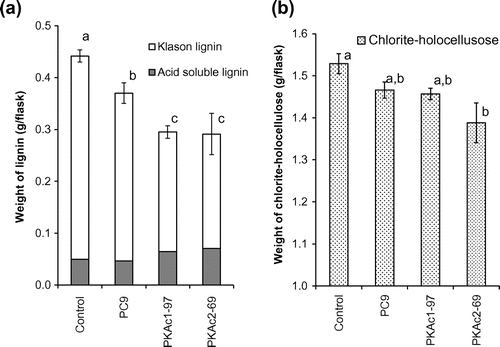
CaM gene transcription in strains PKAc1-97 and PKAc2-69
CaM, which has a negative effect on the ligninolysis system in P. ostreatus, is transcriptionally induced by intracellular cAMP in P. ostreatus.Citation21) To investigate whether PKAc1 and PKAc2 overexpression affect the transcription of the CaM gene in P. ostreatus, we measured the absolute quantity of gene transcript in strains PKAc1-97 and PKAc2-69. On day 6, CaM transcript levels in both recombinant strains were at the same level as in the wild-type strain (Fig. ), while influences of PKAc1 and PKAc2 overexpression on the expression of LME isozymes had already emerged (Figs. ). After day 6, whereas CaM transcript levels in the wild-type strain increased, those in both recombinant strains remained the same levels (Fig. ).
Fig. 8. Time course studies of Calmodulin (CaM) gene transcript levels.
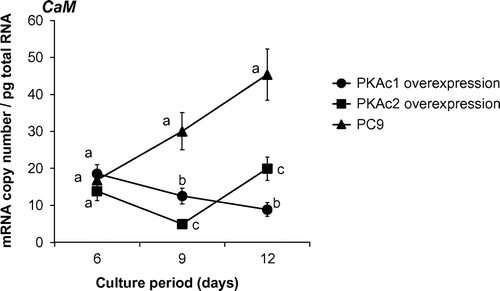
Discussion
Overall, PKAc1 overexpression had a greater positive effect on the activities and transcription levels of LMEs than PKAc2 overexpression (Figs. ). Conversely, Mn2+-dependent oxidation activity was delayed by PKAc2 overexpression (Fig. (b)). However, PKAc2 overexpression increased the absolute transcript quantities of about half of the isozyme genes encoding MnP and VP (Fig. ). These results suggest that PKAc2 overexpression affects the transcription of some MnP and VP isozyme genes, but was not enough to increase Mn2+-dependent oxidation activity. However, it is possible that differences in expression level between PKAc1 and PKAc2 caused this effect, as the absolute quantity of PKAc1 transcript in PKAc1-97 was about 10 times higher than that of PKAc2 transcript in PKAc2-69 (Fig. ). Further experiments are required to determine the exact behavior of excess PKAc molecules free from the PKA regulatory subunit.
Irrespective of the effect on expression of LMEs, both PKAc1 and PKAc2 overexpression increased the lignin degradation rate more than twofold compared with the wild-type (Fig. (a)). This is the first report that a specific gene region causes such a significant fluctuation in lignin digestibility. The culture of the PKAc2-overexpression strain also showed 2.24-fold faster holocellulose degradation rates than PC9, though it was not statistically significant (Fig. (b)). Over the last few decades, there has been emerging evidence of that non-enzymatic mechanisms mostly assisted by oxidation through the production of free hydroxyl radicals are involved in degradation of polysaccharides and lignin in plant cell walls in a non-specific manner.Citation4) There is a probability that PKAc2 overexpression might induce the non-enzymatic mechanisms.
While the influences of PKAc1 and PKAc2 overexpression on LME isozyme expression were already observed on day 6 (Fig. ), CaM transcript levels in both recombinant strains were as high as in the wild-type strain (Fig. ). This suggests that the effects of PKAc on the ligninolysis system are mainly mediated by unknown pathways independent from CaM, though PKAc affects CaM transcription after day 9 (Fig. ). It was supported by our previous observation that overexpressing CaM only slightly inhibited the lignin degradation.Citation21) We updated the putative signal transduction pathway for LME expression and lignin degradation in P. ostreatus (Fig. ) using results from this and previous reports by Suetomi et al. (2014) and Yao et al. (2013). CaM can be up- and down-regulated under cAMP relating signal pathway in P. ostreatus. There is possibility that CaM has a role to optimize quantity of oxdants required by lignin degradation to minimize the impact on fungal growth.
