Abstract
In high-quality sake brewing, the cerulenin-resistant sake yeast K1801 with high ethyl caproate-producing ability has been used widely; however, K1801 has a defective spindle assembly checkpoint (SAC). To identify the mutation causing this defect, we first searched for sake yeasts with a SAC-defect like K1801 and found that K13 had such a defect. Then, we searched for a common SNP in only K1801 and K13 by examining 15 checkpoint-related genes in 23 sake yeasts, and found 1 mutation, R48P of Cdc55, the PP2A regulatory B subunit that is important for the SAC. Furthermore, we confirmed that the Cdc55-R48P mutation was responsible for the SAC-defect in K1801 by molecular genetic analyses. Morphological analysis indicated that this mutation caused a high cell morphological variation. But this mutation did not affect the excellent brewing properties of K1801. Thus, this mutation is a target for breeding of a new risk-free K1801 with normal checkpoint integrity.
Graphical abstract
K1801 (triploid) having 2 copies of R48P-type cdc55 and 1 copy of R48-type cdc55 showed a defective spindle assembly checkpoint (SAC). Cdc55-R48P is semidominant mutation in the SAC function.
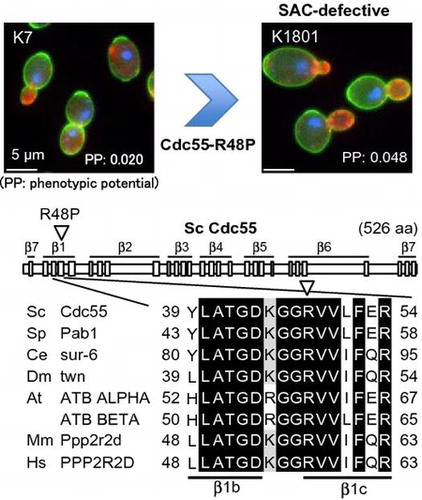
Ethyl caproate, a major flavor component in high-quality Japanese alcoholic beverage sakes, such as Daiginjo-shu, which is produced from highly polished rice (less than a 50% polishing ratio), is synthesized from ethanol and caproic acid by sake yeast (Saccharomyces cerevisiae). In the yeast, caproic acid is synthesized by fatty acid synthase, the α6β6 composition consisting of 2 multifunctional subunits, α (Fas2) and β (Fas1).Citation1) A sake yeast mutant with high ethyl caproate-producing ability was isolated as a strain resistant to cerulenin, an inhibitor of fatty acid synthesis.Citation2) The cerulenin-resistance FAS2-G1250S mutation, in which the 1250th amino acid in Fas2 is changed from glycine to serine, is responsible for the high ethyl caproate-producing ability by causing an increase in the content of caproic acid.Citation2,3) Currently, in the brewing of high-quality sake, such as Daiginjo-shu, the cerulenin-resistant sake yeast strain K1801 (bred by the mating of the K9-segregant 9-Hp5 MATα with the cerulenin-resistant K1601-segregant 1601-Hp10 MATa by the Brewing Society of Japan) with high ethyl caproate-producing abilityCitation4) has been used widely.
The DNA integrity checkpoint (DIC) and spindle assembly checkpoint (SAC) in eukaryotes are essential for the maintenance of genome integrity in the event of perturbed DNA replication and spindle assembly abnormality, respectively.Citation5,6) Checkpoint integrity of yeast cells is evaluated by the sensitivity of yeast to drugs activating the checkpoint and by cell viability in the presence of the drug.Citation7–10) Benomyl activates the SAC by inhibiting microtubule polymerization,Citation7,8) whereas HU (hydroxyurea) activates the DIC by inhibiting DNA replication.Citation9,10) The genetic stability of sake yeast is thought to be important for the maintenance of both the fermentation properties of sake yeast and quality of the final product, sake. Therefore, the investigation of checkpoint integrity of yeast is an important step for breeding of risk-free industrial yeasts.
Recently, we investigated the checkpoint integrity of sake yeasts and found that K1801, but not the representative sake yeast strain K7,Citation11) exhibits sensitivity to benomyl, indicating that K1801 has a defect in its SAC.Citation12) In addition to the high producing ability of ethyl caproate, K1801 has excellent brewing properties such as low producing ability of total acids during sake brewing.Citation4) Therefore, the isolation of a spontaneous mutant from K1801, having both the excellent brewing properties of K1801 and normal checkpoint integrity, has been desired. In this study, as the first step for this breeding, we sought to identify the mutation causing the SAC defect in K1801.
