Abstract
The filamentous fungus Trichoderma reesei is a potent cellulase producer and the best-studied cellulolytic fungus. A lot of investigations not only on glycoside hydrolases produced by T. reesei, but also on the machinery controlling gene expression of these enzyme have made this fungus a model organism for cellulolytic fungi. We have investigated the T. reesei strain including mutants developed in Japan in detail to understand the molecular mechanisms that control the cellulase gene expression, the biochemical and morphological aspects that could favor this phenotype, and have attempted to generate novel strains that may be appropriate for industrial use. Subsequently, we developed recombinant strains by combination of these insights and the heterologous-efficient saccharifing enzymes. Resulting enzyme preparations were highly effective for saccharification of various biomass. In this review, we present some of the salient findings from the recent biochemical, morphological, and molecular analyses of this remarkable cellulase hyper-producing fungus.
Graphical abstract
For Trichoderma reesei, transcriptional regulation of cellulolytic enzyme genes, comparative genomics and morphological insights, and applications of them were summarized.
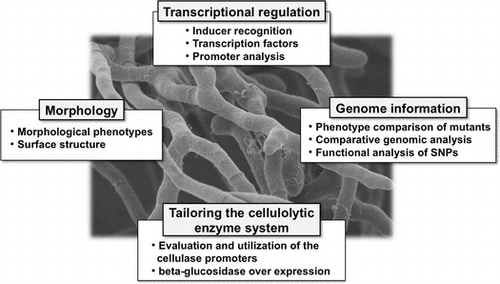
Plant biomass is the most abundant renewable carbon resource in nature. Major components of plant biomass are cellulose, hemicellulose, and the aromatic polymer lignin. In recent years, bioconversion of plant polysaccharides has received much attention because of its potential application in the pulp and paper, food and feed, and fuel industries. In nature, this biomass is utilized by a variety of cellulolytic organisms, which thereby play a key role in carbon recycling into the ecosystem. Numerous organisms produce cellulase and hemicellulase, including bacteria,Citation1) fungi,Citation2) and insects.Citation3) Among them, the filamentous fungi are considered extremely efficient degraders of plant polysaccharides. They produce a large amount of cellulases that are comprised of three types of enzymes: cellobiohydrolases (CBH: EC 3.2.1.91) and endoglucanases (EG: EC 3.2.1.4), which act synergistically to degrade cellulose to cello-oligosaccharides (mainly cellobiose), and β-glucosidases (BGL: EC3.2.1.21), which hydrolyze cellobiose into glucose. In addition, the recently discovered class of enzymes known as lytic polysaccharide monooxygenases (LPMOs) has also been recognized as an effective auxiliary enzyme for cellulose degradation.Citation4,5)
The filamentous fungus Trichoderma reesei (anamorph of Hypocrea jecorina) (Fig. ) is a fast-growing Ascomycete that is widely distributed in soil environments. It was first isolated from the South PacificCitation6) and is well-known for its ability to secrete high amounts of cellulolytic enzymes. Generally, T. reesei produces cellulase when cellulose is available as the sole carbon source. In addition to cellulose, some soluble disaccharides (cellobiose, α-sophorose, lactose, etc.) also trigger cellulase expression by this organism (reviewed by Bisaria and Mishra, and Kubicek et al.Citation7,8)). Under cellulase-inducing conditions, the genes encoding cellulases are transcribed coordinately suggesting a common regulatory mechanism for the control of cellulase expression.Citation9,10) Several transcription regulators that control cellulase gene expression have been isolated from T. reesei. These include Xyr1, a key activator of cellulase and hemicellulase expression,Citation11,12) Ace2 and Ace3, additional activators,Citation13,14) Ace1, a repressor,Citation15,16) and BglR, an activator for β-glucosidase gene.Citation17) In addition to pathway specific regulators, Cre1, the wide domain carbon catabolite repressor that represses cellulase gene expression during growth on glucose, has also been reported.Citation18–20)
Fig. 1. The filamentous fungus Trichoderma reesei.
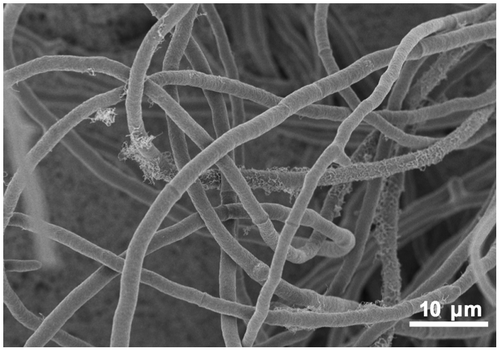
Because of their industrial importance and the variety of uses for T. reesei hydrolases, a number of mutants that improved cellulase productivity have been developed by classical mutagenesis. At present, several industrial mutants have been reported to secrete over 30 g/L of extracellular proteins into culture media.Citation21) Among the mutant strains, T. reesei QM9414, a cellulase overproducing strain developed earlier by MandelsCitation22) at Natick Laboratories in US, is used as the standard. Several hyper-cellulolytic mutant strains have since been derived from QM9414 by further mutagenesis.Citation23) In Japan, several T. reesei mutants have been developed in order to generate a lineage of new strainsCitation24) (Fig. ). The PC-3-7 strain exhibits twice as much cellulase activity as QM9414 when induced by α-sophorose.Citation10) Due to its high cellulase and hemicellulase productivity,Citation25) the T. reesei PC-3-7 mutant is used as a model strain in our laboratory for the study of gene expression and protein production. We have carried out a comparative genomic analysis of this mutant lineage and using high-throughput genomic DNA sequencing technologies and identified all single-nucleotide polymorphisms (SNPs) that exist between the wild-type QM6a and PC-3-7.Citation26) Recently, we reported that PC-3-7’s enhanced cellulase-producing ability is related to the SNPs in cre1, bglr and bgl2.Citation17,26,27)
Fig. 2. The lineage of mutant T. reesei strains developed in Japan.
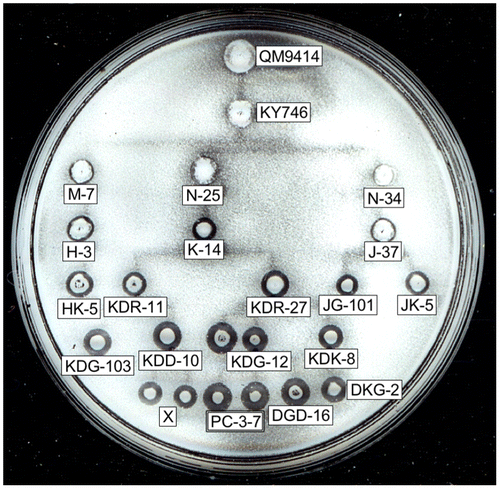
This enhanced cellulase-producing ability, if combined with an efficient saccharification machinery, could aid in an efficient bioconversion of cellulosic biomass into biofuels. The second-generation biofuel ethanol produced from lignocellulosic biomass offers a promising alternative and environmentally friendly energy due to its cost benefits, abundance, and renewability.Citation28–30) In general, however, BGL activity has been found to be quite low in cellulase preparations from T. reesei.Citation31) The low BGL activity causes cellobiose accumulation, resulting in a reduced biomass conversion efficiency, accompanied by cellobiose-mediated product inhibition of CBHI (Cel7A).Citation32) We screened more than 40 species of BGL and selected A. aculeatus BGL1 (AaBG1) as the best enzyme. Recombinant T. reesei strains that express an AaBG1 under the control of the selected promoters were constructed. The developed enzymes showed high activity for all types of pretreated cellulosic biomasses, indicating its usefulness in the saccharification of various cellulosic biomasses.
Further understanding of the mechanisms responsible for cellulase production could help in producing better strains that are more suitable for industrial use. Here, we discuss some of the current understanding of cellulase production, the various genetic factors involved and the advances in recent years in generating desirable strains that combine all the salient features.
I. Mechanism of cellulase induction in T. reesei
In T. reesei, the production of cellulolytic and xylanolytic enzymes are regulated at the transcriptional level depending on the carbon source available. The production of these enzymes begins only when the fungi need to use plant polysaccharides as an energy and carbon source. These enzymes are produced abundantly when the fungus is cultivated on the media containing cellulose, xylan and mixtures of plant polysaccharides as a sole carbon source.Citation33) However, the production of the enzymes does not occur in the presence of glucose and other easily metabolizable carbon sources such as fructose. The production of many of these enzymes has been reported to be coordinately regulated at the transcriptional level along with other enzymes.Citation9,10,34) It can be assumed that co-regulation of hydrolytic enzymes enables an efficient degradation of plant cell wall polysaccharides via the synergistic action of all these enzymes. Because plant polymers cannot directly enter the fungal cell, it has been proposed that the transcription of genes encoding cellulase and xylanase is stimulated by the presence of trace amount of soluble sugars derived from cellulose through constitutively expressed cellulase activity. For cellulase genes, this theory is supported by findings that trace amounts of mRNA corresponding to major cellulase genes are detected in uninduced cellsCitation35) and that the addition of anti-cellulase antibody hindered cellulase formation during growth on cellulose.Citation36) The primary end product generated from cellulose degradation by cellulases is cellobiose. It has been shown that cellulase production is induced by cellobiose in many fungi, including T. reesei.Citation37) In some cases, the production of cellulase is not observed during growth on cellobiose due to the hydrolysis of cellobiose into glucose by extracellular BGL. Glucose inhibits cellobiose uptakeCitation38) and can cause carbon catabolite repression (CCR) of cellulase expression. Some reports describe that the methods to reduce BGL activity, such as the addition of a β-glucosidase inhibitor to the culture, knock-out of the major extracellular BGL, or lowering the pH of the culture medium, resulted in an enhanced cellulase production.Citation39–41) Results from kinetic studies showed that since the Km of BGL toward cellobiose was 20- to 40-fold higher than that of β-glucoside permease,Citation38) indicating that cellobiose is released from cellulose in concentrations that favor its uptake rather than hydrolysis. Therefore, it is possible that cellobiose is the in vivo inducer of cellulase induction in T. reesei.
The most potent inducer of cellulase gene expression for T. reesei is α-sophorose. It has been proposed that α-sophorose is converted from cellobiose by transglycosylation.Citation42) Disruption of the gene encoding BGLI that is the major extracellular BGL has been shown to result in a delay in the production of cellulase on cellulose, but not on α-sophorose. A BGLI overproducing strain has been reported to exhibit higher cellulase production. The same report also showed that nojirimycin, a β-glucosidase inhibitor, inhibited cellulase production on α-sophorose.Citation43) Therefore, α-sophorose might not be the final inducer for cellulase induction and it is possible that some other BGL(s) are involved in true inducer formation. Among the BGL genes, the expression of bgl2 has been shown to be responsible for α-sophoroseCitation31) and it is partially released from the control of Xyr1, the xylanase and cellulase gene activator.Citation44) This indicates that bgl2 expression is controlled by some other factors on α-sophorose. Therefore, it is possible that BGLII is located upstream of the cellobiose signaling cascade in T. reesei. Recently, a report focusing on the physiological role of BGLII described that BGLII participates in the rapid induction of cellulase genes by cellulose and cellobiose.Citation45) Furthermore, comparative genomic analysis has revealed that a mutation in BGLII results in enhanced cellulase productivity in T. reesei mutants, indicating that BGLII has a definite part in cellulase inducer formation in T. reesei (see Chapter 5). Furthermore, an additional compound with a low molecular weight sugar, l-sorbose, promotes cellulase gene expression.Citation10) This suggests that several signal transduction pathways responsible for each of these inducers might control cellulase expression in parallel.