Fig. 9. Putative signal transduction pathway for LME expression and lignin degradation in P. ostreatus in the presence of yeast extract.
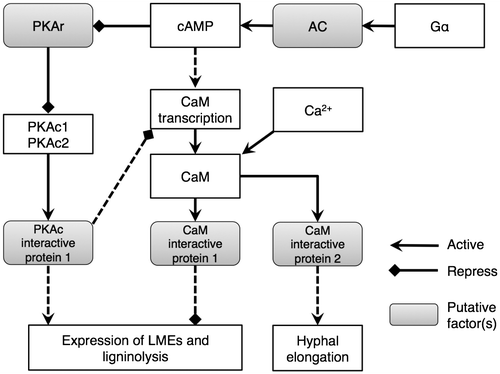
Our results clearly indicate that PKAc plays an important role in the expression of the wood degradation system in P. ostreatus. But further study, especially about behavior of LMEs and other ligninolytic factors in PKAc-overexpressing strains cultured in wood, are required to reveal the detailed mechanism of how PKAc isoforms induce ligninolysis.
Author contributions
Toshikazu Irie conceived and designed the experiments. Chihana Toyokawa, Misaki Sobu, Rie Tsukamoto, Saki Okamura, and Toshikazu Irie performed the experiments. Yoichi Honda, Hisatoshi Kamitsuji, Kousuke Izumitsu, and Kazumi Suzuki contributed reagents/materials/analysis tools. Chihana Toyokawa and Toshikazu Irie wrote the paper.
Disclosure statement
No potential conflict of interest was reported by the authors.
Funding
This work was partially supported by a grant from the FS Stage Project for Advanced Low Carbon Technology Research and the Development Program of the Japan Science and Technology Agency and by a Grant-in-Aid for Scientific Research (C) (to T.I.).
References
- Kirk TK, Connors WJ, Bleam RD, et al. Preparation and microbial decomposition of synthetic [14C]ligins. Proc. Natl. Acad. Sci. USA. 1975;72:2515–2519.10.1073/pnas.72.7.2515
- Floudas D, Binder M, Riley R, et al. The paleozoic origin of enzymatic lignin decomposition reconstructed from 31 fungal genomes. Science. 2012;336:1715–1719.10.1126/science.1221748
- Cullen D, Kersten PJ. Enzymology and molecular biology of lignin degradation. In: Brambl R, Marzluf GA, editors. Biochemistry and molecular biology. vol. III. Springer-Verlag; 2004. p. 249–273.
- Dashtban M, Schraft H, Syed TA, et al. Fungal biodegradation and enzymatic modification of lignin. Int. J. Biochem. Mol. Biol. 2010;1:36–50.
- Hofrichter M, Ullrich R, Pecyna MJ, et al. New and classic families of secreted fungal heme peroxidases. Appl. Microbiol. Biotechnol. 2010;87:871–897.10.1007/s00253-010-2633-0
- Heinzkill M, Messner K. The ligninolytic system of fungi. In: Anke T editor. Fungal biotechnology. Weinheim: Wiley-VCH; 1997. p. 213–227.
- Riley R, Salamov AA, Brown DW, et al. Extensive sampling of basidiomycete genomes demonstrates inadequacy of the white-rot/brown-rot paradigm for wood decay fungi. Proc. Natl. Acad. Sci. USA. 2014;111:9923–9928.10.1073/pnas.1400592111
- Moreno AD, Ibarra D, Alvira P, et al. A review of biological delignification and detoxification methods for lignocellulosic bioethanol production. Crit. Rev. Biotechnol. 2014;10:10.
