Abstract
The mitochondrial citrate transport protein (CTP) functions as a malate–citrate shuttle catalyzing the exchange of citrate plus a proton for malate between mitochondria and cytosol across the inner mitochondrial membrane in higher eukaryotic organisms. In this study, for functional analysis, we cloned the gene encoding putative CTP (ctpA) of citric acid-producing Aspergillus niger WU-2223L. The gene ctpA encodes a polypeptide consisting 296 amino acids conserved active residues required for citrate transport function. Only in early-log phase, the ctpA disruptant DCTPA-1 showed growth delay, and the amount of citric acid produced by strain DCTPA-1 was smaller than that by parental strain WU-2223L. These results indicate that the CTPA affects growth and thereby citric acid metabolism of A. niger changes, especially in early-log phase, but not citric acid-producing period. This is the first report showing that disruption of ctpA causes changes of phenotypes in relation to citric acid production in A. niger.
Graphical abstract
Disruption of the gene encoding the citrate transport protein (CTPA, i.e., malate–citrate shuttle protein) affects the phenotype of Aspergillus niger.

Citric acid is an organic acid with a broad range of versatile applications. For example, citric acid is used as acidulant, antioxidant in food and beverage, chelating agent, buffer, and pH adjuster in industry.Citation1,2) Nowadays, citric acid is industrially produced by fermentation using the filamentous fungus Aspergillus niger, and the global production of citric acid reaches approximately 1.75 million tons/year in 2011.Citation2)
Many studies have been done from various viewpoints to explain the mechanism and machinery of citric acid production by A. niger.Citation3–6) Because citric acid is an intermediate of the tricarboxylic acid (TCA) cycle, the causal relationships between citric acid production and the activities of related enzymes in TCA cycle and glycolysis have been extensively studied.Citation6–13) On the other hand, the transport system exporting citric acid generated in mitochondria must be also a very important subject of research directly linked to citric acid production. Guebel and Torres predicted that the transport of citric acid from mitochondria is one of the major regulatory steps of citric acid production in A. niger using a metabolic flux balance model.Citation14) Moreover, de Jongh and Nielsen pointed out the importance of the organic acids transport system between mitochondria and cytosol by gene insertion.Citation15) However, there are only a few reports on exporting citric acid from the cytosol to the external medium,Citation16,17) and less is known about the transport system, especially the system for exporting citric acid from mitochondria to the cytosol.
As shown in Fig. , citrate transport protein (CTP) localized at inner mitochondrial membrane is widely distributed in eukaryotes, such as plants,Citation18) mammals,Citation19,20) and microorganisms.Citation21) In eukaryotic microorganisms, the CTP of Saccharomyces cerevisiae, designated as CTP1, has been intensively studied.Citation21–29) The CTP is an anion transporter belonging to a mitochondrial carrier family (MCF)Citation30) and catalyzes the exchange of the dibasic form of TCAs (citric acid, isocitric acid) for dicarboxylic acids (malic acid, succinic acid) or phosphoenolpyruvic acid.Citation31) The amino acid sequence of CTP1 containing 6 transmembrane domains (TMDs),Citation21) namely one of the common characteristics of MCF, and CTP1 is presumed to be a homodimer.Citation23) Moreover, the amino acid residues essential for dimer conformation (G115, G119, E122, and Q182), transport activity (R181 and R189), and binding citrate (K37, K83, K239 and R87, R276, R279) were identified by construction of CTP mutants using site-directed mutagenesis.Citation24,28,32,33)
Fig. 1. Metabolic pathway in relation to citric acid production in A. niger.
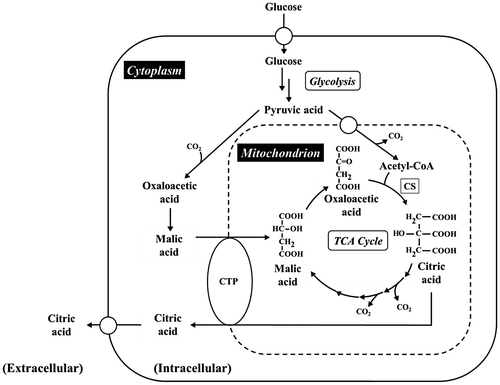
In A. niger CBS 513.88, the ancestor of currently used enzyme production strain, genomic analysis has been done, and on the genome, there are two annotated genes (An11g11230 and An18g00070) encoding putative CTP1 homologs.Citation34) The malate–citrate shuttle has been believed as the major transport system exporting citric acid generated in mitochondria.Citation3,35) However, functions of CTP and its genes from A. niger have not been examined so far. Identification and functional analysis of CTP and its genes in A. niger, especially citric acid-producing strain, are essential for explaining intricacies of the mechanism and machinery of citric acid production and application to efficient production of organic acid including citric acid. Recently, the gene encoding CTP1 homolog in A. kawachii, a filamentous fungus used for making Japanese distilled spirit shochu, has been identified and examined as a factor concerning citric acid accumulation in this fungus.Citation36)
In this study, for functional analysis, we cloned and identified the gene encoding CTP (ctpA) of citric acid-producing A. niger WU-2223L and constructed ctpA disruptants to characterize their phenotypes.
