Abstract
Sword bean (Canavalia gladiata) seeds are a traditional food in Asian countries. In this study, we aimed to determine the optimal methods for the precipitation of sword bean proteins useful for the food development. The soaking time for sword beans was determined by comparing it with that for soybeans. Sword bean proteins were extracted from dried seeds in distilled water using novel methods. We found that most proteins could be precipitated by heating the extract at more than 90 °C. Interestingly, adding magnesium chloride to the extract at lower temperatures induced specific precipitation of a single protein with a molecular weight of approximately 48 kDa. The molecular weight and N-terminal sequence of the precipitated protein was identical to that of canavalin. These data suggested that canavalin was precipitated by the addition of magnesium chloride to the extract. Our results provide important insights into the production of processed foods from sword bean.
Graphical abstract

White sword beans (Canavalia gladiata) have traditionally been eaten in the Asian tropics and subtropicsCitation1,2) and are used as a source of Chinese medicines in many East Asian countries. Dried sword bean seeds were used in ancient times as a food or forage crop in the Southwestern United States, Mexico, and Central America countries.Citation3) Recently, sword bean seeds and pods have also been used to make tea in Japan. From an agricultural perspective, the average yield of sword beans is comparable to that of soybeans under optimal agricultural management conditions.Citation1) In addition, sword beans are relatively resistant to pests and diseases.Citation4) These applications and agricultural characteristics of sword beans suggest their potential use in the production of processed foods.
The crude protein content of sword beans is slightly lower than that of soybeans.Citation1,5–7) Thus, sword beans may be used as an alternative protein source after proper cooking and processing.Citation5) Interestingly, some recent animal experiments have suggested that consumption of sword bean seeds may prevent postmenopausal osteoporosisCitation8) and that extracts prepared with 50% ethanol inhibit alveolar bone resorption induced by Porphyromonas gingivalis infection.Citation9) Despite its nutritional, functional, and production potential, the seeds of sword beans have not been used for the production of processed foods as an alternative protein source, partly because our lack of understanding of the characteristics of sword bean proteins.
Some sword bean proteins have been analyzed in various biochemical studies. For example, proteins have been extracted from ground whole mature seeds in a high-concentration (0.15 M) sodium chloride solution.Citation10–14) However, because protein extracts containing high concentration of salts are inadequate for the production of processed foods, this method may not be applied for commercial purposes. Therefore, in order to use sword beans in the production of processed foods, novel methods are needed for the optimal extraction of sword bean proteins.
In this study, we established a novel method for the extraction of sword bean proteins from dried seeds in distilled water. In addition, we examined whether heating and the addition of magnesium chloride could be used to precipitate protein from crude extracts. This study provides important insights into the production of processed foods from sword beans and can thus enable sword beans to be used as potential alternative protein sources.
Materials and methods
Materials
Dried white sword beans and soybeans (cultivar Fukuyutaka) were purchased from Koyamaen (Hyogo, Japan) and Tomizawa Corporation (Tokyo, Japan), respectively. General chemical reagents were purchased from Wako Pure Chemical Industries Ltd. (Osaka, Japan).
Determination of soaking time
Soaking time was determined by measuring the bean size and absorbed water weight. Beans were soaked in 10 volumes (v/w) of distilled water at 20 °C for various durations. Bean size was measured with micrometer calipers (VC-15, AZONE, Osaka, Japan). The ratio was calculated by dividing the size of soaked beans by that of dried beans. The weight of beans was measured using an electronic balance (HR-120; A&D Company, Tokyo, Japan). The weight of the absorbed water was estimated by subtracting the initial weight of dried beans from that of soaked beans. The ratio was calculated by dividing the weight of the absorbed water by the initial weight of the dried beans. Data are represented as the average ± standard deviation of 5 beans.
Preparation of sword bean extracts
Soaked sword beans were ground in eight volumes (v/w) of distilled water with a hand blender (CSB-77JBSTRW, Cuisinart, CT, USA) on ice for 5 min. In a manipulation, which is generally used for making soymilk, a suspension containing ground beans was heated at 100 °C for 3 min and then separated into an extract (Extract A) and waste by squeezing with a cotton cloth. In another manipulation, the suspension was squeezed with the same cotton cloth to be separated into an extract (Extract B) and waste. Furthermore, Extract B was heated at 100 °C for 3 min and then separated into an extract (Extract C) and waste by squeezing with the same cotton cloth. The experimental scheme for the manipulation is shown in Supplemental Fig. 1.
Measurement of protein concentration
Protein concentrations were determined using the Bradford method with reagents from Bio-Rad Laboratories Inc. (CA, USA), using bovine serum albumin as a standard. Data are represented as the average ± standard deviation of three independent experiments.