Materials and methods
Strain and medium
The laboratorial and industrial budding yeast Saccharomyces cerevisiae strains were used in this study. The YPD medium was used in this study for general pre-culture and culture.
Drug sensitivity
After the pre-culturing of yeast cells in YPD medium at 28 °C for 12 h, the number of cells was adjusted to approximately 1.0 × 107 cells/mL (an optical density of 1.0 at 660 nm). The cell suspension was diluted serially and inoculated onto YPD plates, each containing one of various drugs: 0.5 μg/mL AbA (Aureobasidin A, TaKaRa No. 9000), 0.1 μg/mL NAT (Nourseothricin/clonNAT, Werner Bioagents No. 5.000 000), 2 μg/mL Cer (Cerulenin, WAKO No. 031-18183), 10–20 μg/mL Benomyl (SIGMA No. 1001328433), or 150 mM HU (Hydroxyurea, WAKO No. 089-06651). After incubation at 28 °C for 3–5 days, the cell growth (drug sensitivity) on the plate was observed.
Investigation of checkpoint function
Yeast cells were cultured in YPD medium at 28 °C for 12 h, and then the cells were inoculated freshly into YPD medium at 5.0 × 105 cells/mL. After incubation at 28 °C for 4 h, benomyl (final concentration 15 μg/mL for industrial strains or 30 μg/mL for laboratory ones) was added to the cell culture at early log phase (1.0–2.0 × 106 cells/mL). Then, during incubation at 28 °C for various times (0, 2, 4, and 6 h), the cell number was counted using a Sysmex F820 Hematology Analyzer, and the cells were then sampled and spread on a YPD plate. After incubation at 28 °C for 3 days, the number of developed colonies was counted for measurement of cell viability.
Analysis of morphological variation
Yeast cells at log phase (0.4–1.0 × 107 cells/mL) grown in YPD medium were fixed in a fixative solution (37% formaldehyde: 1 M potassium phosphate buffer, pH 6.5 = 1:1). The fixed cells were stained with fluorescein isothiocyanate–concanavalin A (FITC–ConA, Sigma, St. Louis, MO, USA), rhodamine–phalloidin (rh–ph, Invitrogen Corp, USA), and 4′,6′-diamidino-2-phenylindole (DAPI, Sigma) to visualize the cell wall, actin, and nucleus, respectively. Fluorescence microscopy images of the cells were acquired using an Axio Imager microscope equipped with a 6100 ECplan-Neofluar lens (Carl Zeiss, Germany), a CoolSNAP HQ cooled-CCD camera (Roper Scientific Photometrics, Tucson, AZ, USA), and AxioVision software (Carl Zeiss). Microscopy images of the cells were analyzed with CalMorph (ver. 1.3) image-processing software designed for diploids,Citation13) and we obtained the morphological data of the 501 traits from the single cell data. The CalMorph user manual is available at the Saccharomyces cerevisiae Morphological Database (SCMD, http://yeast.gi.k.u-tokyo.ac.jp/datamine/). Based on 5 independent experiments, morphological variation was investigated by calculating the phenotypic potential (PP) of the yeast strains as previously described.Citation13)
Sake brewing test
To investigate the fermentation properties of the sake yeasts, we performed a small-scale sake brewing test (total rice of 100 g, 20 g of rice with 60% polishing ratio as koji (Tokushima Seiko No.1–60) and 80 g of rice with 60% polishing ratio (Tokushima Seiko No.AA-60) added directly to the sake mash). The general properties and flavor components of the sake were measured by standard methods established by the National Tax Agency of Japan.
Results and discussion
Investigation of the effect of triploidy on SAC function
Before identification of the mutation, as the genome sequencing data indicated that K1801 was triploid (T. Akao, unpublished results), we investigated whether triploidy itself causes the SAC defect. To investigate this possibility, we constructed a laboratorial triploid strain by crossing BY4743 diploid (MATa/α his3∆1/his3∆1 leu2∆0/leu2∆0 LYS2/lys2∆0 met15∆0/MET15 ura3∆0/ura3∆0) with A13-18 haploid (MATa lys1), and examined its sensitivity to benomyl (10 μg/mL). No significant difference in drug sensitivity between the constructed triploid cells and the parental wild-type cells was observed, indicating that triploidy was not responsible for the SAC defect in K1801.