In T. reesei, the production of xylanase (XYN) is induced by cellulose and its derivatives (e.g. α-sophorose), xylan and its degradation products (e.g. d-xylose and xylobiose), arabitol and l-sorbose.Citation33,46) Unlike cellulase genes, expression of xylanase genes is not co-regulated and differences in their expression profiles have been reported. Among the xylanase genes, the expression of xyn1 is mainly induced by d-xylose and is subjected to CCR,Citation47) whereas expression of xyn2 is partially constitutive and is further induced by xylobiose and α-sophorose.Citation48) On the other hand, the expression of xyn3 from T. reesei PC-3-7 is induced by Avicel, α-sophorose, and l-sorbose, but not by d-xylose, xylooligosaccharides or birch wood xylan, and is subjected to glucose repression.Citation48) These data suggest that the induction pathway for xylanase gene expression partially differs from that regulating the cellulase gene expressions. Based on the constitutive expression of xyn2, a general model for the extracellular xylan recognition has been proposed.Citation49) The authors hypothesized that low levels of constitutively expressed XYNII initially attack the xylan backbone resulting in the release of xylooligosaccharides. Thereafter, the released xylooligosaccharides trigger the induction of xyn1, xyn2, bxl1, and other xylanolytic genes expression.
II. Transcriptional regulators involved in the regulation of cellulolytic and xylanolytic gene expression in T. reesei
Over the past two decades, significant progress has been made in elucidating the molecular mechanisms involved in the transcriptional regulation of cellulolytic and xylanolytic genes in filamentous fungi. Extensive analysis of the expression profiles of cellulase and xylanase genes as well as their promoter regions have led to the identification of several cis- and trans-acting elements regulating gene expression. This regulatory system includes both pathway-specific and wide-domain regulatory controls of transcription. Transcription factors involved in the regulation of the cellulase and xylanase gene expression and their functions are discussed in the following section and are summarized in Table and Fig. .
Table 1. Transcription factors involved in the regulation of the cellulase and hemicellulase genes expression in T. reesei.
Fig. 3. Schematic representation for the transcriptional regulation network of the genes encoding cellulolytic and xylanolytic enzymes in T. reesei.
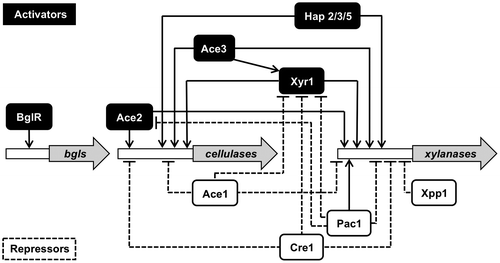
II. i. Specific transcription factors for cellulase and xylanase genes
II.i.a. Xyr1 (xylanase regulator 1)
The transcription activator Xyr1 is the major activator for cellulase and xylanase genes and is isolated as an orthologue of A. niger XlnR.Citation12) The xyr1 open-reading frame encodes a typical fungal-specific Zn2Cys6-binuclear zinc cluster transcription factor with 934 amino acids and with a predicted molecular mass of 102 kDa. Disruption of xyr1 has been shown to abolish the expression of major cellulolytic genes (cbh1, cbh2, egl1 and bgl1) and xylanolytic genes (xyn1, xyn2, and bxl1), regardless of the inducer (xylose, xylobiose, α-sophorose, and lactose) and the mode of gene expression (basal, derepression, and induction).Citation11,50) In addition, Xyr1 also regulates the transcription of the gene encoding d-xylose reductase (xyl1).
Functional Xyr1 binding sequences have been reported to 5′-GGCTAA-3′ motifs arranged as an inverted repeat separated by a 10-bp spacer within the xyn1 promoter region, and an inverted repeat of a 5′-GGGTAA-3′ and a 5′-GGCTGG-3′ motif separated by 12-bp spacer within the xyn2 promoter region, respectively.Citation12,51) From further analysis, target sequence of Xyr1 binding was determined as 5′-GGCWWW-3′ and the single 5′-GGCWWWW-3′ motifs play important roles as functional XYR1-binding sites.Citation44) 5′-GGCWWW-3′ motifs on cellulase and xylanase promoters are shown in Fig. . Recent genomic analysis of T. reesei mutants has revealed insights into the structure of Xyr1. The genomic analysis of cellulase negative mutant revealed that the truncation of Xyr1 C-terminal 140 amino acids by a frame shift was responsible for cellulase-negative phenotype. The truncated region includes the highly conserved domain structure of this family, the C-terminal acidic activating domain. Therefore, this domain of Xyr1 is an essential for expression of cellulase and hemicellulase genes.Citation52) Interestingly, a single amino acid mutation in Xyr1 acidic activating domain (A804V by Lichius et al., corresponding to A824V by Derntl et al.) of an industrial strain brought out a glucose-blind phenotype in which constitutive xylanase expression and elevated basal expression of cellulase were observed,Citation53) suggesting that this mutation is only functional when the whole acidic activation domain is present. xyr1 gene expression is regulated at the transcriptional level by a Cre1-mediated repression/derepression mechanism, but is not subjected to induction by d-xylose and xylobiose. In addition, xyr1 transcription has also shown to be repressed by Ace1 repressor.Citation54) Just recently, using a pull-down approach, an additional xylanase regulator was found to bind the repressive cis-element in the promoter of xyn2, AGAA region.Citation55) This regulator is identified as Xpp1, a basic helix-loop-helix protein, and its target sequence is a hexameric palindrome, 5′-WCTAGW-3′, with an inverted AGAA-repeat.Citation56) A comparison of strain with a deletion or overexpression of xpp1 with the parent strain showed that Xpp1 regulates the expression of hemicellulase genes, but not cellulase genes, at later cultivation stages. The expression of Xpp1 was found to be upregulated, in addition to d-glucose, by high d-xylose availability.Citation56)
Fig. 4. The localization of Xyr1-binding consensus sequences within the 1.5-kb upstream region of cellulolytic and xylanolytic genes.
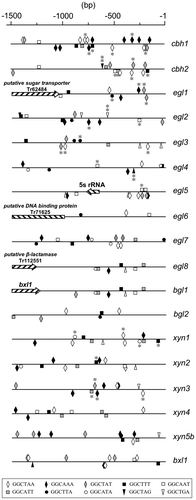
II.i.b. Ace2 (Activator of cellulase expression 2)
Ace2 is an additional activator of cellulase and xylanase. The ace2 gene was isolated from a cellulose-induced cDNA library of T. reesei using the yeast expression screening system (yeast one-hybrid method) on the basis of the ability of its translation product to activate the transcription of a 1.15-kbp T. reesei cbh1 promoter fused to the Saccharomyces cerevisiae HIS3 gene.Citation57) ace2 encodes a typical zinc binuclear cluster protein consisting of 341 amino acids. Disruption of ace2 leads to reduce expression of all the main cellulase genes and xyn2, particularly at the early stage of induction, in the presence of cellulose, but not during induction by α-sophorose. This indicates that Ace2 acts as an activator for cellulase and xylanase gene expression but other activators should also be involved in this regulation. It also suggests that cellulose- and α-sophorose-induced gene expressions in T. reesei are controlled by at least partially different molecular mechanisms. The DNA-binding domain of Ace2 (residues 1 to 58) has shown to bind in vitro to 5′-GGCTAATAA-3′ sequences in the cbh1 promoter region and 5′-GGGTAA-3′ sequences in the cbh2 and xyn2 promoter region. In addition, binding of in vitro translated full-length of Ace2 to an inverted repeat motif consisting of a 5′-GGGTAA-3′ and a 5′-GGCTGG-3′ sequence in xyn2 promoter region has been demonstrated by Stricker et al.Citation51) Up until now, an Ace2 homologue has not yet been found in the other fungal genomes, including in A. nidulans and A. oryzae, suggesting that Ace2 is a specific transcriptional activator for T. reesei.
II.i.c. Ace1 (Activator of cellulase expression 1)
Ace1 is a class I zinc finger protein that contains three Cys2His2-type zinc finger repressor.Citation58) The ace1 was isolated from cellulose-induced cDNA library of T. reesei in a similar screen along with the isolation of ace2.Citation15) Ace1 binds to 5′-AGGCA-3′ motifs within the cbh1 promoter region and to a 5′-GGCTAA-3′ motif within the xyn1 promoter region in vitro.Citation12,15) Although ace1 activated the cbh1 promoter in S. cerevisiae, its deletion from T. reesei genome resulted in an increased expression of all the major cellulase genes (cbh1, cbh2 and egl1) and xylanase genes (xyn1 and xyn2) under α-sophorose- and cellulose-inducing conditions.Citation16) Based on this finding, Ace1 is now considered to be a repressor of the induced expression of these genes. The discrepancy between S. cerevisiae and T. reesei in the Ace1 has been explained by the fact that the Ace1 expressed from the yeast expression library was truncated and lacked 242 amino acids from the N-terminus.Citation16) This suggests the functional importance of the N-terminus region for repressing effects of Ace1. Orthologues of ace1 have been recently identified in the other 19 fungal genomes.Citation59) Among them, A. nidulans stzA has been well characterized and its translation product has been shown to encode an abiotic stress response regulator that mitigates sensitivity to salt and DNA-damaging agents.Citation60) Overall, these findings suggest that Ace1 may have a more general regulatory role in addition to the repressor function of cellulase and xylanase gene expression.
II.i.d. BglR (beta-glucosidase regulator)
Recently, some novel transcription factors involved in the regulation of polysaccharide bioconversion have been reported. From comparative genome analysis between QM9414 and cellulase hyper-producing mutant PC-3-7, bglr encoding fungal-specific zinc binuclear cluster protein is BglR. Although preliminary results show that this gene may function as the activator of BGL genes on the cellobiose cultivation,Citation17) detailed analysis is required to further characterize the function of BglR.
II.i.e. Ace3 (Activator of cellulase expression 3)
ace3 encoding activator Ace3 was isolated using specific gene screening strategy.Citation14) Genes that encode a protein with a DNA binding domain and are induced on the different lignocellulose-derived materials were selected. Among them, genes that the locus on the genome is near the cellulase or hemicellulase gene were chosen and were overexpressed in T. reesei. ace3 was one of these genes and its overexpression led to the enhancement of cellulase expression. Deletion of ace3 not only led to a significantly reduced cellulase and hemicellulase activity, but also affected xyr1 expression. This suggests that Ace3 is located on upstream of Xyr1 in the induction mechanism of cellulase and hemicellulase.
II.ii. Global transcription factors
II.ii.a. Cre proteins
In the presence of easily metabolizable carbon sources (e.g. d-glucose), the biosynthesis of the enzymes that utilize of less-favored carbon sources is repressed via CCR. In filamentous fungi, CCR is mediated by the wide-domain transcription factor Cre proteins, which repress the expression of numerous genes by binding to specific sites in the promoter region of the target genes. In T. reesei, Cre1 was isolated as an orthologues of the A. nidulans and A. niger CreA.Citation18) The Cre1 proteins are class I zinc finger protein, possessing two Cys2His2-type DNA-binding motifs. Cre1 is also a phosphoprotein and a Ser241 residue within the conserved acidic region has been identified as a phosphorylation site. It has been suggested that Cre1 is phosphorylated by casein kinase II-like protein in the presence of d-glucose and this phosphorylation is required for DNA binding of Cre1.Citation61) The consensus binding sequences for Cre1 have been determined as 5′-SYGGRG-3′Citation19,62) and the only two closely spaced binding motifs have been shown to be the functional binding sites in vivo.Citation63,64) The cbh1 and xyn1 expression have been demonstrated to be under the direct control of Cre1.Citation20,65,66) However, it has been reported that Cre1 dose not mediate cbh2 and xyn2 expression directly. This contradiction has been explained by the double-lock mechanism of Cre1-mediated gene regulation.Citation54) Based on the complementation experiments of cre1 truncated-mutant T. reesei Rut C-30 with full-length cre1 gene, it was shown that CCR of cellulase and hemicellulase genes are mediated by Cre1.Citation67,68) PC-3-7, the Japanese mutant has a single-nucleotide mutation in cre1 at the position encoding the amino acid of the DNA binding domain, which results in the partial release of PC-3-7 from CCR.Citation26) Recently, additional factors controlling CCR have also been reported in T. reesei. Cre2, an orthologue of CreB of A. nidulans, is a ubiquitin C-terminal hydrolase that might be involved in the deubiquitination of Cre1.Citation69) In Aspergillus nidulans, CreB is stabilized by forming a complex with CreC that is a WD40-repeat protein. Because the orthologue of CreC exists in T. reesei genome as well, the mechanism of CCR may be conserved between these two species.