Sodium dodecyl sulfate-polyacrylamide gel electrophoresis (SDS-PAGE)
SDS-PAGE was carried out with 12.5% polyacrylamide gels at a constant current of 12.5 mA for 2.5 h according to standard methods described by Laemmli.Citation15) Proteins were stained with 0.25% Coomassie Brilliant Blue R-250. Samples were mixed with 0.33 volumes of an SDS buffer (0.25 M Tris–HCl at pH 7.0 containing 4% SDS, 5% 2-mercaptoethanol, and 40% glycerol). Prior to SDS-PAGE, the samples were incubated in boiling water for 5 min. The molecular weight standard was purchased from Life Technologies Japan Ltd. (Tokyo, Japan).
Determination of the precipitation efficiency following heating
Extract B was separated into the supernatant and precipitate by centrifugation at 9,100 × g at 4 °C for 10 min. The supernatant was used as an initial sample for determining the precipitation efficiency. The sample was then heated at various temperatures for 20 min followed by incubation on ice for 5 min. The sample was separated into the supernatant and precipitate by centrifugation, as described for the preparation of the initial sample. Supernatant proteins were quantified as described above. The ratio of protein concentration was expressed as the percentage of the supernatant protein concentration of the heated sample to that of the initial sample. Wet precipitate weight was measured with an electronic balance (HR-120, A&D Company). The precipitation efficiency was expressed as the percentage of the weight of the wet precipitate to that of the initial sample. Data represent the average of three independent experiments.
Determination of the precipitation efficiency following addition of magnesium chloride
The initial sample was prepared as described above. The sample was incubated at 25, 50, 75, or 100 °C, respectively, for 5 min. Next, 0.11 volumes of 200 mM magnesium chloride or distilled water were added to the incubated sample while mixing, and the samples were then incubated again at the same temperature for 15 min. After heating, the mixtures were incubated on ice for 5 min and then separated into supernatants and precipitates by centrifugation at 9,100 × g for 20 min at 4 °C. The precipitation efficiency was expressed as the percentage of the wet weight to that of the initial sample. Supernatant protein concentrations were quantified as described above. The ratio of protein concentration was expressed as the percentage of the supernatant protein concentration of the sample with added magnesium chloride to that of the sample with distilled water only. Data represent the average of three independent experiments.
Analysis of the N-terminal amino acid sequence
After SDS-PAGE, proteins were transblotted onto polyvinylidene difluoride membranes (GE Healthcare Japan, Tokyo, Japan). The N-terminal amino acid sequence was determined with a protein sequenator (Procise 492, Life Technologies Japan Ltd).
Statistical analyses
A one-way analysis of variance and Bartlett test were used to compare the means among groups in the experiment for the protein concentration of the extract. A two-way analysis of variance and Bartlett test were used to compare the means among groups in the experiment for the effect of heating and adding magnesium chloride. A post hoc analysis was performed with the Tukey–Kramer test if the analysis of variance had revealed significance. Differences with p < 0.05 are considered to be significant in these statistical analyses.
Results
Size and weight of white sword beans
White sword beans were much larger than soybeans in appearance (Fig. (A)). The sizes and weights of dried beans are summarized in Table . The minor axis size and thickness of sword beans were approximately 1.7 times larger than that of soybeans (Table ), while the long axis size was about 3.1 times larger than that of soybeans (Table ). Furthermore, sword beans weighed about 7.8 times more than soybeans (Table ). The standard deviations demonstrated that there were only minor differences between individual sword beans.
Fig. 1. Size transition of sword beans and weight transition of absorbed water during soaking.
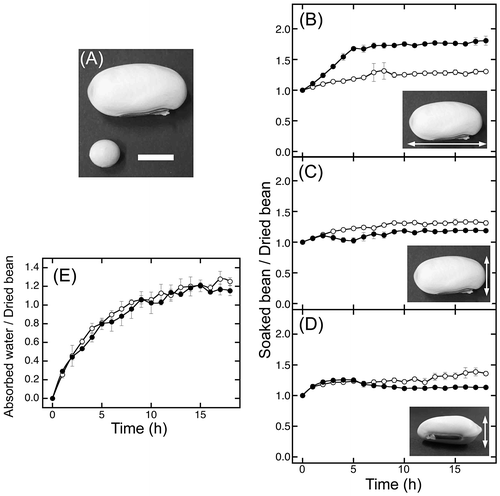
Table 1. Comparison of the size and weight between dried beans.