Materials and methods
Strains of A. niger and Escherichia coli, cultivation conditions and media. A. niger WU-2223L,Citation6,11,13,37–42) a citric acid-producing strain was used as a parental strain which has been deposited in National Institute of Technology and Evaluation (NITE) Biological Resource Center (NBRC) (accession number NBRC 111403; http://www.nite.go.jp/). As described later, strain DCTPA-1 (a ctpA disruptant strain) and cCTPA-1 (a strain DCTPA-1 complemented with ctpA) were used. Conidia of these strains were suspended in 60 mL of a synthetic medium to a final concentration of 5.0 × 106 conidia/mL and were cultivated aerobically with shaking at 120 rpm in 500-mL shake flasks at 30 °C. Citric acid production medium (SLZ medium) contained the following (per liter of pure water): glucose, 120 g; (NH4)2SO4, 3 g; K2HPO4, 1 g; KH2PO4, 1 g; MgSO4·7H2O, 0.5 g; MnSO4·5H2O, 0.014 g; FeCl3·6H2O, 0.01 g; and ZnCl2, 0.075 mg. The initial pH was adjusted to 3.0. Unless otherwise indicated, 2% (v/v) methanol was added as a promotive substance for citric acid production.Citation6) For isolation and maintenance or growth test of the transformants of A. niger, Czapek-Dox (CD) medium was used as a minimal medium, and it contained the following (per liter of distilled water): glucose, 30 g; NaNO3, 2 g; K2HPO4, 1 g; MgSO4·7H2O, 0.5 g; KCl, 0.5 g; FeSO4·7H2O, 0.01 g; and 0.7 M KCl, added as osmostabilizer for protoplasts. The initial pH was adjusted to 6.0, and when necessary, 10 g/L of agar BA-10 (Ina Food Industry, Nagano, Japan) was added to prepare agar plates of CD medium. E. coli JM109 cells were used to amplify the plasmids described later. The recombinant E. coli cells harboring the plasmids were cultivated in 100-mL Erlenmeyer flask containing 20 mL of Luria-Bertani (LB) medium with 100 mg/mL of ampicillin at 37 °C for 16 h at 120 rpm.
DNA and RNA isolation
Genomic DNA and total RNA were isolated basically according to the methods reported previously.Citation37–39) After cultivation for appropriate intervals, mycelia were harvested using a glass microfiber filter GF/A and then qualitative paper filter no. 4 (Whatman, Brentford, UK). The mycelia harvested were washed twice with pure water on the paper filter. The washed mycelia were frozen in liquid nitrogen and ground into fine powder with a mortar and pestle. Chromosomal DNA was isolated from the powdered mycelia using ISOPLANT II (Nippon Gene, Tokyo) in accordance with the manufacturer’s manual. Total RNA was isolated from the powdered mycelia using the guanidinium thiocyanate–phenol–chloroform extraction method,Citation43) with some modifications.Citation37–39)
Cloning of CTP homolog genes
To amplify the gene encoding CTP1 homologs (ctpA and ctpB) by PCR, genomic DNA of A. niger WU-2223L was used as the template. The specific primers were designed in accordance with the sequence of the gene encoding CTP1 homolog reported for A. niger CBS 513.88 (An11g11230 and An18g00070).Citation34) To amplify ctpA, the Pr1 primer (5′- ATG ACT CCT AGT GCT GAG CG -3′) and Pr2 primer (5′- TCA AAT GTA TCG CCT TTC CGG ATC -3′) were used. The cDNA fragment of ctpA was also amplified by reverse-transcription PCR using Pr1 and Pr2 primers. Reverse-transcription PCR was performed using ReverTra-Plus (Toyobo, Osaka, Japan) in accordance with the manufacturer’s manual. The flanking region of ctpA in A. niger WU-2223L was amplified as follows. Chromosomal DNA of A. niger WU-2223L was digested with Hind III, Sac I, or Sac II (Nippon Gene, Tokyo, Japan), and the digested DNA fragment was self-ligated using T4 DNA ligase (Takara Bio, Otsu, Japan) at 16 °C for 16 h. Subsequently, the self-ligated DNA was used as the template for inverse PCR using the Pr3 primer (5′- CTT CAA CCT CTC CCC GGG TCT TT -3′) and Pr4 primer (5′- GAG TCC ATA GAG TAC AGA GGG TTC C -3′). The amplified 3,806-bp fragment was cloned into pGEM-T Easy vector (Promega, Tokyo, Japan) for construction of a plasmid pGANCTPAgeno, sequenced and used as ctpA and its flanking regions. The other CTP1 homolog gene, ctpB, was also amplified using Pr5 primer (5′- ATG GCT ACC CTT GCC TCC TCA AC-3′) and Pr6 primer (5′- TCA TTC AAC CCC AGC CCT CG-3′) and sequenced.
Transcriptional analyses by reverse-transcription PCR (RT-PCR)
Transcriptional levels of ctpA and ctpB in A. niger WU-2223L and its derivatives, DCTPA-1 and cCTPA-1, were analyzed by reverse-transcription PCR (RT-PCR). The gene encoding the cytoskeleton protein actin, i.e., act1 was used as internal standard. The cDNAs were synthesized using total RNA isolated by PrimeScript 1st Strand cDNA Synthesis Kit (Takara Bio, Shiga, Japan) according to the manufacture’s protocol. The genes analyzed and specific primers for these genes were listed in Table .
Table 1. Primers used for transcriptional analyses.
Construction of plasmids for ctpA disruption and complementation of ctpA disruption with Saccharomyces cerevisiae ctp1
The plasmid pDCTPA-PTR for ctpA disruption was constructed as follows. The plasmid pGANCTPAgeno harboring genomic DNA fragment of ctpA and its flanking region were digested with Nhe I and deleted a part of ctpA containing 5th–8th exon to construct the plasmid pGDANCTPA. Subsequently, pGDANCTPA was digested with BssH II, and the pyrithiamine resistance gene ptrA, amplified from pPTRI (Takara Bio) using Pr7 primer (5′- TTA GCG CGC GGG CAA TTG ATT ACG -3′) and Pr8 primer (5′- TTA GCG CGC ATG GGG TGA CGA TGA -3′), was inserted into the BssH II site of ctpA in pGDANCTPA to construct the plasmid pDCTPA-PTR.