II.ii.b. Hap complex
In filamentous fungi, Hap complexes have been identified in Aspergillus species (HapB/C/E), T. reesei (Hap2/3/5), and N. crassa (Hap2/3/5).Citation70,71) These CCAAT binding complexes have been shown to modulate the expression of several genes such as the A. nidulans acetamidase gene (amdS), the A. oryzae Taka-amylase gene (taa), the A. nidulans penicillin biosynthesis gene (ipnA and aatA), N. crassa NADPH-specific glutamate dehydrogenase gene (am),Citation72) and the T. reesei cellulase and xylanase genes (cbh2 and xyn2).Citation66,73) The CCAAT sequence is present in approximately 30% of eukaryotic promoters.Citation74) Generally, these elements lie about 50–200 bp upstream from the transcriptional starting point and can be present in either orientation. The first heteromeric protein complex bound to CCAAT sequences was found in S. cerevisiae.Citation75,76) Since this discovery, CCAAT sequence binding complexes have been isolated and characterized in various eukaryotic organisms, including in fungi, plant, and mammals. Different names are given for the respective CCAAT sequence binding complexes (e.g. HAP in S. cerevisiae, A. nidulans, T. reesei and Arabidopsis thaliana, CBF in rat, and NF-Y in human and Xenopus). Recent analysis on the regulation of cellulase and xylanase gene expression in T. reesei has indicated that the Hap2/3/5 complex cooperatively participates in gene regulation with other pathway-specific regulators, such as Xyr1 and Ace2.Citation51,66) Interestingly, Hap complex of A. nidulans has been shown to play a role in the formation of an open chromatin structure in the promoter region of the amdS.Citation77) A similar observation has been reported for cbh2 of T. reesei, in which the binding of Hap complex to the cbh2 activating element affected the nucleosome rearrangement of the cbh2 promoter.Citation78) These findings might suggest that the Hap complex plays a role in modifying the nucleosome structure, enabling the recruitment of other transcription factors in the promoter region.
II.ii.c. Pac1
The ambient pH affects the growth and the types or amounts of secreted protein in filamentous fungi. A pH signal transduction pathway exists in these organisms and its mechanism has been well investigated in Aspergillus nidulans. This pathway contains six pal proteins (PalA, B, C, F, H, and I)Citation79–83) and a pH-responsive transcription factor PacC.Citation84) PacC activates alkali-expressed genes and represses acid-expressed genes on a high pH condition. T. reesei possesses the orthologue of PacC and six pal proteins.Citation85) A large number of genes, especially encoding transporters, signaling-related proteins, extracellular enzymes, and proteins involved in different metabolism-related functions were found to be pH-responsive in T. reesei. Some cellulase and hemicellulase genes were also found among the pH responsive genes. In particular, the genes encoding hemicellulases appeared to be differentially regulated depending on the ambient pH. The xylanase genes, xyn2, xyn3, and GH30 xylanase genes are abundantly expressed at high pH, whereas xyn1 and xyn5 are more highly expressed at a lower pH. Although T. reesei produces meager amount of cellulase and hemicellulase on high pH, deletion of pac1 dramatically increased cellulase production and the transcription levels of major cellulase genes at neutral pH. This suggests that Pac1 is involved in the regulation of cellulase production. In addition, the expression levels of xyr1 and ace2 also increased in the ∆pac1 mutant at neutral pH. Although a variety of glycoside hydrolase genes respond to changes in pH, only a few are certainly under the regulation of Pac1. It is possible that other regulatory mechanisms of cellulase and hemicellulase genes have a stronger effect and mask the effect of Pac1 regulation.Citation86)
III. cis-acting elements on the promoter of cellulase and xylanase genes in T. reesei
As described, numerous attempts have been made to elucidate the transcriptional regulation of cellulase and xylanase genes in T. reesei. Although detailed molecular mechanisms underlying these regulatory controls are still poorly understood, several promoter regions of the hydrolase-encoding genes have been investigated for their cis-acting elements and transcription factors. Recent findings concerning the transcriptional regulation of cellulase and xylanase genes in T. reesei are summarized below.
III.i. Promoter analysis of cellulase genes
cbh1 is the most highly expressed cellulase gene in T. reesei. It has been estimated that its translation product (CBHI) constitutes approximately 50–60% of the total secreted protein. This large amount of enzyme is produced from a single copy of cbh1 gene in the genome. Thus the cbh1 promoter is considered to be a very strong promoter. Due to its high protein productivity, the cbh1 promoter has been frequently used for heterologous and homologous protein production by T. reesei. In addition, the cbh1 promoter has also received a lot of attention as the target for the analysis of gene regulation. First, using a promoter deletion assay, Ilmén et al.Citation67) determined the important nucleotide regions responsible for cbh1 gene expression. They identified that the cis-acting elements that mediate glucose repression are positioned upstream of −500 bp from the translation initiation codon, and that the mutation of the Cre1 site at −720 bp was sufficient for derepression. A later study revealed the presence of three putative Cre1 binding sites around the −700 bp region, and confirmed in vitro binding of the recombinant Cre1 to these binding motifs.Citation19) The deletion analysis also showed that the elements responsible for α-sophorose induction lie within a 161-bp region upstream of ATG. In addition, Henrique-Silva et al.Citation87) have reported that the region between −241 and −72 bp upstream of the TATA-box is responsible for induction by cellulose, and that the 72 bp region around ATG is responsible for a low constitutive expression during induction on neutral carbon source. After the isolation of Ace1, Ace2, Xyr1, the roles of these factors in cbh1 expression as well as in the in vitro binding of the transcription factors to the cbh1 promoter region have been analyzed.Citation15,57,88)
cbh2 is the second highest expressed gene under a cellulase-inducing condition. Therefore, the promoter of cbh2 has also been the target for exploration of the cis-acting element. Using electrophoretic mobility shift assay (EMSA) technique, an early study determined the area between −361 and −170 in the cbh2 promoter region as capable of forming a α-sophorose-specific DNA-protein complex.Citation89) Subsequently, Zeilinger et al.Citation65) identified the cbh2-activating element (CAE) as the essential regulatory sequences for cbh2 expression. The CAE comprises 5′-ATTGGGTAATA-3′ undecameric nucleotide sequences that contain two adjacent binding sites for transcription factors (The CCAAT sequence on the non-coding strand and the 5′-GGGTAATA-3′ sequence on the coding strand). After identification of the CAE, the same authors cloned the genes encoding hap2, hap3, and hap5 from T. reesei and provided evidences for the binding of the Hap2/3/5 complex to CAE in vitro.Citation73) Schmoll et al.Citation90) showed the ability of Ace2 for interacting with 5′-GGGTAA-3′ sequences within the CAE. From the results of the in vivo foot printing analysis, it has been suggested that both transcription factors constantly bind to CAE under inducing and repressing conditions.Citation65) The cbh2 promoter region also contains a single Cre1 binding site at −222 but is not directly regulated by Cre1.Citation78)
Studies on cellulase gene regulation in T. reesei have been limited to the major cellulase genes, and little is known about how the minor cellulase genes are regulated and how the expression level of each cellulase gene is determined. To understand the gene regulation of minor cellulase genes, functional analysis of egl3 encoding endoglucanase III was carried out.Citation91) egl3 is induced along with other cellulase genes, but its expression level is very low, with mRNA levels of almost 1% of those of cbh1 mRNA.Citation10) In the upstream region of egl3, there are two 5′-GGCTAA-3′ motifs, six CCAAT boxes, two Cre1 binding sites, and no Ace1 site. From the promoter deletion analysis with GUS (β-glucuronidase) reporter system, it was revealed that the 5′-GGCTAT-3′ sequence, which resembles to the Xyr1 binding site 5′-GGCTAT-3′ at −1,018 bp and 5′-GGCTAA-3′ sequence at −979 bp on the noncoding strand, serves in egl3 induction. In addition to these sites, the 5′-GGCTAA-3′ sequence at −176 bp also has been shown to participate in the induction to a smaller extent. The formation of a complex between Xyr1 and these sites was confirmed by EMSA analysis and a sequence recognition analysis showed that Xyr1 preferred 5′-GGCTAA-3′ to 5′-GGCTAA-3′. These results, together with the in vivo data, suggest that Xyr1 plays an activating role in egl3 expression and can recognize not only the 5′-GGCTAA-3′ sequence but also the 5′-GGCTAT-3′ sequence. However, depending on the 5′-GGCTAA/T-3′ motif, different binding modes of Xyr1 were observed, indicating that the sequences around 5′-GGCTAA/T-3′ motif are also important for Xyr1 binding.
Promoter structure of cbh1, cbh2, and egl3 is summarized in Fig. .
Fig. 5. The architecture of the cbh1 (A), cbh2 (B) and egl3 (C) promoter.
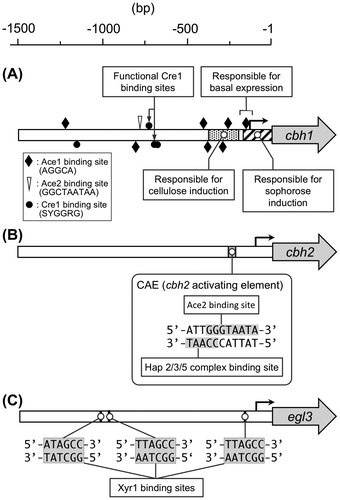
III.ii. Promoter analysis of xylanase genes
As described in the section “Mechanism of cellulase induction in T. reesei”, expression of the three major xylanase genes (xyn1 to xyn3) is not co-regulated and several differences in their mode of expression have been reported. To elucidate the detailed molecular mechanisms controlling their transcriptional regulation, the promoter region of the three major xylanases has been studied extensively.