Bean size and absorbed water weight
Soaking time is an important factor in the production of processed foods from dried beans. Therefore, we determined the optimal time for soaking of beans prior to processing. When beans had absorbed a sufficient amount of water, the size and weight would plateau. To determine the soaking time required for sufficient water absorption, we analyzed the changes in bean size and absorbed water weight for sword beans and compared these data with soaking of soybeans (Fig. (B)–(E)).
Sword bean size increased slowly and nearly reached a plateau after 16 h (Fig. (B)–(D)). In the long and minor axis directions, the size was approximately 1.3 times greater than that of dried beans (Fig. (B) and (C)). For the thickness measurements, the size was approximately 1.4 times greater than that of dried beans (Fig. (D)). For soybeans, the length of the long axis markedly increased at a soaking time of 5 h and nearly reached a plateau, measuring approximately 1.8 times greater than that of dried soybeans (Fig. (D)). Additionally, the length of the minor axis measured 1.1 times greater than that of dried beans at 2 h and then decreased to the same size of dried beans at 5 h; after 5 h, the minor axis size slowly increased by approximately 1.2-fold (Fig. (C)). The thickness of soybeans was approximately 1.2 times greater than that of dried beans by 2 h, plateaued, and then decreased (Fig. (D)). These results demonstrated that the size of soybeans inconstantly changed, in contrast to the gradual and continual increase in size of sword beans.
The weight of absorbed water in sword beans increased and nearly reached a plateau at 13 h, similar to that in soybeans (Fig. (E)). At 18 h, the weight of absorbed water increased by approximately 1.3-fold (Fig. (E), open circles). These results indicated that sword beans absorbed 1.3 volumes of water relative to the weight of dried beans. From the observed changes in sword bean size and weight, we concluded that sword beans absorbed sufficient water after soaking for at least 16 h. Therefore, in subsequent experiments, we soaked sword beans for 18 h.
Extraction of sword bean proteins
For the extraction of sword bean proteins from soaked beans, sword beans were ground in distilled water with a blender. The suspension containing ground beans was heated at 100 °C and then squeezed (Extract A), a method similar to the traditional preparation of soymilk. Extract A was a slightly clouded solution (Fig. (A), a). The protein concentration of Extract A was 1.2 ± 0.2 mg/mL (Fig. (B)), which was much lower than expected, suggesting that heating at 100 °C induced protein precipitation. The same suspension was therefore squeezed without heating (Extract B) and then heated at 100 °C followed by squeezing (Extract C). Extract B had a watery milky appearance (Fig. (A), b) and exhibited a protein concentration of 15.9 ± 1.9 mg/mL (Fig. (B)). During the preparation of Extract C, the protein from Extract B precipitated after heating at 100 °C (Fig. (A), c). Extract C was also a slightly clouded solution; it looked similar to Extract A (Fig. (A), d) and exhibited a protein concentration of 0.8 ± 0.1 mg/mL (Fig. (B)). Thus, the protein concentration of Extract B was more than 13-fold higher than that of Extract A and Extract C (Fig. (B)). Statistical analysis also indicated that the protein concentration of Extract B was significantly higher than that of Extract A and Extract C (p < 0.001) and that the concentration was not significantly different between Extract A and Extract C (p > 0.5). As described above, proteins in Extract B were precipitated by heating at 100 °C (Fig. (A), c). The precipitated proteins were collected by squeezing with a cotton cloth (Fig. (C)). Approximately 8.6 g of wet precipitate was obtained from 100 g of Extract B. These results suggested that most sword bean proteins were precipitated by heating at 100 °C.
Fig. 2. Protein extraction from sword beans.
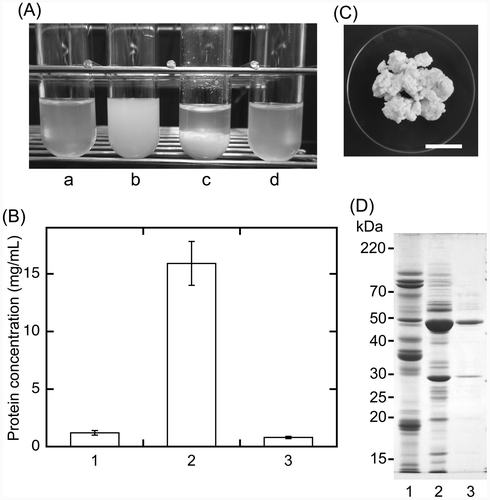
The sword bean proteins contained in Extract B were separated on an SDS-polyacrylamide gel (Fig. (D)). SDS-PAGE analysis indicated that the crude extract of soybean (lane1) and sword bean (lanes 2 and 3) contained a variety of proteins (lane 2). However, the band pattern of sword bean proteins (lane 2) was completely different from that of soybean proteins (lane 1) for an equivalent amount of protein (10 μg per lane). The band pattern also showed that Extract B contained high levels of proteins having molecular weights of approximately 48 and 29 kDa (Fig. (D), lanes 2 and 3). From these results, we chose to use Extract B for subsequent physicochemical analyses to determine the properties of sword bean proteins.