Investigation of sake yeast strain with the SAC defect as K1801
To investigate the mutation causing the defective SAC in K1801, we searched for sake yeast strains with the SAC defect as seen in K1801. For this purpose, we first examined the sensitivity of 22 other sake yeasts (the strains listed in Fig. (A)) to benomyl (10 μg/mL in YPD plates) and HU (150 mM in YPD plates). As a result (Fig. (A)), only K13 among the examined strains showed sensitivity to benomyl, as in the case of K1801 (data on other strains not shown). K13 was slightly more sensitive to benomyl than K1801 (Fig. (A)). We further examined cell viability in the presence of benomyl. Cells at early log phase were cultured in YPD liquid medium containing 15 μg/mL benomyl, and cell viability versus incubation time was then examined. As a result (Fig. (C)), after a 6-h incubation in the presence of benomyl, the viability of K13 dropped to 12% as K1801. These results indicated that K13 had a defect in its SAC function.
Fig. 1. SNP in the CDC55 gene in the sake yeast strains.
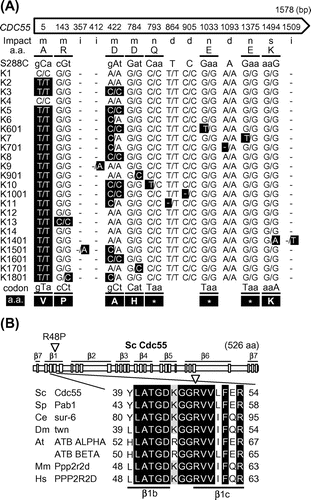
Investigation of a common SNP existing in only K13 and K1801
Next, we looked for single-nucleotide polymorphism (SNP) in 15 checkpoint-related genes (7 for SAC genes, BUB1, BUB3, MAD1, MAD2, MAD3, MPS1, and CDC55; and 8 for other checkpoint related-genes, MRC1, RAD9, RAD53, CHK1, TEL1, MEC1, SWE1, and CDC28) among the 23 sake yeasts (the strains listed in Fig. (A)) by comparative genomics using genome data of NRIB (personal communications from T. Akao, whole-genome data on the sake yeast strains will be reported elsewhere), and searched for a common SNP that existed in only K13 and K1801. As a result (Fig. (A)), we found 1 common SNP in the CDC55 gene (change of the 143rd nucleotide from G to C) in these 2 strains, leading to the change in the 48th amino acid of Cdc55 from arginine (R: CGT; the underlined nucleotide located in 2nd position of the codon) to proline (P: CCT, R48P). In addition to this SNP, the CDC55 gene in these 2 strains contained another SNP (the 5th nucleotide) that existed in a number of sake yeasts (Fig. (A)). The genome data indicated that K13 (diploid) had 2 copies of the R48P-type cdc55 gene (K13cdc55); on the other hand, K1801 (triploid) had 2 copies of the R48P-type cdc55 gene and 1 copy of R48-type cdc55 gene.
Cdc55, a regulatory B subunit of the protein phosphatase 2A (PP2A) complex, plays a role in a prolonged activation of the SAC by regulating MPF (maturation/M-phase promoting factor) activity and sister chromatid cohesion.Citation14) The regulatory B subunit with the WD40 domain structure forms a 7-bladed β propeller (β1-7), with each blade consisting of 4 β-strands (a–d; Fig. (B)).Citation15) The R48 residue is the evolutionally conserved amino acid and is located in the 3rd β-strand within the 1st blade (β1c) of Cdc55 (Fig. (B)). It should be noted that their parental strains (K9 and K1601 for K1801, and K9 and K10 for K13) do not have this R48P mutation (Fig. (A)), indicating that the mutation would have been generated during the breeding process, isolation of segregants or their mating. Although at the present time, K13 has not been used in sake brewing in Japan, it will be important to clarify why this mutation was generated/selected during the breeding of K13.
A Cdc55-R48P mutation is responsible for the SAC defect
To confirm that this Cdc55-R48P mutation was the cause of the SAC defect in sake yeasts K1801 and K13, we isolated the CDC55 gene from the genome of K7 (K7cdc55, R48-type) and K13 (K13cdc55, R48P-type) by PCR, cloned these amplified CDC55 gene on the integrative vector pAUR101, checked the cloned gene by DNA sequencing, and integrated them into the AUR1 locus on the genome of K1801 to construct 3 strains, K7cdc55-integrant (K1801-K7cdc55), K13cdc55-integrant (K1801-K13cdc55), and vector/pAUR101-integrant (K1801-V). The results of DNA sequencing and RT-PCR indicated that 1 copy of the cdc55 gene (K7cdc55 or K13cdc55) was integrated into the AUR1 locus on the genome of K1801 (data not shown). To investigate the checkpoint function of these integrants, we examined their sensitivity to benomyl and viability in the presence of the drug. As a result (Fig. (A)), the integration of K7cdc55 gene (K1801-K7cdc55), but neither K13cdc55 gene (K1801-K13cdc55) nor vector only (K1801-V), into the K1801 strain suppressed the sensitivity to benomyl (Fig. (A), 15 μg/mL). Furthermore, the viability of the K1801-K7cdc55 strain was maintained in the presence of benomyl (15 μg/mL); whereas the viabilities of both K1801-K13cdc55 and K1801-V strains dropped as in the case of K1801 (Fig. (C)).