Early promoter deletion analysis of the 5′-upstream region of xyn1 revealed that a 217-bp region between −321 and −534 from the xyn1 translation initiation site bears all the necessary information for the regulation of xyn1 expression.Citation47,48) Detailed analysis of the 217-bp region led to the identification of three cis-acting elements including the CCAAT sequence, two Cre1 binding sites, and an inverted repeat of the 5′-GGCTAA-3ʹ motifs separated by a 10-bp spacer.Citation12) In this study, the authors investigated the Xyr1 binding to the inverted repeat motif with other regulators including Ace1 and Ace2. EMSA, using recombinant regulators, revealed that Xyr1, but not Ace2, is able to interact with both of the 5′-GGCTAA-3′ motifs and it was proposed that Xyr1 binds as a homodimer to the inverted repeat motif to activate xyn1 expression. Furthermore, specific binding of Ace1 to the downstream 5′-GGCTAA-3′ motif was also demonstrated. Based on these results, Rauscher et al.Citation12) hypothesized that the competition between Xyr1 and Ace1 for the downstream 5′-GGCTAA-3′ element could occur during the induction of xyn1. In addition to these findings, the binding of Hap2/3/5 complex and Cre1 to the respective binding motifs was also demonstrated by EMSA using recombinant proteins. The functionality of the Cre1 binding sequences for xyn1 expression was investigated by promoter mutation analysis, and the results showed that xyn1 expression is under the direct control of Cre1.Citation47)
Zeilinger et al.Citation48) reported that a 55-bp fragment from −235 to −180 of the xyn2 promoter region contains all cis-acting elements essential for the regulation of xyn2 expression. Using both in vitro as well as in vivo techniques, Würleitner et al.Citation66) identified the cis-acting elements within the region, termed xylanase-activating element (XAE). The XAE consists of 5′-GGGTAAATTGG-3′ sequences that contain two adjacent binding sites for Ace2 and Hap2/3/5 complex (the 5′-GGGTAA-3′ sequences on the coding strand for Ace2 and the CCAAT sequence on the non-coding strand for Hap2/3/5 complex). A later study revealed that the in vitro translation product of full-length Xyr1 and Ace2 binds to an inverted repeat motif consisting of a 5′-GGGTAA-3′ and a 5′-GGCTGG-3′ elements separated by a 12-bp spacer within the xyn2 promoter region.Citation51) This binding motif encompasses a part of the nucleotide sequences of XAE. Because two transcription factors bind to the same nucleotide sequences, the authors hypothesized that a regulatory interplay between Xyr1 and Ace2 is responsible for xyn2 expression. Based on these findings, Ace2 has been suggested to act as an antagonist of Xyr1 at the early stage of induction and as an enhancer of a continuous extension of the expression of xyn2.Citation50,51) In addition, a 5′-AGAA-3′ motif immediately upstream of XAE has shown to mediate repression of xyn2 expression under both inducing and repressing conditions. Mach and Zeilinger (2003)Citation49) designated the 5′-AGAA-3′ binding protein(s) as the xylanase repressor protein 2 (Xrp2). The corresponding transcription factor, Xylanase promoter-binding protein 1 (Xpp1), was identified and characterized very recently.Citation55,56)
xyn3 is a third xylanase gene identified by our group from T. reesei PC-3-7.Citation25) Unlike xyn1 and xyn2, both of which encode a glycoside hydrolase (GH) family 11 xylanase, xyn3 is the only gene encoding a GH10 xylanase and contributes to approximately 25% of total xylanase activity in T. reesei PC-3-7.Citation25) Our gene expression profiling study revealed that xyn3 is coordinately expressed with cellulase genes suggesting that the mechanisms regulating xyn3 expression differ from those regulating the two major xylanase genes.Citation46) In addition, we also found that no xyn3 transcripts were formed in T. reesei QM9414 mycelia under any tested inducing conditions, although the same xyn3 structural gene and 5′-upstream region exist in the QM9414 genome.Citation46,92)
To elucidate the molecular basis of this characteristic expression of xyn3, our group has been studying the promoter region of the gene in T. reesei PC-3-7.Citation92,93) Our initial deletion analysis of the 1,071 bp xyn3 upstream region revealed that at least two regulatory regions mediate its carbon source-dependent expression.Citation92) Detailed deletion analysis of the regulatory regions determined that the regulatory elements located on a 50-bp region from −722 to −673 play a critical role in xyn3 expression during induction on α-sophorose and l-sorbose.Citation93) Although no 5′-GGCTAT-3′ motif for Xyr1 or Ace2 was found in this region, similar motifs 5′-GGCTAT-3′ and 5′-GGCAAA-3′ were identified at positions −700 and −673, which form an inverted repeat structure spaced by 16-bp internal sequences. The functionality of these nucleotide motifs in xyn3 expression was investigated by in vivo mutagenesis analysis, which showed that the mutation of the GGC triplet in both the nucleotide motifs led to drastic reductions in reporter gene expression. An additional cis-acting motif involved in this regulation was identified within the region between −331 and −221. Although the 5′-GGCTAT-3′ and 5′-GGCAAA-3′ motifs have a marked influence on the promoter activity, mutation of the 5′-GGCTAA-3′ motif located at position −224 caused a moderate decrease in reporter activity. Furthermore, EMSA using heterologously expressed DNA-binding domain of Xyr1 showed that all the identified cis-acting elements are able to interact with Xyr1. From these results, we concluded that the expression of xyn3 in T. reesei PC-3-7 is regulated by the three cis-acting elements acting as a Xyr1 binding site.
The schematic representation of the promoter structure of xylanase genes is shown in Fig. .
Fig. 6. The structure of the xyn1 (A), xyn2 (B) and xyn3 (C) promoter.
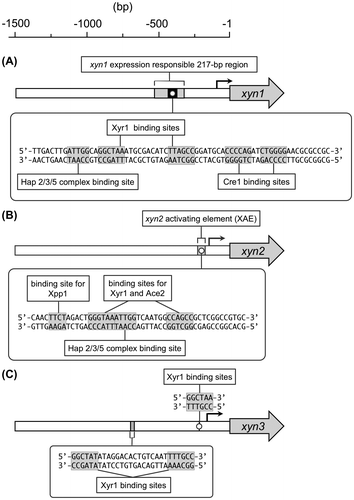
IV. Evaluation and utilization of the promoter of cellulase genes to improve strain for effective biomass saccharification
To date, a number of cellulase-overproducing strains have been derived from the wild-type T. reesei QM6a strain by classical mutagenesis (i.e. UV irradiation or N-methyl-N′-nitro-N′-nitrosoguanidine treatment.Citation94) While random traditional mutagenesis is used extensively, it is unpredictable and does not always produce improved strains. Contemporary recombinant DNA technology, on the other hand, provides more precise methods for obtaining cellulase-overproducing strains.Citation95–99) In T. reesei, maximum extracellular protein production under optimal culture conditions was reported as 30 g/lCitation21) from 10% carbon sources. Considering the limitations of carbon utilization, it may be impossible to prepare cellulase-overproducing strains with further high productivity. Therefore, quality modification of T. reesei cellulase preparations would be pertinent for enzymatic saccharification of cellulosic biomass. In order to construct highly functional cellulase producing strains of T. reesei, it is important to characterize the promoters of the cellulase genes thoroughly because their expressions are coordinated and regulated at the transcriptional level. Due to its exceptionally high capacity for protein production, the T. reesei strains are considered a very desirable host for the production of heterologous proteins. Production of heterologous proteins in Trichoderma is generally much less efficient than production of endogenous proteins.Citation100,101) Generally, a strong cbh1 promoter is used for heterologous protein expression in T. reesei.Citation95–99) However, for the expression of a heterologous gene encoding an effective glycoside hydrolase, the use of cbh1 promoter brings some limitations: (i) the expression construct should be integrated in the genomic location other than the cbh1 locus because CBHI is an essential enzyme for cellulose saccharification, (ii) the titration of the transcription factor may affect the expression of other hydrolase genes, (iii) the importance of the ratio of the expressed heterologous cellulase in the enzyme cocktail should be taken into account. Taking all these into consideration, evaluation of the cbh1, cbh2, egl1, egl3, and xyn3 promoters was carried out using GUS reporter system to select the promoter of T. reesei cellulase and xylanase genes with suitable expression strength for a target gene.Citation102) The cbh1 promoter was the strongest one for GUS production as expected. GUS protein productions by various promoters was found to be correlated with the mRNA levels of the native genes in wild-type T. reesei PC-3-7, with the exception of the cbh2 promoter (Fig. ). This gus expression profiles controlled by the promoter of cbh1, egl1, egl3, and xyn3 in T. reesei PC-3-7 were similar to previously reported results.Citation10) Since these reporter strains were homologous recombinants, each of them can be used as the disruptant for cellulase gene. T. reesei has two cellobiohydrolase genes, cbh1 and cbh2, and their disruptions significantly reduce the total cellulose-degrading ability. Disruption of the major endoglucanase egl1 also brings about reduction of in carboxymethyl-cellulose degradation. However, disruption of egl3 or xyn3, encoding minor glucoside hydrolases, has little effect on the total cellulose-degrading activity in T. reesei PC-3-7. While several promising studies, mostly using the cbh1 promoter, have reported on improved strains possessing high cellulase activity, their filter paper degrading activity or extracellular cellulase production seem to be the same or less than that of the native strains.Citation95–99) Because the disruption of cbh1, cbh2, and egl1 leads to lower cellulase activity, egl3 and xyn3 are better suited promoters for constructing an improved T. reesei strain with cellulase high-saccharifying capacity. In T. reesei, one of the major drawbacks in cellulosic biomass degradation is the low level of BGL activity,Citation103,104) which leads to an incomplete conversion of cellobiose to glucose during the hydrolysis of cellulose. To overcome this disadvantage, endogenous BGLI-overproducing strains were constructed by placing an additional copy of bgl1 under the control of the egl3 or the xyn3 promoter, with the aim of enhancing the cellulase activity of the recombinant T. reesei PC-3-7 strain.Citation105) The recombinant bgl1 mRNA was expressed under cellulase or xylanase promoters as efficiently to match the native mRNA levels of the cellulase or xylanase genes in the host strain. Cellulase preparations from the strain expressing BGLI under the control of egl3 or xyn3 promoter have high saccharifying ability toward cellulosic biomass. Therefore, this concept has been successful in developing a T. reesei stain that produces improved enzyme preparations and is effective in biomass saccharification in Japan (see Chapter 7).
Fig. 7. Comparison of GUS activities controlled cellulase and xylanase promoter and gene expression levels of native cellulase in PC-3-7.
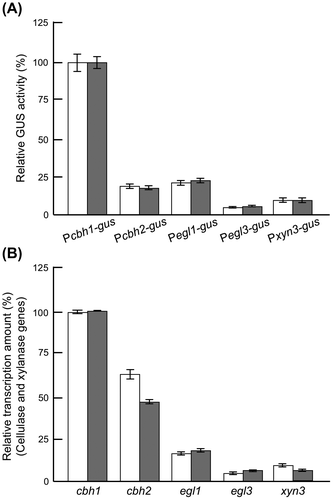
V. Morphological analysis of T. reesei
The molecular basis of T. reesei protein production has been widely analyzed, but a systematic morphological investigation of T. reesei mutants has not been carried out, despite the awareness of a large difference between the wild type and the mutants. For example, colony formation on the plate culture (Fig. ) and the shape of the hyphae in the submerged culture are drastically different between the wild-type strain and the mutants.
In order to determine the possible existence of distinct morphological phenotypes that can be associated with enzyme production capabilities and to understand the difference between mutants, the morphological features of each of the mutant lineage that was developed in Japan were analyzed.Citation106) For the scanning electron microscopy (SEM) observation of the hyphae of the standard strain QM9414 and the mutant strain PC-3-7, we used low-voltage SEM with a non-coating or slightly coating technique developed by Osumi et al.Citation107) Fig. shows the hyphal surface architecture of QM9414 and mutant PC-3-7. Both the strains exhibited a similar surface morphology when grown in glucose culture (Fig. (A and C)). The hyphal surface of both the strains was partially and sparsely covered with a fibrous material. There are a few reports that recorded the appearance of such a fibrous material,Citation108–110) but details of this material are not available in the morphological analysis of filamentous fungi. In contrast to the glucose cultivation, the hyphae of both strains obtained from Avicel cultivation were densely covered with a massive amount of fibrous material (Fig. (B and D)). When the fibrous material in close proximity to the surface of the cell wall was magnified, it seemed as if the fibrous material was fused to the cell wall and a granular material was seen on the cell surface (Fig. ). Although there have not been any experimental evidences, it is possible that the fibrous material synthesized on the cell wall or on the membrane was secreted to the surface of the hypha. Secreted fibrous materials may eventually form a layer by entangling with each other. Previously, studies using immuno-electron microscopic analysis reported that T. reesei produces a fibrous layer called as “exopolysaccharide layer” on the cell wall and showed that it plays a role in trapping the secreted BGLsCitation109) and EGs.Citation108,110) It is probable that the fibrous material enables T. reesei to trap the essential enzymes in the vicinity of the cell wall for effective cellulose degradation. This might be one of the survival strategies of T. reesei. The composition of the fibrous material is not well understood yet and studies are needed in future to characterize this material. The observations of the massive fibrous material produced in the cultivation on crystalline cellulose suggest a correlation between cellulase production and the amount of fibrous material produced.
Fig. 8. Low-voltage SEM images of T. reesei QM9414 and PC-3-7.