- Janusz G, Kucharzyk KH, Pawlik A, et al. Fungal laccase, manganese peroxidase and lignin peroxidase: gene expression and regulation. Enzyme Microb Technol. 2013;52:1–12.10.1016/j.enzmictec.2012.10.003
- Salame TM, Knop D, Levinson D, et al. Release of Pleurotus ostreatus versatile-peroxidase from Mn2+ repression enhances anthropogenic and natural substrate degradation. PLoS One. 2012;7:e52446.10.1371/journal.pone.0052446
- Sugiura T, Mori T, Kamei I, et al. Improvement of ligninolytic properties in the hyper lignin-degrading fungus Phanerochaete sordida YK-624 using a novel gene promoter. FEMS Microbiol. Lett. 2012;331:81–88.10.1111/fml.2012.331.issue-1
- Sakamoto T, Yao Y, Hida Y, et al. A calmodulin inhibitor, W-7 influences the effect of cyclic adenosine 3′, 5′-monophosphate signaling on ligninolytic enzyme gene expression in Phanerochaete chrysosporium. AMB Express. 2012;2:7.10.1186/2191-0855-2-7
- MacDonald J, Suzuki H, Master ER. Expression and regulation of genes encoding lignocellulose-degrading activity in the genus Phanerochaete. Appl. Microbiol. Biotechnol. 2012;94:339–351.10.1007/s00253-012-3937-z
- Singh D, Zeng J, Chen S. Increasing manganese peroxidase productivity of Phanerochaete chrysosporium by optimizing carbon sources and supplementing small molecules. Lett. Appl. Microbiol. 2011;53:120–123.10.1111/lam.2011.53.issue-1
- Boominathan K, Reddy CA. cAMP-mediated differential regulation of lignin peroxidase and manganese-dependent peroxidase production in the white-rot basidiomycete Phanerochaete chrysosporium. Proc. Natl. Acad. Sci. USA. 1992;89:5586–5590.10.1073/pnas.89.12.5586
- MacDonald MJ, Paterson A, Broda P. Possible relationship between cyclic AMP and idiophasic metabolism in the white rot fungus Phanerochaete chrysosporium. J. Bacteriol. 1984;160:470–472.
- Fernández-Fueyo E, Ruiz-Dueñas FJ, Martínez MJ, et al. Ligninolytic peroxidase genes in the oyster mushroom genome: heterologous expression, molecular structure, catalytic and stability properties, and lignin-degrading ability. Biotechnol. Biofuels. 2014;7:2.10.1186/1754-6834-7-2
- Fernández-Fueyo E, Castanera R, Ruiz-Duenas FJ, et al. Ligninolytic peroxidase gene expression by Pleurotus ostreatus: Differential regulation in lignocellulose medium and effect of temperature and pH. Fungal Genet. Biol. 2014;18:150–161.
- Ruiz-Dueñas FJ, Fernández E, Martínez MJ, et al. Pleurotus ostreatus heme peroxidases: an in silico analysis from the genome sequence to the enzyme molecular structure. C R Biol. 2011;334:795–805.10.1016/j.crvi.2011.06.004
- Yao Y, Sakamoto T, Honda Y, et al. The white-rot fungus Pleurotus ostreatus transformant overproduced intracellular cAMP and laccase. Biosci. Biotechnol. Biochem. 2013;77:2309–2311.10.1271/bbb.130470
- Suetomi T, Sakamoto T, Tokunaga Y, et al. Effects of calmodulin on expression of lignin-modifying enzymes in Pleurotus ostreatus. Curr. Genet. 2014;61:127–140.
- Dürrenberger F, Wong K, Kronstad JW. Identification of a cAMP-dependent protein kinase catalytic subunit required for virulence and morphogenesis in Ustilago maydis. Proc. Natl. Acad. Sci. USA. 1998;95:5684–5689.10.1073/pnas.95.10.5684
- Salame TM, Knop D, Tal D, et al. Predominance of a versatile-peroxidase-encoding gene, mnp4, as demonstrated by gene replacement via a gene targeting system for Pleurotus ostreatus. Appl. Environ. Microbiol. 2012;78:5341–5352.10.1128/AEM.01234-12
- Honda Y, Matsuyama T, Irie T, et al. Carboxin resistance transformation of the homobasidiomycete fungus Pleurotus ostreatus. Curr. Genet. 2000;37:209–212.10.1007/s002940050521
- Salame TM, Yarden O, Hadar Y. Pleurotus ostreatus manganese-dependent peroxidase silencing impairs decolourization of Orange II. Microb. Biotechnol. 2010;3:93–106.10.1111/mbt.2010.3.issue-1
- Dence CW. The determination of lignin. In: Lin SY, Dence CW, editors. Methods in lignin chemistry. Heidelberg: Springer-Verlag; 1992. p. 33–61.10.1007/978-3-642-74065-7
- Rowell RM, Pettersen R, Tshabalala MA. Cell wall chemistry. In: Rowell RM, editor. handbook of wood chemistry and wood composites. 2nd ed. New York, NY: CRC Press; 2013. p. 33–74.