K1801 having 2 copies of R48P-type cdc55 and 1 copy of R48-type cdc55 showed a defective SAC. One copy-integration of K7cdc55 (R48-type) suppressed the SAC-defect of K1801, indicating that 2 copies of R48-type cdc55 could suppress 2 copies of R48P-type cdc55. These results indicated that Cdc55-R48P is semidominant mutation in the SAC function.
To further confirm that the Cdc55-R48P mutation was responsible for the checkpoint defect, we examined the function of this mutation in the SAC in the CDC55-deleted laboratorial strain (X2180, diploid) background. For this purpose, we deleted 2 copies of the CDC55 gene (NAT marker) of X2180 (Fig. (B)) and confirmed the complete deletion by PCR. Then, we integrated the PCR-isolated K7cdc55, K13cdc55 or vector only into the AUR1 locus on the genome of CDC55-deleted X2180 strain (X2180∆cdc55). The result of RT-PCR indicated that 2 copies of K7cdc55 or 1 copy of K13cdc55 were integrated into the AUR1 locus on the genome of X2180∆cdc55 (data not shown). To investigate the SAC function of these integrants, we examined their sensitivity to benomyl. As a result (Fig. (B)), the CDC55-deleted strain (X2180∆cdc55) showed sensitivity to benomyl (20 μg/mL). As expected, integration of the K7cdc55 gene (X2180∆cdc55-K7cdc55), but that of neither the K13cdc55 gene (X2180∆cdc55-K13cdc55) nor vector (X2180∆cdc55-V), suppressed the benomyl sensitivity of the CDC55-deleted X2180 strain (X2180∆cdc55, Fig. (B)).
We further examined the viability of the integrants in the presence of benomyl (Fig. (D)). Consistent with the drug sensitivity, the viability of the X2180∆cdc55 cells dropped under this condition (30 μg/mL benomyl). On the other hand, the integration of K7cdc55 gene (X2180∆cdc55-K7cdc55), but that of neither the K13cdc55 gene (X2180∆cdc55-K13cdc55) nor vector (X2180∆cdc55-V), suppressed the decrease in the viability of the X2180∆cdc55 cells (Fig. (D)). These results indicated that the Cdc55-R48P mutation leads to a defective SAC and is a major cause of the SAC-defect in K1801.
Morphological analysis supports the conclusion
The image-processing program CalMorphCitation16) has been used to analyze high-dimensional morphological phenotyping of yeast strains.Citation16,17) One of the interesting morphological features, called the PP was developed by Levy and Siegal.Citation18) They used PP as the indicator of overall phenotypic variation in a cell population and showed a linkage between PP and genetic stability.Citation18) Earlier, we used PP for practical evaluation of morphological variation of sake yeast and showed that the SAC-defective K1801 strain was more heterogeneous in cell morphology (higher PP) than the sake yeast strains with normal checkpoint integrity.Citation12) Therefore in order to support the above conclusion, we analyzed the PP of several sake yeast strains after quantifying their phenotypic variance in terms of cell morphology, actin cytoskeleton, and nuclear DNA at single-cell resolution. We observed that checkpoint-defective K13 and K1801 strains showed more heterogeneous morphology in cell population than the representative sake yeast strain K7 (Fig. (A)), exhibiting a significantly high score in PP (p < 0.05 by Student’s t-test, Fig. (B)). Integration of the K7cdc55 gene (K1801-K7cdc55), but not that of the vector only (K1801-V), to the K1801 strain suppressed the increase in PP (Fig. (B)), implying that the Cdc55-R48P mutation was responsible for the high PP of these sake strains. We also examined the PP in the laboratorial CDC55-deleted strain (X2180∆cdc55) and found that deletion of CDC55 resulted in a high PP and that the integration of the K7cdc55 gene reduced the PP (Fig. (C)). Taken together, this analysis supported our contention that CDC55 was involved in overall phenotypic variation in the cell population and that the Cdc55-R48P mutation was the cause of the high PP in K13 and K1801.