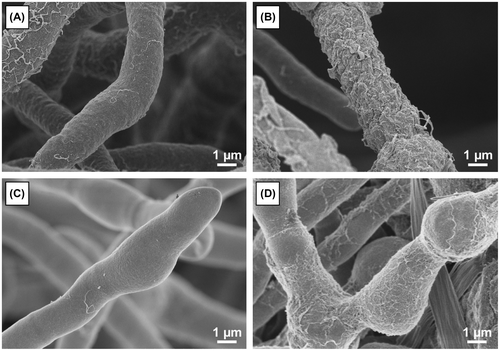
Fig. 9. Hyphal surface structure of T. reesei QM9414.
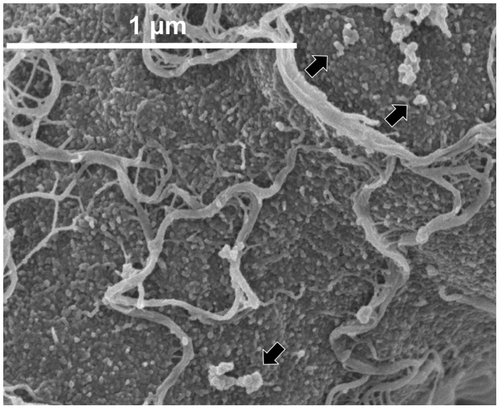
From the viewpoint of hyphal morphology, PC-3-7 formed shorter, swollen, and highly branched hyphae compared to hyphae produced by QM9414. In addition, swollen bead-like cells with an oval shape were observed on the PC-3-7 hyphae. It was obvious that the cellulase-producing hyphae underwent a drastic morphological change in the lineage of the mutant. The transmission electron microscopy (TEM) analysis of ultrathin sections of the T. reesei showed that the hypha of PC-3-7 frequently exhibited long parallel-stacked layers of endoplasmic reticulum (ER) when grown on crystalline cellulose. It has been reported that the proliferation of rough ER in T. reesei hyper-cellulolytic mutants was accompanied by an increased secretion of cellulase.Citation111) In addition to ER, vacuoles of differing sizes were observed to be randomly distributed in the cytoplasm of large numbers of cells in both strains. PC-3-7, in particular, had many vacuoles that were much larger than those of QM9414 (Fig. (A and B)). In contrast to cultivation on cellulose, there are little cytological differences in hyphae of either strain when grown on glucose. A previous report using immuno-electron microscopic observation has shown that xylanases were located in the vacuoles during cellulase production in the high cellulase-producing mutant of T. reesei, the Rut-C30 strain.Citation112) Altogether, these observations and previous reports suggest that the secreted proteins that were not completely folded or those that did not undergo posttranslational modifications might have been transferred to vacuoles.
Fig. 10. TEM observation of T. reesei QM9414 and PC-3-7.
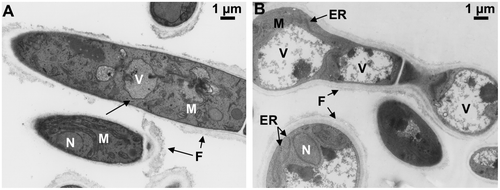
Although T. reesei is extensively studied because of its industrial usefulness, there is a paucity of reports focusing on the morphological features of this strain. However, enough evidence derived from previous morphological analyses suggests that the hyphal morphology and intracellular architecture of these strains render selective advantages resulting in the enhanced enzyme production in T. reesei.
VI. Comparative genomic analysis of T. reesei mutants
The advent of massive parallel sequence technology has enabled the sequencing of large numbers of genomes in a short period of time.Citation113) Next generation sequencing (NGS) technology has made genome-wide identification of polymorphisms among genetic variants of the same or related species possible. Recently, it is used for comparative genomic analysis in whole-genome sequencing studies to identify insertion–deletion mutations (in/del) and SNP in a variety of organisms, including viruses,Citation114) bacteria,Citation115–117) plants,Citation118,119) and humans.Citation120–122) Therefore, a comparison of genetic variants among T. reesei mutants using a high through-put sequencing technology provides important insights into the basis of increased hydrolase production observed after each round of mutagenesis. Recently, the genome of hyper-cellulolytic mutant strains was sequenced using NGS technology.Citation52,53,123–125) In addition, a massive sequence analysis of RNA from T. reesei has also been reported.Citation45,126,127) In Japan, T. reesei mutants have been sequenced by NGS and SNPs that occurred in each mutant were identified (unpublished data). Among them, the PC-3-7 strain was a favorite for performing genome analysis because of the long history of investigation for PC-3-7 in Japan and because it acquired enhanced cellulase inducing ability on l-sorbose and enhanced cellulase production on cellobiose, and, especially, after comparison of the genomes of QM6a and PC-3-7, 260 SNPs were found in the PC-3-7 genome. Among them, 164 SNPs existed in the coding and non-coding regions (promoter, 1 kb upstream of the start codon; terminator, 0.5 kb downstream of the stop codon).Citation26) The majority of the SNPs (68.2%) were located in the non-coding regions, e.g. promoters, terminators, and introns (103 SNPs), and only 31.8% (49 SNPs) occurred in the exons. A majority of these (45 SNPs) were mutations that caused a change in amino acid sequence in the mature protein (missense mutations). Only three SNPs resulted in stop codons (nonsense mutations), and one resulted in a silent mutation.
Interestingly, analysis of the transcription-related genes showed that SNPs were found in the cellulase regulators ace1 and cre1. In ace1, the missense SNP results in a glycine replacement of valine 71 amino acids before the first zinc finger, in a region without a predicted function. In cre1, the SNP resulted in a threonine-to-proline substitution in amino acid 78 of the Cre1 zinc finger domain. Thus, it is possible that if the SNP in cre1 affected Cre1 binding affinity, it could result in significant phenotypic consequences. The gene complementation and deletion analysis of cre1 revealed that PC-3-7 strain is partially released from CCR and that the mutant Cre1 is partially functional in vivo.Citation26)
bglr encoding transcription factor BglR was identified as one of the gene harboring a SNP. The SNP was found to cause a missense mutation close to the end of the DNA binding region of BglR, which turned out to be a Zn2Cys6-type fungal-specific transcription factor.Citation17) Whereas, the disruptant of bglr as well as PC-3-7 exhibited elevated cellulase production during growth on cellobiose, reversion of the SNP missense mutation within bglr to the wild-type allele resulted in reduced cellulase production. Expression of bgls in a bglr gene disruptant was repressed and it exhibited reduced ability to hydrolyze cellobiose even when induced. Therefore, one of the functions of BglR is the upregulation of bgls (with the exception of bgl1, which is seemingly under the direct control of Xyr1). However, the complete function of BglR is still unknown, and analysis of BglR in the standard strain QM9414 is under investigation.
Another notable SNP occurred in bgl2 encoding intracellular β-glucosidase BGLII, giving rise to an amino acid substitution. Since it has been proposed that some BGLs may correlate with inducer formation for cellulase expression, the effects of the BGLII mutation in cellulase induction were analyzed.Citation27) PC-3-7 revertants of bgl2 exhibited reduced expression and inducibility of cellulase during growth on cellulose and cellobiose substrates. Furthermore, the introduction of a bgl2 mutation in the standard strain QM9414 brought about enhanced cellulase production compared to that of PC-3-7. Enzymatic analysis of mutant BGLII exhibited weakened cellobiose hydrolytic activity, but produced some transglycosylation products, suggesting that the SNP in bgl2 strongly diminished the cellobiase activity, but did not result in a complete loss of function of BGLII. The analysis of the recombinant BGLII revealed that major transglycosylation products might be oligosaccharides, composed probably of glucose linked β-1,4, β-1,3 or a mixture of both, and α-sophorose, which was a minor product.
The analysis of cre1, bglr, and bgl2 had brought forward some plausible explanations for the high cellulase-producing ability of PC-3-7 (Fig. ). Extracellular cellobioses are hydrolyzed to glucoses or transglycosylated by extracellular or cell wall-associated BGLs. Glucose causes CCR of cellulase expression. However, since Cre1 controlling CCR is mutated, PC-3-7 is relieved from glucose repression. Alternatively, cellobioses are incorporated in the cell and may be accumulated in the cell because BGLII, the major intracellular BGL of T. reesei, is mutated and therefore possesses significantly reduced hydrolyzing activity. The mutated BGLII still has some residual transglycosylation activity and could generate possible cellulase inducers from cellobiose. Further, BglR mutation causes low expression of intracellular BGLs, and consequently, some low amount of glucose production in the cell. This also might also be the reason for the release from CCR.
Fig. 11. The schematic representation of the model of enhanced cellulase production responsive to cellobiose in T. reesei PC‐3‐7.
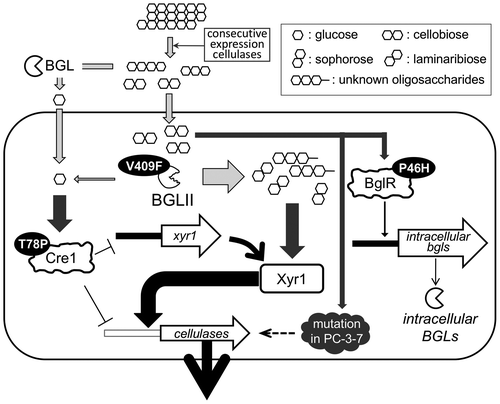
However, in addition to the three mutated gene analyzed in PC-3-7, some other factor(s) that is mutated in the PC-3-7 may also affect the role of cellobiose in cellulase induction because bgl2 disruptant of PC-3-7 still shows cellulase production comparable to PC-3-7. Therefore, other genes with SNPs should be analyzed for a better understanding of the cellulase production by cellobiose.
VII. Construction of a recombinant T. reesei strain for efficient biomass conversion
BGL activity has generally been found to be quite low in cellulase preparations from T. reesei. The construction of a single recombinant T. reesei that produced the full suite of saccharifying enzymes, including BGL, would help in reducing processing costs. Toward this goal, we screened more than 40 species of BGL. Among them, the A. aculeatus AaBG1 was selected as the best BGL because of its high specific activity and efficient expression in T. reesei. In particular, the cellobiase activity of A. aculeatus AaBG1 is 18 times as high as that of T. reesei BGLI. From this point of view, AaBG1 has significant advantages for use in biomass saccharification.
Use of the cbh1 and cbh2 promoters, which are known to be very strong, was found to reduce the overall cellulase activity,Citation102) while the use of xyn3 promoters in GUS constructs did not (See Chapter 4). The relative GUS expression level of the xyn3 promoter in the presence of cellulose was found to be 10% that of the cbh1 promoter.Citation105) Expression in T. reesei using the xyn3 promoter may provide sufficient BGL activity if the low productivity that generally results from heterologous expression can be avoided or overcome.
We constructed X3AB1, a recombinant T. reesei strain that expressed AaBG1 with high specific activity under the control of the xyn3 promoter, to enable the successful cellulase hyper-producing T. reesei mutant to perform efficient saccharification of pretreated cellulosic biomass.Citation128) The culture supernatant, named JN11, from T. reesei X3AB1 grown on 1% Avicel as a carbon source had 63- and 25-fold higher β-glucosidase activity against cellobiose compared to that of the parent strain PC-3-7 and that of the T. reesei recombinant strain expressing an endogenous β-glucosidase I, respectively.
The ability of JN11 to convert cellulosic biomass into fermentable sugars is enhanced by the replacement of xyn3 by A. aculeatus aabg1. However, subsequent experiments using T. reesei extracts supplemented with XYNIII as GH10 xylanase and XYNII as the GH11 xylanase showed increased conversion of alkaline-treated cellulosic biomass, which is rich in xylan, underscoring the importance of XYNIII. To attain the optimal saccharifying potential in T. reesei, we constructed a new strain E1AB1, in which aabg1 was expressed heterologously by means of the egl1 promoter, so that the endogenous XYNIII synthesis remained intact.Citation129) Due to the presence of wild-type xyn3 in T. reesei E1AB1, JN13, the enzyme preparations from this strain, was 20–30% more effective in the saccharification of NaOH-pretreated rice straw than enzyme extracts from X3AB1, and it also outperformed recent commercial cellulase preparations. These results demonstrate the importance of XYNIII in the conversion of NaOH-pretreated cellulosic biomass by T. reesei.