The plasmid pSCCTP1 for complementation of ctpA disruption with S. cerevisiae ctp1 was constructed as follows. The DNA fragments of upstream region of ctpA amplified using Pr9 primer (5′- GTT GAA ATA CTC CCG ACA TGC CTC -3′) and Pr10 primer (5′- TAG CTT TAC TGG ACA TTG CCA GGA TAT CTG CT -3′), the downstream region of ctpA amplified using Pr11 primer (5′- AGT TAT GCT AGC CTG ATG GGG AGC ACT TTG G -3′) and Pr12 primer (5′- AGG CTT GGC AGG TTT TAC GG -3′), and cDNA of S. cerevisiae ctp1 amplified using Pr13 primer (5′- AGC AGA TAT CCT GGC AAT GTC CAG TAA AGC TA-3′) and Pr14 primer (5′- CCA AAG TGC TCC CCA TCA GGC TAG CAT AAC T-3′) were joined by fusion-PCR, and resulting fragment was inserted into the Hinc II site of plasmid pUC19 (Takara Bio) to construct the plasmid pSCCTP1.
Transformation of A. niger for disruption and complementation of ctpA
Transformation of A. niger WU-2223L and its derivative DCTPA-1 was performed by the protoplast–PEG method, as described previously.Citation40) The ctpA disruptants derived from A. niger WU-2223L were constructed by introduction of pDCTPA-PTR. The transformants were isolated on CD medium containing 100 μg/mL pyrithiamine as the selection marker.
The ctpA disruptants complemented with ctpA were constructed by introduction of pGANCTPAgeno into A. niger DCTPA-1 through cotransformation with the plasmid pAN7-1Citation44) harboring hygromycin B resistance gene hph. The transformants were isolated on CD medium containing 100 μg/mL hygromycin B as the selection marker.
The ctpA disruptant DCTPA-1 was complemented with S. cerevisiae ctp1 by introduction of pSCCTP1C into A. niger DCTPA-1 through cotransformation with the plasmid pAN7-1Citation44) harboring hygromycin B resistance gene hph. The transformants were isolated on CD medium containing 100 μg/mL hygromycin B as the selection marker. As for the hygromycin B-resistant transformants, introduction of S. cerevisiae ctp1 was confirmed by PCR using Pr13 and Pr14 primers.
Southern blot analysis
Southern blot analysis was performed as described previously.Citation39,41) Approximately, 500-bp fragment of PCR-amplified ctpA with genomic DNA of A. niger WU-2223L as a template using primer Pr15 (5′- CTT CAA CCT CTC CCG GGT CTT T- 3′) and P16 (5′- GAG TCC ATA GAG TAC AGA GGG TTC C-3′) was used as the probe for Southern blot analysis.
Growth tests on the ctpA disruptant
For growth tests, CD agar plate or modified CD agar plates containing 30 g/L organic acid (citric acid, malic acid, succinic acid and so on), as an alternative to glucose were used. Conidia of A. niger WU-2223L, DCTPA-1, and cCTPA-1 were suspended in distilled water to a final concentration of 2.0 × 108 conidia/mL. Aliquots of 2.5 μL of conidial suspension were inoculated on each of the modified CD agar plates and were incubated for 3 or 5 days at 25, 30, or 35 °C. After appropriate cultivation times, the growth and morphology of each strain were analyzed. Moreover, each strain in submerged culture under all the conditions tested was observed by microscope, an Olympus System Microscope BX51 (Olympus, Tokyo, Japan).
Measurement of intracellular organic acids
Measurement of intracellular organic acids from the mycelia of A. niger strains and calculation of the intracellular volume were performed according to the methods described previously.Citation11)
Determination of mycelial dry weight, citric acid, and glucose in the culture broth
Mycelial dry weights, citric acid, and glucose in the culture broth were determined basically according to the methods described previously.Citation11) The culture filtrate was recovered by filtration of the culture broth through glass microfiber filter GF/A and qualitative paper filter no. 4. Residual mycelia on the filter paper were dried at 105 °C, and the mycelial dry weight was determined as described previously.Citation42) On the other hand, culture filtrate containing citric acid and glucose was analyzed by the HPLC LC-20 system (Shimadzu) equipped with a Shim-pack SCR-101H column (Shimadzu). For quantification, refractive index detector RID-10A and photodiode array detector SPD-M20A at 210 nm (Shimadzu) were used, and 4 mM HClO4 was used as a mobile phase.
Results
Cloning and identification of ctpA and its amino acid sequences
Genomic DNA and total RNA were isolated from A. niger WU-2223L cultivated in SLZ medium for 3 days, and a chromosomal DNA fragment of ctpA with its flanking region (3,806 bp) was cloned by PCR and inverse PCR. As shown in Fig. , a transcript of ctpA was clearly detected, and cDNA of ctpA (888 bp) was also cloned by RT-PCR. As shown in Fig. S1, the nucleotide sequences of chromosomal ctpA and its cDNA were determined and analyzed (GenBank accession no. LC061596). The ctpA of A. niger WU-2223L occupied a 1,423-bp region containing eight introns and encoded a 32.5-kDa protein (CTPA) consisting 296 amino acids. The CpG island exists partially overlapping with the putative promoter region of ctpA (−47 bp). By Southern blot analysis using a 3,806-bp fragment of ctpA as a probe, we also confirmed that there was only one copy of ctpA on the A. niger WU-2223L genome (Fig. (B)).
Fig. 2. Amplifications of CTP1 homolog genes by PCR and reverse-transcription PCR.
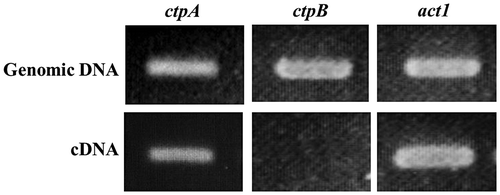
As shown in Fig. , the amino acid sequence of CTPA shows 47% homology to CTP derived from S. cerevisiae S288c, i.e., CTP1 (GenBank accession no. NC_001134), and CTPA contains six TMD, namely one of common characteristics of MCF. Moreover, the four amino acid residues required for the formation of a dimer interfaceCitation32,33) are conserved in the amino acid sequence of CTPA as G108, G112, E115, and Q171. Similarly, two amino acid residues required for transport function, i.e., R181 and R189Citation24) and two citrate binding sites (K37, K83, and K239) and (K87, R276, and R279)Citation28) are conserved in the amino acid sequence of CTPA (Fig. ).