Heat-dependent precipitation of sword bean proteins
Most sword bean proteins were precipitated by heating at 100 °C (Fig. (B)). We therefore investigated the thermal dependency of the precipitation of sword bean proteins. In the preparation of Extract B, we roughly leached the sword bean suspension with a cotton cloth as described above. For further removal of residual precipitable substances from Extract B, the extract was separated into supernatants and precipitates by centrifugation. The supernatants were incubated at various temperatures and then were further separated into supernatants and precipitates by an additional centrifugation. The concentration of protein in the supernatant and the wet precipitate weight were measured for evaluation of heat-dependent precipitation (Fig. (A)). The ratio of the protein concentration increased slightly from 25 to 40 °C (Fig. (A), open circles). Thus, mild heating may only slightly increase the solubility of sword bean proteins in the extract. The ratio of the protein concentration decreased slightly at temperatures ranging from 40 to 75 °C and then decreased dramatically at 90 °C (Fig. (A), open circles). This dramatic decrease suggested that most sword bean proteins were precipitated by heating at more than 90 °C. In contrast, the precipitation efficiency increased substantially at 55 °C, followed by a slight increase at temperatures ranging from 60 to 75 °C and a dramatic increase again at temperatures ranging from 80 to 90 °C (Fig. (A), closed circles). These temperature-dependent changes in protein concentration were roughly consistent with changes in precipitate formation. However, at 55 °C, there were clear differences between protein concentration and precipitate weight. This may be explained by the presence of a non-proteinaceous polymer, such a polysaccharide, following heating at 55 °C.
Fig. 3. Temperature-dependent precipitate formation of sword bean proteins.
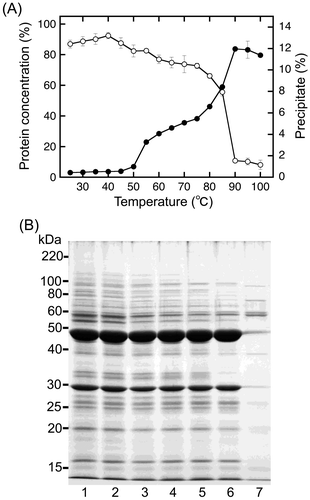
SDS-PAGE analysis provided visualization of the heat-dependent behaviors of sword bean proteins in the supernatant (Fig. (B)). Removal of residual precipitable substances from Extract B only slightly influenced the band pattern (Fig. (B), lanes 1 and 2). Minor protein bands with molecular weights of approximately 20, 27, 33, and 55 kDa were decreased by heating at less than 75 °C (Fig. (B), lanes 3–6). Major protein bands (48 and 29 kDa) were largely decreased in supernatants by heating at 100 °C (Fig. (B), lane 7). Some minor protein bands were not completely disrupted by heating at 100 °C (Fig. (B), lane 7). These results were consistent with the results shown in Figs. (B) and (A).
Magnesium chloride-dependent precipitate formation at various temperatures
During the production of tofu, soybean proteins are precipitated by adding magnesium chloride into soymilk at a concentration of approximately 20 mM.Citation16) We therefore investigated whether adding magnesium chloride into Extract B at the same concentration (20 mM) would induce the precipitation of sword bean proteins (Fig. ). The protein concentration of Extract B was significantly decreased by the addition of magnesium chloride after heating at 25, 50, and 75 °C (Fig. (A)). The precipitation efficiency was significantly increased according to the decreasing protein concentrations at these temperatures (Fig. (B)). The protein concentration and precipitation efficiency were similar for samples treated with or without magnesium chloride after heating at 100 °C, although both the protein concentration and precipitation efficiency were slightly decreased at 100 °C (Fig. (A) and (B)).
Fig. 4. Magnesium chloride-dependent precipitation of sword bean proteins.