Fig. 3. Morphological phenotypic variation of the yeast strains.
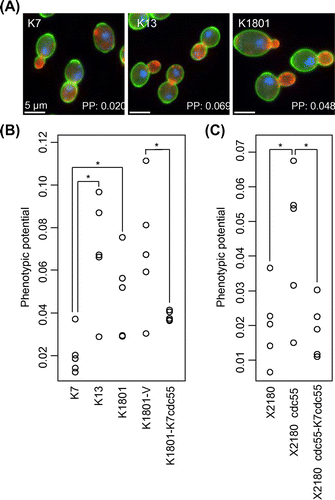
A Cdc55-R48P mutation is not related to the fermentation properties of K1801
To investigate whether the Cdc55-R48P mutation was related to the fermentation properties of K1801, we performed a small-scale (100 g of total rice) sake brewing test using the CDC55-integrants of K1801 (K1801-K7cdc55 and K1801-K13cdc55) and compared their fermentation properties with those of 3 sake yeasts, K7, K13, and K1801. In the CO2 production (Fig. (A)), no significant difference between the CDC55-integrants and other 3 sake yeasts was observed. Consistent with the results on CO2 production, the alcohol contents of sake brewed by the CDC55-integrants were similar to those of sake brewed by the parental K1801 strain (Fig. (B)). The acidity of sake brewed by K1801 was lower than that of sake brewed by the 2 other sake yeasts, K7 and K13 (Fig. (B)), indicating that K1801 has lower producing ability of total acids during sake brewing. The acidity of sake brewed by the CDC55-integrants was also lower than that of sake brewed by K7 and K13 (Fig. (B)). Furthermore, the high production of ethyl caproate of K1801 was not affected by the integration of K7cdc55 or K13cdc55 gene. These results established that the Cdc55-R48P mutation was not related to the major fermentation properties of K1801, such as CO2 production rate, low production of acids, and high production of ethyl caproate. However, the isoamyl acetate contents in sake brewed by the strains showing a defective SAC (K13, K1801, and K1801-K13cdc55) were slightly higher than that in sake by the other strains (K7 and K1801-K7cdc55). So far the relation between the isoamyl acetate production and the SAC function is unclear. Further analysis will be necessary to clarify this point.
Fig. 4. Sake brewing test using the indicated industrial yeast strains.
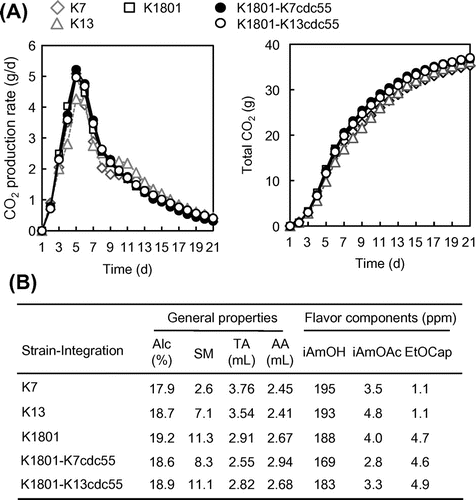
In conclusion, to identify the mutation causing the SAC defect of K1801, we first searched for sake yeast(s) with a SAC-defect like that of K1801 among 23 sake yeast strains and found that only K13 had such a defect. Then, we searched for a common SNP in only K1801 and K13 by examining SNP in 15 checkpoint-related genes in 23 sake yeasts using the NRIB genome data (T. Akao, unpublished results). As a result, we found 1 mutation, R48P of Cdc55, which is the PP2A regulatory B subunit that plays an important role in the SAC. Furthermore, we confirmed that the Cdc55-R48P mutation was responsible for the defective SAC in K1801 by examining the effect of this mutation on the SAC in the CDC55-deleted laboratorial strain background. Morphological analysis confirmed that this mutation caused a high morphological variation in the cell population. We also showed that this mutation did not affect the excellent brewing properties. Taken together, these results indicated that this mutation is a target for breeding of a new risk-free K1801 strain with normal checkpoint integrity. In the future, the isolation of a spontaneous reversion mutant from K1801 will be important for breeding of such sake yeast having both the excellent fermentation properties of K1801 and normal checkpoint integrity.