Fig. 3. Amino acid sequence alignment of CTPs derived from A. niger WU-2223L (CTPA) and S. cerevisiae S288c (CTP1).
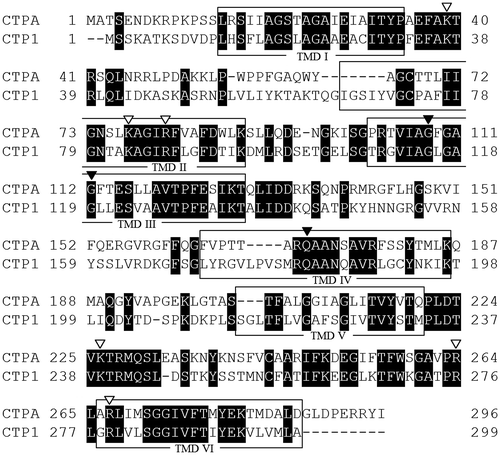
Another gene encoding putative CTP of A. niger was tentatively termed ctpB and also sequenced (Fig. S2; we have already deposited the sequences to DDBJ, and GenBank accession number will be soon available). However, no transcript of ctpB was detected under several conditions tested, including the ones for citric acid production, as shown in Fig. (described later). It is noteworthy that no conserved amino acid residues required for transport functionCitation24) are found in the deduced amino acid sequence of CTPB (Fig. S2).
Transcriptional analysis of ctpA and ctpB
Transcriptional analysis of ctpA and ctpB was performed by RT-PCR using cDNA of these genes synthesized from total RNA isolated from mycelia of strain WU-2223L cultivated under the conditions of citric acid production for 3 days. Only the transcript of ctpA was detected during the whole period of cultivation (Figs. and S3). In contrast, no transcription of ctpB was detected, under all the cultivation conditions tested, including those with different media and/or different carbon sources instead of glucose (details not shown).
Construction of ctpA disruptants and complementation of ctpA in the disruptant
The ctpA disruptants derived from A. niger WU-2223L were constructed using pDCTPA-PTR by the protoplast–PEG method, as shown in Fig. (A). Ninety-eight transformants were isolated on CD medium containing 100 μg/mL pyrithiamine as the selection marker. As shown in Fig. (B), the ctpA disruption through homologous recombination was confirmed by Southern blot analysis using their genomic DNA digested by Hind III and ctpA fragment as the specific probe. Moreover, the gene disruption was clearly shown by transcriptional analysis of ctpA (Fig. (C)). These analyses revealed that three of the 98 transformants retained the predicted gene. We then selected one representative ctpA disruptant, termed DCTPA-1, and used it for subsequent analyses. In addition, we confirmed that other two transformants showed similar phenotypes to that of the strain DCTPA-1.
Fig. 4. Disruption of ctpA in A. niger WU-2223L.
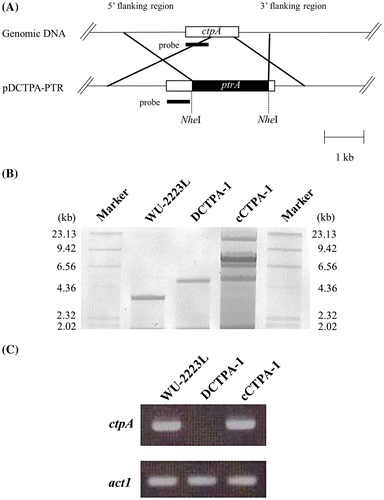
Complementation of ctpA in ctpA disruptant, strain DCTPA-1, was performed by cotransformation with the plasmids pGANCTPAgeno and pAN7-1 containing hygromycin B resistance gene.Citation44) Transformants were isolated on CD medium containing 100 μg/mL hygromycin B as the selection marker. The complementation of ctpA was confirmed by Southern blot analysis and transcriptional analysis of ctpA (Fig. (B) and (C)). These analyses revealed that three transformants retained several copies of ctpA functioning, and numbers of copies of ctpA were revealed to be 3–5 for these transformants, based on PCR analysis (details not shown). Among these complemented transformants, one representative strain termed cCTPA-1 retaining 3 copies of ctpA was used for further analyses. Phenotypes of cCTPA-1, different from those of DCTPA-1, are described later.
Complementation of S. cerevisiae ctp1 in the ctpA disruptant
We constructed several transformants containing cDNA of S. cerevisiae ctp1 (an open reading frame, GenBank accession no. NC_00134) under the control of A. niger WU-2223L ctpA promoter and terminator using strain DCTPA-1 as a host of ctpA disruptant. Complementation of S. cerevisiae ctp1 in ctpA disruptant, strain DCTPA-1, was also performed by cotransformation with the plasmids pSCCTP1P and pAN7-1 containing hygromycin B resistance gene.Citation44) Transformants were isolated on CD medium containing 100 μg/mL hygromycin B as the selection marker. Among 20 hygromycin B-resistant transformants, 3 transformants were confirmed to harbor pSCCTP1C, and S. cerevisiae ctp1 was detected on the genomic DNA of transformants by PCR. One representative transformant, cCTP1-SC1, retaining one S. cerevisiae ctp1 on the genome showed similar phenotypes of strains WU-2223L and cCTPA-1, but not those of DCTPA-1 (Kirimura et al., unpublished results). Thus, it was confirmed that S. cerevisiae ctp1 complemented the defect of A. niger ctpA.