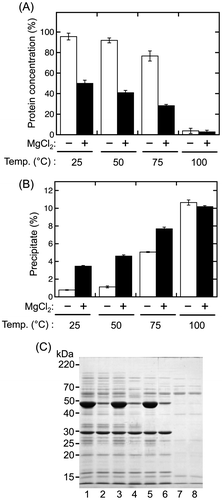
Proteins in Extract B were also analyzed by SDS-PAGE (Fig. (C)). Interestingly, the 48-kDa protein was clearly decreased in the supernatant following addition of magnesium chloride (Fig. (C), lanes 2, 4, and 6). These results suggested that the addition of magnesium chloride induced precipitation of the 48-kDa protein from the crude extract. Analysis of the N-terminal amino acid sequence indicated that the protein consisted of the sequence His-Ser-Gly-His-Ser-Gly-Gly-Glu-Ala-Glu-, which was identical to the N-terminal sequence of sword bean canavalin matured through the removal of the N-terminal signal sequence region comprising the amino acid residues from Met1 to Ala26.Citation17) In addition, the molecular weight of sword bean canavalin is estimated to be approximately 47.6 kDa from the primary sequence. Thus, our results suggested that sword bean canavalin was precipitated from the crude extract following addition of magnesium chloride.
Discussion
In this study, we aimed to establish a novel method for the extraction of sword bean proteins from dried seeds in distilled water and to investigate the precipitation properties of sword bean proteins useful for food development. Interestingly, we found that sword bean canavalin was specifically precipitated by the addition of magnesium chloride. To our knowledge, this is the first report showing that specific precipitation of sword bean canavalin was induced by the addition of magnesium chloride to the crude extracts of sword bean. Thus, our data provide important insights into optimal methods for the extraction of proteins and for the production of processed foods from sword beans.
In previous studies, sword bean proteins have been extracted from ground whole seeds in a solution containing a high concentration of sodium chloride (0.15 mM).Citation10–14) However, protein extracts prepared with high concentrations of salts are not suitable for the production of processed foods. Therefore, we established a new method for the preparation of extracts from dried sword beans with distilled water, similar to the preparation of soymilk. Interestingly, we found that the proteins were precipitated by heating at 100 °C. This was in contrast to the preparation of soymilk, which is prepared by heating of raw soymilk at 100 °C, and has not been shown to cause precipitation of most soybean proteins.Citation18–20) Thus, most soybean proteins are likely to be more thermostable than sword proteins in heated extracts. Heating at less than 85 °C permitted the preparation of extracts containing abundant proteins. In addition, substantial protein precipitation was observed by heating this extract containing abundant proteins at 100 °C. As described in the Results section, we obtained approximately 8.6 g wet precipitate from 100 g of extract. From these data, we estimated that each dried seed might provide approximately 1.4 g of wet precipitate (with each dried bean weighing approximately 2.5 g, as shown in Table ). In addition, heating at 55 °C dramatically increased precipitate formation without a decrease in protein concentration. The inconsistency between the changes in precipitate formation and those in protein concentration implies that a non-proteinaceous polymer, such a polysaccharide, was precipitated by heating at 55 °C. Thus, this substantial protein precipitation may provide the potential for the production of processed foods using sword beans as an alternative protein source; however, it should be noted that the wet precipitate may also contain various substances other than proteins.
Knowledge of the physicochemical properties of proteins within a bean product is important for the development of new processed foods, such as soybean products, as a superior protein source. During the production of tofu from soymilk, heating and the addition of magnesium chloride are important manipulations.Citation16,21) In this study, heating at more than 90 °C induced the precipitation of most of the sword bean proteins. In contrast, adding magnesium chloride induced the precipitation of only some sword bean proteins. From these results, we classified native sword bean proteins into three groups based on precipitate formation (Fig. ). As shown in Fig. , proteins in the first group were not precipitated by heating at more than 90 °C; proteins in the second group were precipitated by heating at more than 90 °C but not by the addition of magnesium chloride; and proteins in the third group were precipitated by both heating and the addition of magnesium chloride. From SDS-PAGE analysis, we found that this third protein group was comprised primarily of a single protein. Further analysis allowed us to identify this protein as canavalin based on the N-terminal amino acid sequence and molecular weight estimation. Thus, the precipitated proteins shown by black circles in Fig. were mainly canavalin.
Fig. 5. Schematic model for the precipitation of sword bean proteins under different conditions.