We also compared the cellulase and hemicellulase activities of JN11 and JN13 together with those of the parental strain PC-3-7 and of the commercially available Enzyme A preparation (Table ). Cellobiase activities of JN11 and JN13 were over 60 times of PC-3-7. Xylanase activity of the enzyme preparation JN13H that is derived from the cultivation on the mixture of cellulose and xylan were very high. The enzyme dose for 80% saccharification of JN13H was less than those of JN11 and Enzyme A in the saccharification of NaOH-pretreated Erianthus, while in H2SO4-pretreated Erianthus JN11 is best (Table ). As shown in Table , among the recombinant enzymes and Enzyme A, JN11 is the most suitable enzyme for the saccharification of H2SO4- and physical-pretreated biomass among the recombinant enzymes and Enzyme A, whereas JN13H is the best for Alkaline-pretreated biomass.
Table 2. The cellulase and hemicellulase activities of PC-3-7, JN11, JN13 and the commercially available Enzyme A (U/mg protein).
Table 3. Enzyme amounts for 80% saccharification (mg-protein/g-biomass).
VIII. Conclusions and future prospects
Many investigations have made T. reesei a model fungus for studying the cellulase production mechanism. In T. reesei, the biosynthesis of the cellulolytic and xylanolytic enzymes is modulated by a complex network involving several regulatory proteins at the level of transcription. The transcription factors involved in cellulase and xylanase gene expression have been isolated in T. reesei. However, studies of cellulase gene regulation in T. reesei have been limited to the major cellulase genes, and little is known about how the minor cellulase genes are regulated and how the expression level of each cellulase gene is determined. Therefore, we are far from possessing a comprehensive understanding of cellulase gene expression as a whole. Because cellulase genes are expressed coordinately, information about one can be applied to others. A better understanding of the relevant regulatory mechanisms is expected to lead to improvements in controlling cellulase gene expression that will result in better cellulosic biomass degradation. Our comparative genomic analysis showed not only a path to engineer T. reesei for industrial use, but also that mutants developed by our great predecessors have the keys to solve the regulatory puzzle of T. reesei cellulase induction. Effort are on the way for developing the next generation recombinant T. reesei strain suitable for cellulosic biomass saccharification using all the information available from studies on gene regulation, comparative genomic analysis and screening of the suitable enzyme.
Acknowledgment
The authors thank all persons listed below who correlate with our investigations described in this review. Dr Yasushi Morikawa, emeritus professor from Nagaoka University of Technology for valuable advice for all investigation of our laboratory. the late Dr Hirofumi Okada from Nagaoka University of Technology for valuable comments on the mechanism of cellulase and xylanase induction in T. reesei. Dr Satoshi Nakagawa, Dr Hiroaki Motoyama and Mr Yoshiyuki Yonetani from Kyowa Hakko Bio Co. Ltd. for providing the information of T. reesei mutants developed in Japan. Prof. Satoru Kuhara, Assoc. Prof. Kosuke Tashiro, and Dr Kazuki Mori from Kyusyu University and Dr Hirakawa Hideki from Kazusa DNA Res. Inst. for their valuable comments on comparative genomic analysis. Dr Masako Osumi, emeritus professor from Japan Women’s University for technical assistance and valuable advice of the microscopic analysis. Prof. Takashi Kawaguch, Assoc. Prof. Jun-ichi Sumitani and Assist. Prof. Shuji Tani, from Osaka Prefecture University for providing enzyme with its enzymatic information. Dr Zinnia Rahman, Dr Mikiko Nitta, Dr Hikaru Nakazawa, Dr Masahiro Yuki, Dr Juliano de O. Porciuncula, Dr Yoshiyuki Suzuki, Dr Machiko Takahashi, Dr Treesukon Treebupachatsakul, Mr Hiroki Hirasawa, Mr Shingo Tahara, Ms Nayani D. Daranagama, Ms Kaori Yamaguchi, Mr Naoki Suda, Mr Hiroki Kitagami, Ms Yuki Ohta, Ms Yota Suzuki, Mr Ryuichi Shimada, and Ms Shiho Nakagawa for studying at our laboratory. We are deeply grateful to many other members for this study. A part of research described in this review was supported by Grants-in-Aid for Scientific Research from Ministry of Education, Culture, Sports, Science and Technology (MEXT), Japan, by a SATREPS Grant from Japan Science and Technology Agency (JST) and Japan International Cooperation Agency (JICA), and by New Energy and Industrial Technology Development Organization (NEDO).
References
- Gilbert HJ, Hazlewood GP. Bacterial cellulases and xylanases. J. Gen. Microbiol. 1993;139:187–194.10.1099/00221287-139-2-187
- Goyal A, Ghosh B. Characteristics of fungal cellulases. Biores. Technol. 1991;36:37–50.10.1016/0960-8524(91)90098-5
- Watanabe H, Noda H, Tokuda G, et al. A cellulase gene of termite origin. Nature. 1998;394:330–331.10.1038/28527
- Harris PV, Welner D, McFarland KC, et al. Stimulation of lignocellulosic biomass hydrolysis by proteins of glycoside hydrolase family 61: structure and function of a large, enigmatic family. Biochemistry. 2010;49:3305–3316.10.1021/bi100009p
- Morgenstern I, Powlowski J, Tsang A. Fungal cellulose degradation by oxidative enzymes: from dysfunctional GH61 family to powerful lytic polysaccharide monooxygenase family. Brief. Funct. Genomics. 2014;13:471–481.10.1093/bfgp/elu032
- Mandels M, Reese ET. Induction of cellulase in Trichoderma viride as influenced by carbon sources and metals. J. Bacteriol. 1957;73:269–278.
- Bisaria VS, Mishra S. Regulatory aspects of cellulase biosynthesis and secretion. Crit. Rev. Biotechnol. 1989;9:61–103.10.3109/07388558909040616
- Kubicek CP, Messner R, Gruber F, et al. The trichoderma cellulase regulatory puzzle: from the interior life of a secretory fungus. Enz. Microb. Technol. 1993;15:90–99.10.1016/0141-0229(93)90030-6
- Ilmén M, Saloheimo A, Onnela M-L, et al. Regulation of cellulase gene expression in the filamentous fungus Trichoderma reesei. Appl. Environ. Microbiol. 1997;63:1298–1306.
- Nogawa M, Goto M, Okada H, et al. L-Sorbose induces cellulase gene transcription in the cellulolytic fungus Trichoderma reesei. Curr. Genet. 2001;38:329–334.10.1007/s002940000165
- Stricker AR, Grosstessner-Hain K, Würleitner E, et al. Xyr1 (Xylanase Regulator 1) regulates both the hydrolytic enzyme system and D-xylose metabolism in Hypocrea jecorina. Eukaryot. Cell. 2006;5:2128–2137.10.1128/EC.00211-06
- Rauscher R, Würleitner E, Wacenovsky C, et al. Transcriptional regulation of xyn1, encoding xylanase I, in Hypocrea jecorina. Eukaryot. Cell. 2006;5:447–456.10.1128/EC.5.3.447-456.2006
- Aro N, Saloheimo A, Ilmén M, et al. ACEII, a novel transcriptional activator involved in regulation of cellulase and xylanase genes of Trichoderma reesei. J. Biol. Chem. 2001;276:24309–24314.10.1074/jbc.M003624200
- Häkkinen M, Valkonen MJ, Westerholm-Parvinen A, et al. Screening of candidate regulators for cellulase and hemicellulase production in Trichoderma reesei and identification of a factor essential for cellulase production. Biotechnol. Biofuels. 2014;7:14.10.1186/1754-6834-7-14
- Saloheimo A, Aro N, Ilmén M, et al. Isolation of the ace1 gene encoding a Cys2-His2 transcription factor involved in regulation of activity of the cellulase promoter cbh1 of Trichoderma reesei. J. Biol. Chem. 2000;275:5817–5825.10.1074/jbc.275.8.5817
- Aro N, Ilmen M, Saloheimo A, et al. ACEI of Trichoderma reesei is a repressor of cellulase and xylanase expression. Appl. Environ. Microbiol. 2003;69:56–65.10.1128/AEM.69.1.56-65.2003
- Nitta M, Furukawa T, Shida Y, et al. A new Zn(II)2Cys6-type transcription factor BglR regulates β-glucosidase expression in Trichoderma reesei. Fungal Genet. Biol. 2012;49:388–397.10.1016/j.fgb.2012.02.009
- Strauss J, Mach RL, Zeilinger S, et al. Crel, the carbon catabolite repressor protein from Trichoderma reesei. FEBS Lett. 1995;376:103–107.10.1016/0014-5793(95)01255-5
- Takashima S, Iikura H, Nakamura A, et al. Analysis of Cre1 binding sites in the Trichoderma reesei cbh1 upstream region. FEMS Microbiol. Lett. 1996;145:361–366.10.1111/fml.1996.145.issue-3
- Ilmén M, Thrane C, Penttilä M. The glucose repressor gene cre1 of Trichoderma: isolation and expression of a full-length and a truncated mutant form. Mol. Gen. Genet. 1996;251:451–460.
- Herpoël-Gimbert I, Margeot A, Dolla A, et al. Comparative secretome analyses of two Trichoderma reesei RUT-C30 and CL847 hypersecretory strains. Biotechnol. Biofuels. 2008;1:18.10.1186/1754-6834-1-18
- Mandels M. Microbial sources of cellulase. Biotechnol Bioeng. 1975;5:81–105.
- Mäntylä AL, Rossi KH, Vanhanen SA, et al. Electrophoretic karyotyping of wild-type and mutant Trichoderma longibrachiatum (reesei) strains. Curr. Genet. 1992;21:471–477.10.1007/BF00351657
- Kawamori M, Morikawa Y, Takasawa S. Induction and production of cellulases by L-sorbose in Trichoderma reesei. Appl. Microbiol. Biotechnol. 1986;24:449–453.
- Xu J, Takakuwa N, Nogawa M, et al. A third xylanase from Trichoderma reesei PC-3-7. Appl. Microbiol. Biotechnol. 1998;49:718–724.10.1007/s002530051237
- Porciuncula JDO, Furukawa T, Mori K, et al. Single nucleotide polymorphism analysis of a Trichoderma reesei hyper-cellulolytic mutant developed in Japan. Biosci. Biotechnol. Biochem. 2013;77:534–543.10.1271/bbb.120794
- Shida Y, Yamaguchi K, Nitta M, et al. The impact of a single-nucleotide mutation of bgl2 on cellulase induction in a Trichoderma reesei mutant. Biotechnol. Biofuels. 2015;8:3305.10.1186/s13068-015-0420-y
- Demain AL. Biosolutions to the energy problem. J. Ind. Microbiol. Biotechnol. 2009;36:319–332.10.1007/s10295-008-0521-8
- Gray KA, Zhao L, Emptage M. Bioethanol. Curr. Opin. Chem. Biol. 2006;10:141–146.10.1016/j.cbpa.2006.02.035
- Mussatto SI, Dragone G, Guimarã PM, et al. Technological trends, global market, and challenges of bio-ethanol production. Biotechnol. 2010;28:817–e830.
- Saloheimo M, Kuja-Panula J, Ylosmaki E, et al. Enzymatic properties and intracellular localization of the novel Trichoderma reesei beta-glucosidase BGLII (Cel1A). Appl. Environ. Microbiol. 2002;68:4546–4553.10.1128/AEM.68.9.4546-4553.2002
- Du F, Wolger E, Wallace L, et al. Determination of product inhibition of CBH1, CBH2, and EG1 using a novel cellulase activity assay. Appl. Biochem. Biotechnol. 2010;161:313–317.10.1007/s12010-009-8796-4
- Kubicek CP, Penttilä M. Regulation of production of plant polysaccharide degrading enzymes by Trichoderma. In: Harman GE, Kubicek CP, editors. Trichoderma and Gliocladium, Enzymes, biological control and commercial applications. Vol 2, Gunpowder Square: London; 1998. p. 49–72.