Effects of disruption of ctpA on the fungal growth
To examine the effects of ctpA disruption on the fungal growth, we performed growth test on A. niger WU-2223L, DCTPA-1, and cCTPA-1 cultivated under various conditions. As shown in Fig. (A), clear difference in the growth was observed for strain DCTPA-1 cultivated on CD agar plate containing 30 g/L glucose as a carbon source at 30 °C (usual cultivation temperature) or 25 °C, and DCTPA-1 showed growth delay compared with that of strain WU-2223L. Strain DCTPA-1 cultivated on SLZ agar plate at 30 °C also showed growth delay compared with that of strain WU-2223L (data not shown). Moreover, as shown in Fig. (B), the number of ungerminated spores of strain DCTPA-1 was greater than that of strain WU-2223L under the shake culture conditions at 30 °C for 24 h. Almost all (100%) spores of strain WU-2223L and cCTPA-1 germinated from the beginning (0 h) to 9 h of cultivation, but germination percentage of strain DCTPA-1 was only 23.7% at 9 h. These results concerning the defect in growth in the early-log phase are in accord with the time-dependent transcriptional level change of ctpA. Transcriptional levels of ctpA in the early-log phase were high during the whole-cultivation period (Fig. S3). All of these results clearly indicate that disruption of ctpA causes changes of phenotypes containing growth and germination rates in strain DCTPA-1.
Fig. 5. Phenotypic characterization of a ctpA disruptant.
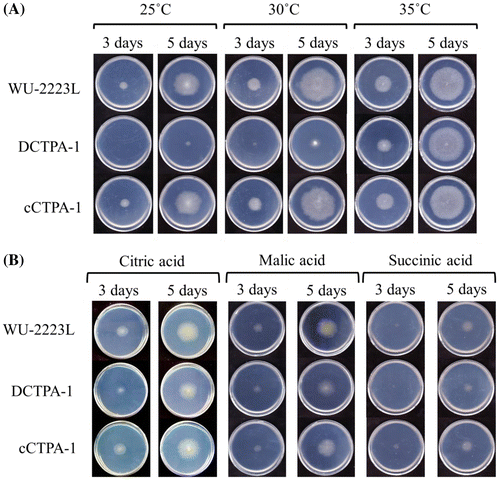
Fig. 6. A, Photomicrographs of A. niger WU-2223L and DCTPA-1.
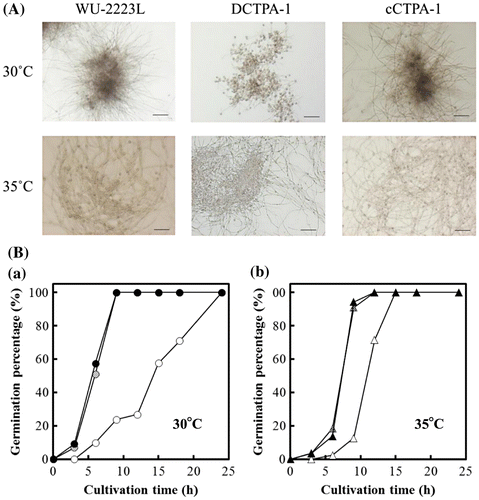
It is interesting to note that strain DCTPA-1 cultivated at 35 °C showed no growth delay, as shown in Fig. (A), and no difference in mycelial dry weight compared with that of strain WU-2223L, as shown in Fig. . Under the shake culture conditions at 35 °C for 24 h, the rate of ungerminated spore of strain DCTPA-1 decreased, as shown in Fig. . We consider that the growth delay on CD agar plates shown in Fig. must have been in relation to the germination delay as observed for Fig. . Moreover, as shown in Fig. (B), on CD agar plates containing organic acids such as citric acid, malic acid, and succinic acid linked to TCA cycle, as the sole carbon source instead of glucose, there was no difference in growth between strain DCTPA-1 and WU-2223L.
Fig. 7. Dry cell weight of A. niger WU-2223L (solid), DCTPA-1 (hollow), and cCTPA-1 (shaded). The strains were cultivated under the conditions of citric acid production for 3 days at 30 (circle) or 35 °C (triangle), 120 rpm.
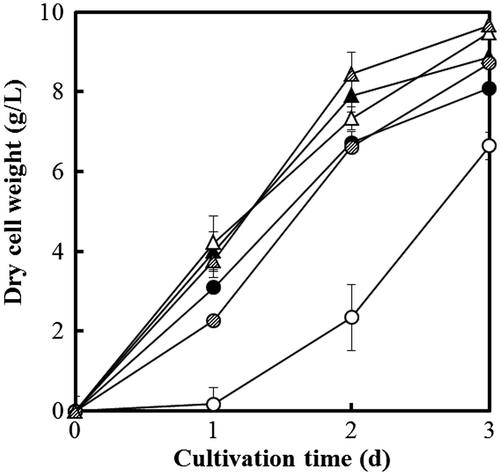
As shown in Figs. and , growth of strain cCTPA-1, a representative strain from DCTPA-1 complemented with the gene ctpA, was similar to that of strain WU-2223L, but different from that of strain DCTPA-1, under all the cultivation conditions tested. These results clearly indicate that the phenotypic changes found in the ctpA disruptants are intimately tied to the functionality of the gene.
Effects of disruption of ctpA on citric acid production
To confirm the contribution of CTPA to citric acid production in A. niger, strain WU-2223L, DCTPA-1, and cCTPA-1 were cultivated in SLZ medium for citric acid production for 12 days. As shown in Fig. , the amount of citric acid produced (62.8 g/L) and glucose consumed (114 g/L) by strain DCTPA-1 on the 12th day of cultivation were almost the same levels of those by strain WU-2223L (63.0 and 116 g/L). Moreover, mycelial dry weight of strain DCTPA-1 on the 12th day of cultivation (15.5 g/L) and that of strain WU-2223L (14.9 g/L) were not substantially different from each other. Citric acid productivity, defined as the ratio of amount of citric acid per mycelial dry weight, for DCTPA-1 (4.0 g/g) was also the same level of that for WU-2223L (4.2 g/g). However, the amounts of citric acid produced and glucose consumed by strain DCTPA-1 during the period from the start to 7th day of cultivation were smaller than those by strain WU-2223L. Especially, on the 3rd day of cultivation, the amount of citric acid produced by strain DCTPA-1 (9.22 g/l) was 38.9% (3.58 g/L) of that by strain WU-2223L. The concentrations of other organic acids in the culture filtrate of strain DCTPA-1 were of approximately the same level as those of strain WU-2223L. Moreover, the concentrations of intracellular organic acids in the TCA cycle, such as succinic acid, fumaric acid, and malic acid, of each strain were also analyzed, but no significant accumulation of the organic acids was confirmed.