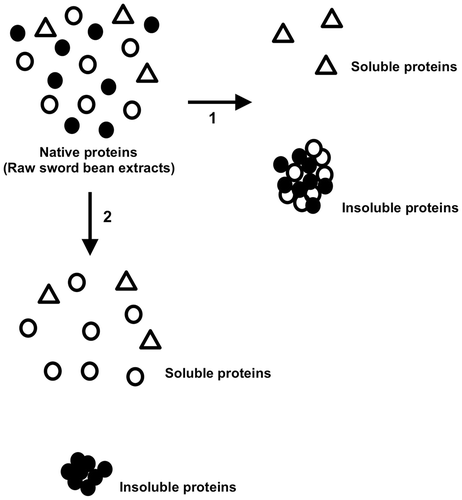
Canavalin was first isolated from jack beans (Canavalia ensiformis)Citation22) and is classified as vicilin or 7S type based on the primary structure.Citation23) The primary structure of jack bean canavalin has been determined using the cDNA sequence.Citation24,25) The primary structure of sword bean canavalin has also been determined using the cDNA sequence.Citation17,26) As described above, the N-terminal sequence of the protein precipitated by the addition of magnesium chloride was completely identical to the N-terminal sequence of sword bean canavalin matured by the removal of the signal sequence region. Only two of the 419 amino acid residues in the mature form differ between the primary sequences of sword bean canavalin and jack bean canavalin. From the high homology of the primary structures, we can assume the functions and structures of the proteins are highly similar. Canavalin is a major storage protein; however, the physiological function of canavalin is still unclear. We found that sword bean canavalin was precipitated following the addition of magnesium chloride. No previous studies have reported precipitation of sword bean or jack bean canavalin induced by the addition of magnesium chloride. Interestingly, several studies have characterized the mechanisms through which magnesium chloride induces protein precipitation. For example, during tofu formation, the addition of divalent cations functions as an initiating factor and facilitates direct interaction among soybean proteins to induce protein precipitation.Citation21) The primary and tertiary structures of canavalin highly resemble those of soybean β-conglycinin, which is a soybean 7S globulin.Citation27) Soybean 7S globulin is also precipitated by the addition of divalent cations.Citation28) Although the primary and tertiary structures of adzuki 7S globulins and common bean phaseolin also highly resemble those of canavalin,Citation27,29) to our knowledge, these proteins have not been reported to be precipitated by magnesium chloride. It is also unclear whether similar precipitation is induced for bean proteins other than soybean and sword bean proteins. These features provide an important insight into the production of processed foods, not only for sword beans but also for other beans. The characteristics of other bean proteins should thus be addressed in future studies. In addition, annexin IV protein interacts with a non-proteinaceous substance through divalent cations coordinated to proteins.Citation29) Consistent with this, we also found evidence of non-proteinaceous substances in our extract. We would expect that canavalin interacts with such non-proteinaceous substances, facilitating precipitation in the presence of magnesium ions. Thus, we will examine the molecular mechanisms of canavalin precipitation in future studies. Our data clearly support the hypothesis that sword bean canavalin may have applications as a new food material for the formation of gels and curds. In addition, analysis of the physicochemical characteristics of sword bean proteins has led to the discovery of a new physiological function of canavalin.
Canavalia seeds contain several antinutritional compounds, such as total tannins, trypsin inhibitors, chymotrypsin inhibitors, phytic acid, canavanine, α-amylase inhibitors, lectins, and total saponins.Citation2,30,31) In animal experiments, sufficient soaking and heating are effective for reducing the amounts of these antinutritional compounds.Citation7,31,32) Although sword beans are traditionally eaten,Citation2,14) more studies will be needed to determine the suitability of sword beans as a food material in the production of processed foods. Interestingly, consumption of sword beans and sword bean extracts has been reported to prevent bone loss.Citation8,9) Although it is unclear whether or not some sword bean proteins prevent bone loss, it is likely that humans can derive functional benefits by eating processed foods containing sword beans. Therefore, sword beans remain an attractive food material for use in the production of processed foods and may have applications as a functional food with health benefits.
In our present study, consistent with the preparation of soymilk extract, we found that soaking time was an important factor determining water absorption in dried sword beans. Maximum water absorption was obtained after more than 16 h of soaking, as measured by changes in bean weight and size. For the preparation of extracts in this study, we soaked beans in distilled water for 18 h. However, it is unclear whether this long soaking time would remove the antinutritional compounds to levels below safety thresholds. Previous studies have shown that shorter soaking times (12 and 15 h) are sufficient for the safe preparation of sword beans.Citation7,33) Thus, for the safe use of sword beans, long soaking times and repeated water exchanges may be needed.
Interestingly, the changes in the sizes of soybeans were different from the trends observed for sword beans during soaking. This difference may result from differences between the shapes of the dried beans and those of the soaked beans. For example, for soybeans, the three-dimensional shape of a dried bean is almost a complete sphere, while that of a soaked bean resembles a bean-shaped sphere. In contrast, sword beans resemble bean-shaped spheres both as dried beans and as soaked beans, with a uniform increase in size during soaking.
Our extract containing abundant proteins is prepared without heating. Sword bean has three trypsin inhibitors, which are the same Bowman-Birk proteinase inhibitors family with the soybean inhibitor.Citation34) In contrast, when the raw extract is heated at 100 °C, most sword bean proteins are precipitated. The heated extract cannot be used as an alternative protein source. These evidences indicate that it is difficult to use the sword bean extract as a drinkable food product such as soymilk. However, the protein precipitates by only heating would be rightly utilized for the production of processed foods as an alternative protein source. In addition, the canavalin precipitate induced by adding magnesium chloride would also be utilized for the production of processed foods by the following heating to the precipitation.