- Margolles-Clark E, Tenkanen M, Nakari-Setala T, et al. Cloning of genes encoding alpha-L-arabinofuranosidase and beta-xylosidase from Trichoderma reesei by expression in Saccharomyces cerevisiae. Appl. Environ. Microbiol. 1996;62:3840–3846.
- el-Gogary S, Leite A, Crivellaro O, et al. Mechanism by which cellulose triggers cellobiohydrolase I gene expression in Trichoderma reesei. Proc. Natl. Acad. Sci. 1989;86:6138–6141.10.1073/pnas.86.16.6138
- Carle-Urioste JC, Escobar-Vera J, El-Gogary S, et al. Cellulase induction in Trichoderma reesei by cellulose requires its own basal expression. J. Biol. Chem. 1997;272:10169–10174.
- Aro N, Pakula T, Penttilä M. Transcriptional regulation of plant cell wall degradation by filamentous fungi. FEMS Microbiol. Rev. 2005;29:719–739.10.1016/j.femsre.2004.11.006
- Kubicek CP, Messner R, Gruber F, et al. Triggering of cellulase biosynthesis by cellulose in Trichoderma reesei. Involvement of a constitutive, sophorose-inducible, glucose-inhibited beta-diglucoside permease. J. Biol. Chem. 1993;268:19364–19368.
- Fowler T, Brown RD. The bgl1 gene encoding extracellular beta-glucosidase from Trichoderma reesei is required for rapid induction of the cellulase complex. Mol. Microbiol. 1992;6:3225–3235.10.1111/mmi.1992.6.issue-21
- Fritscher C, Messner R. Cellobiose metabolism and cellobiohydrolase I biosynthesis by Trichoderma reesei. Exp. Mycol. 1990;14:405–415.10.1016/0147-5975(90)90063-Y
- Sternberg D, Mandels GR. Induction of cellulolytic enzymes in Trichoderma reesei by sophorose. J. Bacteriol. 1979;139:761–769.
- Vaheri M, Leisola M, Kauppinen V. Transglycosylation products of cellulase system of Trichoderma reesei. Biotechnol. Lett. 1979;1:41–46.10.1007/BF01395789
- Mach RL, Seiboth B, Myasnikov A, et al. The bgl1 gene of Trichoderma reesei QM9414 encodes an extracellular, cellulose-inducible beta-glucosidase involved in cellulase induction by sophorose. Mol. Microbiol. 1995;16:687–697.10.1111/mmi.1995.16.issue-4
- Furukawa T, Shida Y, Kitagami N, et al. Identification of specific binding sites for XYR1, a transcriptional activator of cellulolytic and xylanolytic genes in Trichoderma reesei. Fungal Genet. Biol. 2009;46:564–574.10.1016/j.fgb.2009.04.001
- Zhou Q, Xu J, Kou Y, et al. Differential involvement of β-glucosidases from Hypocrea jecorina in rapid induction of cellulase genes by cellulose and cellobiose. Eukaryot. Cell. 2012;11:1371–1381.10.1128/EC.00170-12
- Xu J, Nogawa M, Okada H, Morikawa Y. Regulation of xyn3 gene expression in Trichoderma reesei PC-3-7. Appl. Microbiol. Biotechnol. 2000;54:370–375.10.1007/s002530000410
- Mach RL, Strauss J, Zeilinger S, et al. Carbon catabolite repression of xylanase I (xyn1) gene expression in Trichoderma reesei. Mol. Microbiol. 1996;21:1273–1281.10.1046/j.1365-2958.1996.00094.x
- Zeilinger S, Mach RL, Schindler M, et al. Different inducibility of expression of the two xylanase genes xyn1 and xyn2 in Trichoderma reesei. J. Biol. Chem. 1996;271:25624–25629.10.1074/jbc.271.41.25624
- Mach RL, Zeilinger S. Regulation of gene expression in industrial fungi: Trichoderma. Appl. Microbiol. Biotechnol. 2003;60:515–522.10.1007/s00253-002-1162-x
- Stricker AR, Steiger MG, Mach RL. Xyr1 receives the lactose induction signal and regulates lactose metabolism in Hypocrea jecorina. FEBS Lett. 2007;581:3915–3920.10.1016/j.febslet.2007.07.025
- Stricker AR, Trefflinger P, Aro N, et al. Role of Ace2 (Activator of Cellulases 2) within the xyn2 transcriptosome of Hypocrea jecorina. Fungal Genet. Biol. 2008;45:436–445.10.1016/j.fgb.2007.08.005
- Lichius A, Bidard F, Buchholz F, et al. Genome sequencing of the Trichoderma reesei QM9136 mutant identifies a truncation of the transcriptional regulator XYR1 as the cause for its cellulase-negative phenotype. BMC Genomics. 2015;16:326.10.1186/s12864-015-1526-0
- Derntl C, Gudynaite-Savitch L, Calixte S, et al. Mutation of the Xylanase regulator 1 causes a glucose blind hydrolase expressing phenotype in industrially used Trichoderma strains. Biotechnol. Biofuels. 2013;6:62.10.1186/1754-6834-6-62
- Mach-Aigner AR, Pucher ME, Steiger MG, et al. Transcriptional Regulation of xyr1, encoding the main regulator of the xylanolytic and cellulolytic enzyme system in Hypocrea jecorina. Appl. Environ. Microbiol. 2008;74:6554–6562.10.1128/AEM.01143-08
- Mach-Aigner AR, Grosstessner-Hain K, Poças-Fonseca MJ, et al. From an electrophoretic mobility shift assay to isolated transcription factors: a fast genomic-proteomic approach. BMC Genomics. 2010;11:1–10.
- Derntl C, Rassinger A, Srebotnik E, et al. Xpp1 regulates the expression of xylanases, but not of cellulases in Trichoderma reesei. Biotechnol. Biofuels. 2015;8:1–11.
- Aro N, Saloheimo A, Ilmén M, et al. ACEII, a novel transcriptional activator involved in regulation of cellulase and xylanase genes of Trichoderma reesei. J. Biol. Chem. 2001;276:24309–24314.10.1074/jbc.M003624200
- MacPherson S, Larochelle M, Turcotte B. A fungal family of transcriptional regulators: the zinc cluster proteins. Microbiol. Mol. Biol. Rev. 2006;70:583–604.10.1128/MMBR.00015-06
- Chilton IJ, Delaney CE, Barham-Morris J, et al. The Aspergillus nidulans stress response transcription factor StzA is ascomycete-specific and shows species-specific polymorphisms in the C-terminal region. Mycol. Res. 2008;112:1435–1446.10.1016/j.mycres.2008.06.028
- O'Neil JD, Bugno M, Stanley MS, et al. Cloning of a novel gene encoding a C2H2 zinc finger protein that alleviates sensitivity to abiotic stresses in Aspergillus nidulans. Mycol. Res. 2002;106:491–498.10.1017/S0953756202005701
- Cziferszky A, Mach RL, Kubicek CP. Phosphorylation positively regulates DNA binding of the carbon catabolite repressor Cre1 of Hypocrea jecorina (Trichoderma reesei). J. Biol. Chem. 2002;277:14688–14694.10.1074/jbc.M200744200
- Espeso EA, Peñalva MA. In vitro binding of the two-finger repressor CreA to several consensus and non-consensus sites at the ipnA upstream region is context dependent. FEBS Lett. 1994;342:43–48.10.1016/0014-5793(94)80581-4
- Kulmburg P, Mathieu M, Dowzer C, et al. Specific binding sites in the alcR and alcA promoters of the ethanol regulon for the CREA repressor mediating carbon catabolite repression in Aspergillus nidulans. Mol. Microbiol. 1993;7:847–857.10.1111/mmi.1993.7.issue-6
- Cubero B, Scazzocchio C. Two different, adjacent and divergent zinc finger binding sites are necessary for CREA-mediated carbon catabolite repression in the proline gene cluster of Aspergillus nidulans. EMBO J. 1994;13:407–415.
- Zeilinger S, Mach RL, Kubicek CP. Two adjacent protein binding motifs in the cbh2 (cellobiohydrolase II-encoding) promoter of the fungus Hypocrea jecorina (Trichoderma reesei) cooperate in the induction by cellulose. J. Biol. Chem. 1998;273:34463–34471.10.1074/jbc.273.51.34463
- Würleitner E, Pera L, Wacenovsky C, et al. Transcriptional Regulation of xyn2 in Hypocrea jecorina. Eukaryot. Cell. 2003;2:150–158.10.1128/EC.2.1.150-158.2003
- Ilmén M, Onnela ML, Klemsdal S, et al. Functional analysis of the cellobiohydrolase I promoter of the filamentous fungus Trichoderma reesei. Mol. Gen. Genet. 1996;253:303–314.
- Margolles-clark E, Ilmén M, Penttilä M. Expression patterns of ten hemicellulase genes of the filamentous fungus Trichoderma reesei on various carbon sources. J. Biotechnol. 1997;57:167–179.10.1016/S0168-1656(97)00097-7
- Denton JA, Kelly JM. Disruption of Trichoderma reesei cre2, encoding an ubiquitin C-terminal hydrolase, results in increased cellulase activity. BMC Biotechnol. 2011;11:103.10.1186/1472-6750-11-103
- Brakhage AA, Andrianopoulos A, Kato M, et al. HAP-Like CCAAT-binding complexes in filamentous fungi: implications for biotechnology. Fungal Genet. Biol. 1999;27:243–252.10.1006/fgbi.1999.1136
- Tsukagoshi N, Kobayashi T, Kato M. Regulation of the amylolytic and(hemi-)cellulolytic genes in aspergilli. J. Gen. Appl. Microbiol. 2001;47:1–19.10.2323/jgam.47.1
- Kato M, Aoyama A, Kobayashi T., et al. An Aspergillus nidulans nuclear protein, AnCP, involved in enhancement of Taka-amylase A gene expression, binds to the CCAAT-containing taaG2, amdS, and gatA promoters. Mol. Gen. Genet. 1997;254:119–126.10.1007/s004380050399
- Zeilinger S, Ebner A, Marosits T, et al. The Hypocrea jecorina HAP 2/3/5 protein complex binds to the inverted CCAAT-box (ATTGG) within the cbh2 (cellobiohydrolase II-gene) activating element. Mol. Genet. Genomics. 2001;266:56–63.
- Périer RC, Praz V, Junier T, et al. The eukaryotic promoter database (EPD). Nucleic Acids. Res. 2000;28:302–303.10.1093/nar/28.1.302
- Pinkham JL, Guarente L. Cloning and molecular analysis of the HAP2 locus: a global regulator of respiratory genes in Saccharomyces cerevisiae. Mol Cell Biol. 1985;5:3410–3416.10.1128/MCB.5.12.3410
- McNabb DS, Xing Y, Guarente L. Cloning of yeast HAP5: a novel subunit of a heterotrimeric complex required for CCAAT binding. Genes. Dev. 1995;9:47–58.10.1101/gad.9.1.47
- Narendja FM, Davis MA, Hynes MJ. AnCF, the CCAAT binding complex of Aspergillus nidulans, is essential for the formation of a DNase I-hypersensitive site in the 5′ region of the amdS gene. Mol. Cell Biol. 1999;19:6523–6531.10.1128/MCB.19.10.6523
- Zeilinger S, Schmoll M, Pail M, et al. Nucleosome transactions on the Hypocrea jecorina (Trichoderma reesei) cellulase promoter cbh2 associated with cellulase induction. Mol. Genet. Genomics. 2003;270:46–55.10.1007/s00438-003-0895-2
- Negrete-Urtasun S, Denison SH, Arst HN Jr. Characterization of the pH signal transduction pathway gene palA of Aspergillus nidulans and identification of possible homologs. J. Bacteriol. 1997;179:1832–1835.