Fig. 8. Citric acid production (triangle) and glucose consumption (circle) by A. niger WU-2223L (solid), DCTPA-1 (hollow), and cCTPA-1 (shaded) (A) and citric acid productivities for each strain (B).
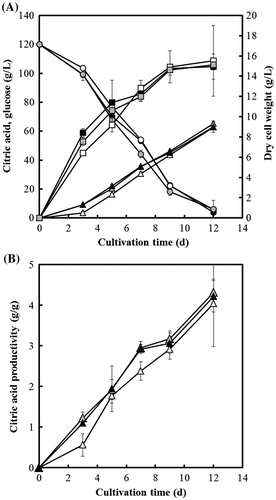
On the other hand, during the whole 12-day cultivation period, the amounts of citric acid produced and glucose consumed by strain cCTPA-1 were the same levels of those by strain WU-2223L. Moreover, both mycelial dry weight (14.2 g/L) and citric acid productivity (4.6 g/g) of strain cCTPA-1 on the 12th day of cultivation were almost the same levels of those by strain WU-2223L. These results indicate that the defect of ctpA causes the effects on the growth and citric acid production of A. niger, and that the phenotypic changes caused by such effects appear to be prominent especially in the early-log phase of cultivation.
Discussion
In this study, for functional analysis of CTP homolog and its gene derived from citric acid-producing A. niger WU-2223L, we cloned ctpA and constructed ctpA disruptants to characterize their phenotypes.
As shown in Figs. , disruption of ctpA caused changes of phenotypes in relation to growth and citric acid production, especially in early-log phase, contrasting quite sharply with the finding that disruption of the gene encoding CTP1 in S. cerevisiae causes no effect on the wild-type phenotype.Citation22) This discrepancy may be explained if the budding yeast could have an alternative CTP gene whose product could supplement the function of the disrupted or mutated CTP1 gene product, while A. niger may have no such a functional substitute. It remains unclear why growth and germination delay was caused by disruption of ctpA, but it seems likely that the disruption of ctpA altered the distribution of organic acids in the intracellular fraction(s) including mitochondria some resulting effects on growth and germination thorough metabolic pathway (Fig. ). For growth and germination, formation of mycelia accompanying syntheses of cell membrane and cell wall is necessary.Citation45) Therefore, there is some possibility that an insufficient supply of citric acid, i.e., a starting substrate for biosynthesis of fatty acids essential for synthesis of cell membrane, from mitochondria caused by defect of CTPA results in growth and germination delay. We confirmed that growth of strain DCTPA-1 cultivated on minimal agar plate containing organic acid, such as citric acid, malic acid, and succinic acid, as a sole carbon source was the same level as that of strain WU-2223L (Fig. ). These results could support the idea that due to the disruption of ctpA, the confusion in relation to transport and metabolism of organic acids caused strong effects on growth of A. niger, especially in early-log phase.
On the other hand, strain DCTPA-1 cultivated even at 35 °C (higher than usual cultivation temperature) showed no growth delay (Fig. (A)). Consumption of glucose and accumulation of extracellular citric acid are well inversely correlated (Fig. ). However, the rate of growth (dry cell weight) slows down with time of cultivation even on 3rd day besides strain DCTPA-1 grown at 30 °C (Fig. ). The defect in growth of strain DCTPA-1 at 30 °C appears to be almost rescued by the cultivation at 35 °C. The phenotypic defect that strain DCTPA-1 showed at 30 °C appears to be rescued at 35 °C or by long period of incubation, but no ctpA transcript can be detected in strain DCTPA-1 grown at any temperatures (data not shown). Moreover, KovatsCitation46) and Noguchi and AraiCitation47) reported that the optimal temperatures for citric acid fermentation were different for each cultivation phase, and that in their reportsCitation46,47) maximum citric acid production was obtained when the cultivation temperature was kept at 28 °C for the initial stage of cultivation and then reduced to lower temperature (20 or 25 °C). These known results agreed well with the possibility that activities of transporters for organic acids including citric acid might be different at each cultivation temperature, and that CTPA might contribute citric acid production at around 30 °C due to high activity.
In this study, we confirmed the contribution of CTPA encoded by ctpA to germination of conidia and mycelial yield in the early cultivation stage. As shown in Fig. , the amounts of citric acid produced and glucose consumed by strain DCTPA-1 from the start to 7th day of cultivation were smaller than those by strain WU-2223L. In particular, on the 3rd day of cultivation, the amount of citric acid produced by strain DCTPA-1 (9.22 g/l) was 38.9% (3.58 g/L) of that by strain WU-2223L. In this connection, it is interesting to note the report that expression of malate dehydrogenase gene from S. cerevisiae in A. niger led to an increase in the initial citric acid production rate.Citation15) Our results in this study also support the idea that the balance of citrate and malate affects the citric acid production rate especially in the early-log phase. Moreover, disruption of the gene ctpA gives slight effect on the final amount of citric acid produced (12th day in Fig. ). Therefore, we consider the possibility that other transporter(s), but not CTPA, have important roles for hyper production of citric acid by A. niger.
In conclusion, this study, for the first time, shows the relationship between CTPA, as the putative transporter catalyzing the exchange of citrate between mitochondria and cytosol in A. niger, and growth of A. niger in relation to citric acid production by genetic engineering. Since we have already confirmed that several gene encoding putative transporters of organic acids are transcribed in A. niger WU-2223L under several cultivation conditions, we have started series of studies on the functions of such genes in relation to citric acid production.
Authors contribution
K. K. 1 designed this study; K. K. 2, Y. U, and T. H. performed the experiments; K. K. 1, K. K. 2, Y. U., and T. H. analyzed the data; K. K. 1 and K. K. 2 wrote the manuscript.
Disclosure statement
No potential conflict of interest was reported by the authors.