In conclusion, we proposed a new method for the preparation of extracts from dried sword beans using distilled water, which is similar to the preparation of soymilk. Heating and the addition of magnesium chloride induced precipitation of sword bean proteins from the crude extract. In addition, native sword bean proteins were classified into three groups based on precipitate formation, and we observed precipitation of canavalin protein from the crude extracts following the addition of magnesium chloride. Thus, our data provided important information regarding the physicochemical properties of sword beans that may be useful for the production of processed foods.
Author contributions
KN and YA conceived and designed the experiments. KN, TM, and YA performed the experiments. HM, FT, and YA contributed reagents/materials/analysis tools. KN, YT, and YA analyzed the data, wrote the paper, and reviewed and edited the manuscript. All authors read and approved the manuscript.
Disclosure Statement
No potential conflict of interest was reported by authors.
Supplemental material
The supplemental material for this paper is available at http://dx.doi.org/10.1080/09168451.2016.1164587.
TBBB_1164587_Supplementary_File.tif
Download TIFF Image (236.2 KB)Acknowledgments
We thank Aya Sakai for her technical assistance. We would like to thank Editage (www.editage.jp) for English language editing.
Additional information
Funding
References
- Bressani R, Brenes RG, García A, et al. Chemical composition, amino acid content and protein quality of Canavalia spp. seeds. J. Sci. Food Agric. 1987;40:17–23.10.1002/(ISSN)1097-0010
- Siddhuraju P, Becker K. Species/variety differences in biochemical composition and nutritional values of India tribal legumes of genus Canavalia. Nahrung. 2001;45:224–233.10.1002/(ISSN)1521-3803
- Sauer J, Kaplan L. Canavalia Beans in American Prehistory. Am. Antiq. 1969;34:417–424.10.2307/277739
- Smartt J., editor. Canavalia gladiata (Jacq.) D.C. (Sword bean). In: Tropical Pulses. London: Longman Group Ltd; 1976. p. 57–58.
- Rajaram N, Janardhanan K. Nutritional and chemical evaluation of raw seeds of Canavalia gladiata (Jacq) DC. and C. ensiformis DC: The under utilized food and fodder crops in India. Plant Foods Hum. Nutr. 1992;42:329–336.10.1007/BF02194094
- Ministry of Education, Culture, Sports, Science, and Technology, Japan [Internet]. Standard tables of food composition in Japan-. 2015, seventh revised edition, Excel file version. Item number 04023. [cited 2016 March 18]. Available from: http://www.mext.go.jp/component/english/__icsFiles/afieldfile/2016/03/14/1365593_1r5_1.xlsx.
- Sasipriya G, Siddhuraju P. Evaluation of growth performance, serum biochemistry and haematological parameters on broiler birds fed with raw and processed samples of Entada scandens, Canavalia gladiata and Canavalia ensiformis seed meal as an alternative protein source. Trop. Anim. Health Prod. 2013;45:811–820.10.1007/s11250-012-0293-z
- Byun JS, Lee SS. Effect of soybeans and sword beans on bone metabolism in a rat model of osteoporosis. Ann. Nutr. Metab. 2010;56:106–112.10.1159/000277663
- Nakatsuka Y, Nagasawa T, Yumoto Y, et al. Inhibitory effects of sword bean extract on alveolar bone resorption induced in rats by Porphyromonas gingivalis infection. J. Periodontal Res. 2014;49:801–809.10.1111/jre.2014.49.issue-6
- Moreira RA, Cavada BS. Lectin from Canavalia brasiliensis (MART.). isolation, characterization and behavior during germination. Biol. Plantarum. 1984;26:13–120.