- Trevisan GL, Oliveira EH, Peres NT, et al. Transcription of Aspergillus nidulans pacC is modulated by alternative RNA splicing of palB. FEBS Lett. 2011;585:3442–3445.10.1016/j.febslet.2011.09.037
- Galindo A, Hervás-Aguilar A, Rodríguez-Galán O, et al. PalC, one of two Bro1 domain proteins in the fungal pH signalling pathway, localizes to cortical structures and binds Vps32. Traffic. 2007;8:1346–1364.10.1111/j.1600-0854.2007.00620.x
- Hervás-Aguilar A, Galindo A, Peñalva MA. Receptor-independent Ambient pH signaling by ubiquitin attachment to fungal arrestin-like PalF. J. Biol. Chem. 2010;285:18095–18102.10.1074/jbc.M110.114371
- Calcagno-Pizarelli AM, Negrete-Urtasun S, Denison SH, et al. Establishment of the Ambient pH signaling complex in Aspergillus nidulans: PalI assists plasma membrane localization of PalH. Eukaryot. Cell. 2007;6:2365–2375.10.1128/EC.00275-07
- Tilburn J, Sarkar S, Widdick DA, et al. The Aspergillus PacC zinc finger transcription factor mediates regulation of both acid- and alkaline-expressed genes by ambient pH. EMBO J. 1995;14:779–790.
- He R, Ma L, Li C, et al. Trpac1, a pH response transcription regulator, is involved in cellulase gene expression in Trichoderma reesei. Enz. Microb. Technol. 2014;67:17–26.10.1016/j.enzmictec.2014.08.013
- Häkkinen M, Sivasiddarthan D, Aro N, et al. The effects of extracellular pH and of the transcriptional regulator PACI on the transcriptome of Trichoderma reesei. Microb. Cell Fact. 2015;14:63.10.1186/s12934-015-0247-z
- Henrique-Silva F, El-Gogary S, Carle-Urioste JC, et al. Two regulatory regions controlling basal and cellulose-induced expression of the gene encoding cellobiohydrolase I of Trichoderma reesei are adjacent to its TATA box. Biochem. Biophys. Res. Commun. 1996;228:229–237.10.1006/bbrc.1996.1646
- Ling M, Qin Y, Li N, et al. Binding of two transcriptional factors, Xyr1 and ACEI, in the promoter region of cellulase cbh1 gene. Biotechnol. Lett. 2009;31:227–231.10.1007/s10529-008-9857-4
- Stangl H, Gruber F, Kubicek CP. Characterization of the Trichoderma reesei cbh2 promoter. Curr. Genet. 1993;23:115–122.10.1007/BF00352009
- Schmoll M, Kubicek CP. Regulation of Trichoderma cellulase formation: lessons in molecular biology from an industrial fungus. A review. Acta Microbiol. Immunol. Hung. 2003;50:125–145.10.1556/AMicr.50.2003.2-3.3
- Shida Y, Furukawa T, Ogasawara W, et al. Functional analysis of the egl3 upstream region in filamentous fungus Trichoderma reesei. Appl. Microbiol. Biotechnol. 2008;78:515–524.10.1007/s00253-007-1338-5
- Ogasawara W, Shida Y, Furukawa T, et al. Cloning, functional expression and promoter analysis of xylanase III gene from Trichoderma reesei. Appl. Microbiol. Biotechnol. 2006;72:995–1003.10.1007/s00253-006-0365-y
- Furukawa T, Shida Y, Kitagami N, et al. Identification of the cis-acting elements involved in regulation of xylanase III gene expression in Trichoderma reesei PC-3-7. Fungal Genet. Biol. 2008;45:1094–1102.10.1016/j.fgb.2008.03.006
- Nevalainen H, Suominen P, Taimisto K. On the safety of Trichoderma reesei. J. Biotechnol. 1994;37:193–200.10.1016/0168-1656(94)90126-0
- Wang TH, Liu T, Wu ZH, et al. Novel cellulase profile of Trichoderma reesei strains constructed by cbh1 gene replacement with eg3 gene expression cassette. Acta Biochim. Biophys. Sin. 2004;36:667–672.10.1093/abbs/36.10.667
- Miettinen-Oinonen A, Suominen P. Enhanced production of Trichoderma reesei endoglucanases and use of the new cellulase preparations in producing the stonewashed effect on denim fabric. Appl. Environ. Microbiol. 2002;68:3956–3964.10.1128/AEM.68.8.3956-3964.2002
- Miettinen-Oinonen A, Paloheimo M, Lantto R, et al. Enhanced production of cellobiohydrolase in Trichoderma reesei and evaluation of the new preparations in biofinishing of cotton. J. Biotechnol. 2004;116:305–317.
- Mäntylä A, Paloheimo M, Hakola S, et al. Production in Trichoderma reesei of three xylanases from Chaetomium thermophilum: a recombinant thermoxylanase for biobleaching of kraft pulp. Appl. Microbiol. Biotechnol. 2007;76:377–386.10.1007/s00253-007-1020-y
- Paloheimo M, Mäntylä A, Kallio J, et al. Increased production of xylanase by expression of a truncated version of the xyn11A gene from Nonomuraea flexuosa in Trichoderma reesei. Appl. Environ. Microbiol. 2007;73:3215–3224.10.1128/AEM.02967-06
- Keränen S, Penttilä M. Production of recombinant proteins in the filamentous fungus Trichoderma reesei. Curr. Opin. Biotechnol. 1995;6:534–537.10.1016/0958-1669(95)80088-3
- Penttilä M. Heterologous protein production in the Trichoderma. In: Harman GE, Kubicek CP, editors. Trichoderma and Gliocladium. Gunpowder Square: London; 1998. p. 365–382.
- Rahman Z, Shida Y, Furukawa T, et al. Evaluation and characterization of Trichoderma reesei cellulase and xylanase promoters. Appl. Microbiol. Biotechnol. 2009;82:899–908.10.1007/s00253-008-1841-3
- Duff SJB, Murray WD. Bioconversion of forest products industry waste cellulosics to fuel ethanol: a review. Biores. Technol. 1996;55:1–33.10.1016/0960-8524(95)00122-0
- Nieves RA, Ehrman CI, Adney WS, et al. Survey and analysis of commercial cellulase preparations suitable for biomass conversion to ethanol. World J. Microbiol. Biotechnol. 1998;14:301–304.
- Rahman Z, Shida Y, Furukawa T, et al. Application of Trichoderma reesei cellulase and xylanase promoters through homologous recombination for enhanced production of extracellular β-glucosidase I. Biosci Biotechnol Biochem. 2009;73:1083–1089.10.1271/bbb.80852
- Nitta M, Shida Y, Okada H, et al. Hyphal surface architecture and cell morphology of Trichoderma reesei. J. Electron Microsc. (Tokyo). 2012;61:187–192.
- Osumi M, Yamada N, Yaguchi H, et al. Ultrahigh-resolution low-voltage SEM reveals ultrastructure of the glucan network formation from fission yeast protoplast. J. Electron Microsc. 1995;44:198–206.
- Chapman CM, Loewenberg JR, Schaller MJ, et al. Ultrastructural localization of cellulase in Trichoderma reesei using immunocytochemistry and enzyme cytochemistry. J. Histochem. Cytochem. 1983;31:1363–1366.10.1177/31.12.6195212
- Sprey B. Localisation of β-glucosidase in Trichoderma reesei cell walls with immunoelectron microscopy. FEMS Microbiol. Lett. 1986;36:287–292.
- Sprey B. Cellular and extracellular localization of endocellulase in Trichoderma reesei. FEMS Microbiol. Lett. 1988;55:283–294.10.1111/fml.1988.55.issue-3
- Ghosh A, Ghosh BK, Trimino-Vazquez H, et al. Cellulase secretion from a hyper-cellulolytic mutant of Trichoderma reesei Rut-C30. Arch. Microbiol. 1984;140:126–133.10.1007/BF00454914
- Kurzątkowski W, Solecka J, Filipek J, et al. Ultrastructural localization of cellular compartments involved in secretion of the low molecular weight, alkaline xylanase by Trichoderma reesei. Arch. Microbiol. 1993;159:417–422.10.1007/BF00288587
- Mardis ER. The impact of next-generation sequencing technology on genetics. Trends Genet. 2008;24:133–141.10.1016/j.tig.2007.12.007
- Spatz SJ, Rue CA. Sequence determination of a mildly virulent strain (CU-2) of Gallid herpesvirus type 2 using 454 pyrosequencing. Virus Genes. 2008;36:479–489.10.1007/s11262-008-0213-5
- Holt KE, Parkhill J, Mazzoni CJ, et al. High-throughput sequencing provides insights into genome variation and evolution in Salmonella Typhi. Nat. Genet. 2008;40:987–993.10.1038/ng.195
- Kotewicz ML, Mammel MK, LeClerc JE, et al. Optical mapping and 454 sequencing of Escherichia coli O157: H7 isolates linked to the US 2006 spinach-associated outbreak. Microbiology. 2008;154:3518–3528.10.1099/mic.0.2008/019026-0
- Manning SD, Motiwala AS, Springman AC, et al. Variation in virulence among clades of Escherichia coli O157:H7 associated with disease outbreaks. Proc. Natl. Acad. Sci. USA. 2008;105:4868–4873.10.1073/pnas.0710834105
- Novaes E, Drost DR, Farmerie WG, et al. High-throughput gene and SNP discovery in Eucalyptus grandis, an uncharacterized genome. BMC Genomics. 2008;9:312.10.1186/1471-2164-9-312
- Sadia B, Craig J, Hudson M, et al. Genomic DNA sequence comparison between two inbred soybean cyst nematode biotypes facilitated by massively parallel 454 micro-bead sequencing. Mol. Genet. Genomics. 2008;279:535–543.
- Qi W, Käser M, Röltgen K, et al. Genomic diversity and evolution of Mycobacterium ulcerans revealed by next-generation sequencing. PLoS Pathog. 2009;5:e1000580.10.1371/journal.ppat.1000580
- Wang J, Wang W, Li R, et al. The diploid genome sequence of an Asian individual. Nature. 2008;456:60–65.10.1038/nature07484
- Wheeler DA, Srinivasan M, Egholm M, et al. The complete genome of an individual by massively parallel DNA sequencing. Nature. 2008;452:872–876.10.1038/nature06884
- Marie-Nelly H, Marbouty M, Cournac A, et al. High-quality genome (re)assembly using chromosomal contact data. Nat. Commun. 2014;5:5695.10.1038/ncomms6695
- Le Crom S, Schackwitz W, Pennacchio L, et al. Tracking the roots of cellulase hyperproduction by the fungus Trichoderma reesei using massively parallel DNA sequencing. Proc. Natl. Acad. Sci. USA. 2009;106:16151–16156.10.1073/pnas.0905848106
- Koike H, Aerts A, LaButti K, et al. Comparative genomics analysis of Trichoderma reesei strains. Ind. Biotechnol. 2013;9:352–367.10.1089/ind.2013.0015
- Ries L, Pullan ST, Delmas S, et al. Genome-wide transcriptional response of Trichoderma reesei to lignocellulose using RNA sequencing and comparison with Aspergillus niger. BMC Genomics. 2013;14:541.10.1186/1471-2164-14-541
- Kang K, Zhong J, Jiang L, et al. Identification of microRNA-Like RNAs in the filamentous fungus Trichoderma reesei by solexa sequencing. PLoS ONE. 2013;8:e76288.10.1371/journal.pone.0076288
- Nakazawa H, Kawai T, Ida N, et al. Construction of a recombinant Trichoderma reesei strain expressing Aspergillus aculeatus β-glucosidase 1 for efficient biomass conversion. Biotechnol. Bioeng. 2012;109:92–99.10.1002/bit.v109.1
- Nakazawa H, Kawai T, Ida N, et al. A high performance Trichoderma reesei strain that reveals the importance of xylanase III in cellulosic biomass conversion. Enzyme Microb. Technol. 2016;82:89–95.10.1016/j.enzmictec.2015.08.019