Supplemental materials
The supplemental material for this paper is available at http://dx.doi.org/10.1080/09168451.2016.1164583.
TBBB_1164583_Supplementary_Material.pdf
Download PDF (425.5 KB)TBBB_1164583_Supplementary_Material.zip
Download Zip (383.5 KB)Acknowledgment
We wish to express our thanks to Dr M. Suzuki for helpful discussion.
Additional information
Funding
Notes
Abbreviations: CD medium, Czapek-Dox medium; CTP, citrate transport protein; LB medium; Luria-Bertani medium; MCF, mitochondrial carrier family; RT-PCR, reverse-transcription PCR; TCA, tricarboxylic acid; TMD, transmembrane domain.
References
- Dhillon GS, Brar SK, Verma M, et al. Recent advances in citric acid bio-production and recovery. Food Bioprocess Technol. 2011;4:505–529.10.1007/s11947-010-0399-0
- Demain AL, Sanchez S. Microbial synthesis of primary metabolites: current trends and future prospects. In: El-Mansi EMT, Bryce CFA, Dahhou B, Sanchez S, Demain AL, editors. Fermentation microbiology and biotechnology. Boca Raton: CRC Press; 2011. p. 77–100.10.1201/b11490
- Karaffa L, Kubicek CP. Aspergillus niger citric acid accumulation: do we understand this well working black box? Appl. Microbiol. Biotechnol. 2003;61:189–196.10.1007/s00253-002-1201-7
- Kirimura K, Honda Y, Hattori T. Bio-based chemicals, citric acid. In: Murray MY, editor. Comprehensive biotechnology. London: Elsevier; 2011. p. 135–142.10.1016/B978-0-08-088504-9.00169-0
- Magnuson JK, Lasure LL. Organic acid production by filamentous fungi. In: Tkacz JS, Lange L, editors. Advance in fungal biotechnology for industry, agriculture, and medicine. New York, NY: Kluwer Academic/Plenum Publishers; 2004. p. 307–340.10.1007/978-1-4419-8859-1
- Usami S. Production of citric acid by submerged culture. Mem. Sch. Sci. Eng. Waseda. Univ. 1978;42:17–26.
- Arts E, Kubicek CP, Röhr M. Regulation of phosphofructokinase from Aspergillus niger: effect of fructose-2,6-biphosphate on the action of citrate, ammonium ions and AMP. J. Gen. Microbiol. 1987;133:1195–1199.
- Harmsen HJM, Kubicek-Pranz EM, Röhr M, et al. Regulation of 6-phosphofructo-2-kinase from the citric-acid-accumulating fungus Aspergillus niger. Appl. Microbiol. Biotechnol. 1992;37:784–788.
- Ruijter GJ, Panneman H, Visser J. Overexpression of phosphofructokinase and pyruvate kinase in citric acid-producing Aspergillus niger. Biochim. Biophys. Acta. 1997;1334:317–326.10.1016/S0304-4165(96)00110-9
- Ruijter GJ, Panneman H, Xu D, et al. Properties of Aspergillus niger citrate synthase and effects of citA overexpression on citric acid production. FEMS Microbiol. Lett. 2000;184:35–40.10.1111/fml.2000.184.issue-1
- Kobayashi K, Hattori T, Hayashi R, et al. Overexpression of the NADP+-specific isocitrate dehydrogenase gene (icdA) in citric acid-producing Aspergillus niger WU-2223L. Biosci. Biotechnol. Biochem. 2014;78:1246–1253.10.1080/09168451.2014.918483
- Zehentgruber O, Kubicek CP, Röhr M. Alternative respiration of Aspergillus niger. FEMS Microbiol. Lett. 1980;8:71–74.10.1111/fml.1980.8.issue-2
- Kirimura K, Hirowatari Y, Usami S. Alternations of respiration systems in Aspergillus niger under the conditions of citric acid fermentation. Agric. Biol. Chem. 1987;51:1299–1303.10.1271/bbb1961.51.1299
- Guebel DV, Darias NVT. Optimization of citric acid production by Aspergillus niger through a metabolic flux balance model. Electron. J. Biotechnol. 2001;4:1–17.
- de Jongh WA, Nielsen J. Enhanced citrate production through gene insertion in Aspergillus niger. Metab. Eng. 2008;10:87–96.10.1016/j.ymben.2007.11.002
- Kontopidis G, Mattey M, Kristiansen B. Citrate transport during the citric acid fermentation by Aspergillus niger. Biotechnol. Lett. 1995;17:1101–1106.10.1007/BF00143110
- Netik A, Torres NV, Riol JM, et al. Uptake and export of citric acid by Aspergillus niger is reciprocally regulated by manganese ions. Biochim. Biophys. Acta. 1997;1326:287–294.10.1016/S0005-2736(97)00032-1
- Laloi M. Plant mitochondrial carriers: an overview. Cell. Mol. Life. Sci. 1999;56:918–944.10.1007/s000180050484
- Bisaccia F, De Palma A, Palmieri F. Identification and purification of the tricarboxylate carrier from rat liver mitochondria. Biochim. Biophys. Acta. 1989;977:171–176.10.1016/S0005-2728(89)80068-4
- Claeys D, Azzi A. Tricarboxylate carrier of bovine liver mitochondria. J. Biol. Chem. 1989;264:14627–14630.
- Kaplan RS, Mayor JA, Gremse DA, et al. High level expression and characterization of the mitochondrial citrate transport protein from the yeast Saccharomyces cerevisiae. J. Biol. Chem. 1995;270:4108–4114.