- Ceccatto VM, Cavada BS, Nunes EP, et al. Purification and partial characterization of lectin from Canavalia grandiflora Benth. seeds. Protein Pept. Lett. 2002;9:67–73.10.2174/0929866023409002
- Moreno FB, Delatorre P, Freitas BT, et al. Crystallization and preliminary X-ray diffraction analysis of the lectin from Canavalia gladiata seeds. Acta Crystallogr. D Biol. Crystallogr. 2004;60:1493–1495.10.1107/S0907444904014489
- Delatorre P, Rocha BA, Souza EP, et al. Structural of a lectin from Canavalia gladiata seeds: new structural insights for old molecules. BMC Struct. Biol. 2007;7:52.10.1186/1472-6807-7-52
- Bezerra GA, Oliveira TM, Moreno FB, et al. Structural analysis of Canavalia maritime and Canavalia gladiata lectins complexed with different dimannosides: new insights into the understanding of the structure-biological activity relationship in legume lectins. J. Struct. Biol. 2007;160:168–176.10.1016/j.jsb.2007.07.012
- Laemmli UK. Cleavage of structural proteins during the assembly of the head of bacteriophage T4. Nature. 1970;227:680–685.10.1038/227680a0
- Arii Y, Takenaka Y. Magnesium chloride concentration-dependent formation of tofu-like precipitate with different physicochemical properties. Biosci. Biotechnol. Biochem. 2013;77:928–933.10.1271/bbb.120864
- Yamauchi D, Nakamura K, Asahi T, et al. cDNAs for canavalin and concanavalin A from Canavalia gladiata seeds. Nucleotide sequence of cDNA for canavalin and RNA blot analysis canavalin and concanavalin A mRNAs in developing seeds. Eur. J. Biochem. 1988;170:515–520.
- Ono T, Choi MR, Ikeda A, et al. Changes in the composition and size distribution of soymilk protein particles by heating. Agric. Biol. Chem. 1991;55:2291–2297.10.1271/bbb1961.55.2291
- Kohyama K, Sano Y, Doi E. Rheological characteristics and gelation mechanism of Tofu (Soybean Curd). J. Agric. Food Chem. 1995;43:1808–1812.
- Guo S-T, Ono T, Mikami M. Interaction between protein and lipid in soybean milk at elevated temperature. J. Agric. Food Chem. 1997;45:4601–4605.
- Arii Y, Takenaka Y. Initiation of protein association in tofu formation by metal ions. Biosci. Biotechnol. Biochem. 2014;78:86–91.10.1080/09168451.2014.877341
- Sumner JB, Howel SF. The globulins of the Jack bean, Canavalia ensiformis. J. Biol. Chem. 1919;37:137–142.
- Gibbs RE, Strongin KB, McPherson A. Evolution of legume seed storage proteins-a domain to common to legumins and vicilins is duplicated in vicilins. Mol. Biol. Evol. 1989;6:614–623.
- Ng JD, Stinchcombe T, Ko TP, et al. PCR cloning of the full-length cDNA for the seed protein canavalin from the jack bean plant, Canavalia ensiformis. Plant Mol. Biol. 1992;18:147–149.10.1007/BF00018469
- Ng JD, Ko TP, McPherson A. Cloning, expression, and crystallization of jack bean (Canavalia ensiformis) canavalin. Plant Physiol. 1993;101:713–728.10.1104/pp.101.3.713
- Takei Y, Yamauchi D, Minamikawa T. Nucleotide sequence of the canavalin gene from Canavalia gladiata seeds. Nucleic Acids Res. 1989;17:4381.10.1093/nar/17.11.4381
- Maruyama N, Adachi M, Takahashi K, et al. Crystal structures of recombinant and native soybean β-conglycinin β homotrimers. Eur. J. Biochem. 2001;268:3595–3604.10.1046/j.1432-1327.2001.02268.x
- Appu Rao AG, Narasinga Rao MS. Binding of Ca(II), Mg(II), and Zn(II) by 7S fraction of soybean proteins. J. Agric. Food Chem. 1976;24:490–494.
- Fukuda T, Maruyama N, Salleh MR, et al. Characterization and crystallography of recombinant 7S globulins of adzuki bean and structure-function relationships with 7S globulins of various crops. J. Agric. Food Chem. 2006;56:4145–4153.
- Arii Y, Butsusihta K, Fukuoka SI. Role of calcium-binding sites in calcium-dependent membrane association of annexin A4. Biosci. Biotechnol. Biochem. 2015;79:978–985.10.1080/09168451.2014.1003131
- Makkar HP, Siddhuraju P, Becker K. Plant secondary metabolites. Methods Mol. Biol. 2007;393:1–6.10.1007/978-1-59745-425-4
- Berlitz HD, Grosch W, Schieberle P. Food chemistry. 4th ed. Germany: Springer, Berlin Hiedelberg; 2009. Chapter 16, Legumes; p. 746–769.
- Ekanayake S, Skog K, Asp NG. Canavanine content in swords beans (Canavalia gladiata): analysis and effects of processing. Food Chem. Toxicol. 2007;45:797–803.10.1016/j.fct.2006.10.030
- Park SS, Sumi T, Ohba H, et al. Complete amino acid sequences of three proteinase inhibitors from white sword bean (Canavalia gladiata). Biosci. Biotechnol. Biochem. 2000;64:2272–2275.10.1271/bbb.64.2272