- Kaplan RS, Mayor JA, Kakhniashvili D, et al. Deletion of the nuclear gene encoding the mitochondrial citrate transport protein from Saccharomyces cerevisiae. Biochem. Biophys. Res. Commun. 1996;226:657–662.10.1006/bbrc.1996.1411
- Kotaria R, Mayor JA, Walters DE, et al. Oligomeric state of wild-type and cysteine-less yeast mitochondrial citrate transport proteins. J. Bioenerg. Biomembr. 1999;31:543–549.10.1023/A:1005460810527
- Xu Y, Kakhniashvili DA, Gremse DA, et al. The yeast mitochondrial citrate transport protein. Probing the roles of cysteines, Arg(181), and Arg(189) in transporter function. J. Biol. Chem. 2000;275:7117–7124.10.1074/jbc.275.10.7117
- Kaplan RS, Mayor JA, Kotaria R, et al. The yeast mitochondrial citrate transport protein: determination of secondary structure and solvent accessibility of transmembrane domain IV using site-directed spin labeling. Biochemistry. 2000;39:9157–9163.10.1021/bi000433e
- Ma C, Kotaria R, Mayor JA, et al. The yeast mitochondrial citrate transport protein: characterization of transmembrane domain III residue involvement in substrate translocation. J. Biol. Chem. 2005;280:2331–2340.
- Ma C, Remani S, Kotaria R, et al. The mitochondrial citrate transport protein: evidence for a steric interaction between glutamine 182 and leucine 120 and its relationship to the substrate translocation pathway and identification of other mechanistically essential residues. Biochim. Biophys. Acta. 2006;1757:1271–1276.10.1016/j.bbabio.2006.06.011
- Ma C, Remani S, Sun J, et al. Identification of the substrate binding sites within the yeast mitochondrial citrate transport protein. J. Biol. Chem. 2007;282:17210–17220.10.1074/jbc.M611268200
- Aluvila S, Kotaria R, Sun J, et al. The yeast mitochondrial citrate transport protein: molecular determinants of its substrate specificity. J. Biol. Chem. 2010;285:27314–27326.10.1074/jbc.M110.137364
- Kaplan RS, Mayor JA, Wood DO. The mitochondrial tricarboxylate transport protein. cDNA cloning, primary structure, and comparison with other mitochondrial transport proteins. J. Biol. Chem. 1993;268:13682–13690.
- Palmieri F, Stipani I, Quagliariello E, et al. Kinetic study of the tricarboxylate carrier in rat liver mitochondria. Eur. J. Biochem. 1972;26:587–594.10.1111/ejb.1972.26.issue-4
- Ma C, Kotaria R, Mayor JA, et al. The mitochondrial citrate transport protein: probing the secondary structure of transmembrane domain III, identification of residues that likely comprise a portion of the citrate transport pathway, and development of a model for the putative TMDIII-TMDIII’ interface. J. Biol. Chem. 2004;279:1533–1540.10.1074/jbc.M310866200
- Walters DE, Kaplan RS. Homology-modeled structure of the yeast mitochondrial citrate transport protein. Biophys. J. 2004;87:907–911.10.1529/biophysj.104.042127
- Pel HJ, de Winde JH, Archer DB, et al. Genome sequencing and analysis of the versatile cell factory Aspergillus niger CBS 513.88. Nat. Biotechnol. 2007;25:221–231.10.1038/nbt1282
- Ruijter G.JG, Kubicek CP, Visser J. Production of organic acids by fungi. In: Osiewacz HD, editor. Industrial Applications. Berlin, Heidellberg: Springer; 2002. p. 213.10.1007/978-3-662-10378-4
- Futagami T, Mori K, Wada S, et al. Transcriptomic analysis of temperature responses of Aspergillus kawachii during barley koji production. Appl. Environ. Microbiol. 2015;81:1353–1363.10.1128/AEM.03483-14
- Kirimura K, Yoda M, Usami S. Cloning and expression of the cDNA encoding an alternative oxidase gene from Aspergillus niger WU-2223L. Curr. Genet. 1999;34:472–477.10.1007/s002940050422
- Kirimura K, Yoda M, Ko I, et al. Cloning and sequencing of the chromosomal DNA and cDNA encoding the mitochondrial citrate synthase of Aspergillus niger WU-2223L. J. Biosci. Bioeng. 1999;88:237–243.10.1016/S1389-1723(00)80003-1
- Kirimura K, Yoda M, Kumatani M, et al. Cloning and expression of Aspergillus niger icdA gene encoding mitochondrial NADP+-specific isocitrate dehydrogenase. J. Biosci. Bioeng. 2002;93:136–144.10.1016/S1389-1723(02)80005-6
- Kirimura K, Ogawa S, Hattori T, et al. Expression analysis of alternative oxidase gene (aox1) with enhanced green fluorescent protein as marker in citric acid-producing Aspergillus niger. J. Biosci. Bioeng. 2006;102:210–214.10.1263/jbb.102.210
- Hattori T, Kino K, Kirimura K. Regulation of alternative oxidase at the transcription stage in Aspergillus niger under the conditions of citric acid production. Curr. Microbiol. 2009;58:321–325.10.1007/s00284-009-9369-z
- Kirimura K, Nakajima I, Lee SP, et al. Citric acid production by the diploid strains of Aspergillus niger obtained by protoplast fusion. Appl. Microbiol. Biotechnol. 1988;27:504–506.10.1007/BF00451621
- Chomczynski P, Sacchi N. Single-step method of RNA isolation by acid guanidinium thiocyanate-phenol-chloroform extraction. Anal. Biochem. 1987;162:156–159.10.1016/0003-2697(87)90021-2
- Punt PJ, Oliver RP, Dingemanse MA, et al. Transformation of Aspergillus based on the hygromycin B resistance marker from Escherichia coli. Gene. 1987;56:117–124.10.1016/0378-1119(87)90164-8
- Mysyakina IS, Feofilova EP. The role of lipids in the morphogenetic processes of mycelial fungi. Microbiology. 2011;80:297–306.10.1134/S0026261711030155
- Kovats J. Influence of temperature on the production of citric acid by Aspergillus niger. Przemyl. Chem. 1946;2:122–128.
- Noguchi Y, Arao O. Citric Acid Fermentation (III): The Correlation between NH4NO3 concentration and fermentation temperature, and the effect of temperature changes on citric acid production. Hakkou Kogaku Zasshi. 1960;38:491–494. (in Japanese).