Authors contribution
Experimental design and interpretation of data were conducted by all authors. T.G., R.N., K.K., H.O., and H.S. carried out experiments. T.G., R.N., K.K., H.T., H.H., M.I., N.O., T.A., H.S., Y.O., and D.H. planned the project. T.G. and D.H. wrote the paper with input from the co-authors, and the other authors reviewed the manuscript.
Disclosure statement
No potential conflict of interest was reported by the authors.
Funding
This work was supported by Ministry of Education, Culture, Sports, Science and Technology, Japan [grant number 15H04402] to Y.O.
Acknowledgments
We thank Y. Zhou and T. Tako (National Research Institute of Brewing), S. Morinaga (University of Tokyo) for their support of the experiments, as well as the Brewing Society of Japan and Niigata Sake Brewers Association for helpful discussion, advice, and communication. We also thank all members of Asahi Sake Brewing Co. Ltd. for their support.
References
- Stoops JK, Wakil SJ. The yeast fatty acid synthetase. J. Biol. Chem. 1981;256:8364–8370.
- Ichikawa E, Hosokawa N, Hata Y, et al. Breeding of sake yeast with improved ethyl caproate productivity. Agric. Biol. Chem. 1991;55:2153–2154.
- Aritomo K, Hirosawa I, Hoshida H, et al. Self-cloning yeast strain containing novel FAS2 mutations produce a higher amount of ethyl caproate in Japanese sake. Biosci. Biotechnol. Biochem. 2004;68:206–214.10.1271/bbb.68.206
- Yoshida K. Kyokai sake yeast 1801. Nippon Jozo Kyokaishi (in Japanese). 2006;101: 910–922.
- Boddy MN, Russell P. DNA replication checkpoint. Curr. Biol. 2001;11:R953–R956.10.1016/S0960-9822(01)00572-3
- Musacchio A, Salmon ED. The spindle-assembly checkpoint in space and time. Nat. Rev. Mol. Cell Biol. 2007;8:379–393.10.1038/nrm2163
- Hoyt MA, Totis L, Roberts BT. S. cerevisiae genes required for cell cycle arrest in response to loss of microtubule function. Cell. 1991;66:507–517.10.1016/0092-8674(81)90014-3
- Li R, Murray AW. Feedback control of mitosis in budding yeast. Cell. 1991;66:519–531.10.1016/0092-8674(81)90015-5
- Weinert TA, Kiser GL, Hartwell LH. Mitotic checkpoint genes in budding yeast and the dependence of mitosis on DNA replication and repair. Genes Dve. 1994;8:652–665.10.1101/gad.8.6.652
- Allen JB, Zhou Z, Siede W, et al. The SAD1/RAD53 protein kinase controls multiple checkpoints and DNA damage-induced transcription in yeast. Genes Dve. 1994;8:2401–2415.10.1101/gad.8.20.2401
- Akao T, Yashiro I, Hosoyama A, et al. Whole-genome sequencing of sake yeast Saccharomyces cerevisiae Kyokai no. 7. DNA Res. 2011;18:423–434.10.1093/dnares/dsr029
- Tamura H, Okada H, Kume K, et al. Isolation of a spontaneous cerulenin-resistant sake yeast with both high ethyl caproate-producing ability and normal checkpoint integrity. Biosci. Biotechnol. Biochem. 2015;79:1191–1199.10.1080/09168451.2015.1020756
- Yvert G, Ohnuki S, Nogami S, et al. Single-cell phenomics reveals intra-species variation of phenotypic noise in yeast. BMC Syst. Biol. 2013;7:54.10.1186/1752-0509-7-54
- Minshull J, Straight A, Rudner AD, et al. Protein phosphatase 2A regulates MPF activity and sister chromatid cohesion in budding yeast. Curr. Biol. 1996;6:1609–1620.10.1016/S0960-9822(02)70784-7
- Xu Y, Chen Y, Zhang P, et al. Structure of a protein phosphatase 2A holoenzyme: insights into B55-mediated Tau dephosphorylation. Mol. Cell. 2008;31:873–885.10.1016/j.molcel.2008.08.006
- Ohtani M, Saka A, Sano F, et al. Development of image processing program for yeast cell morphology. J. Bioinform. Comput. Biol. 2004;01:695–709.10.1142/S0219720004000363
- Ohya Y, Sese J, Yukawa M, et al. High-dimensional and large-scale phenotyping of yeast mutants. Proc. Natl. Acad. Sci. USA. 2005;102:19015–19020.10.1073/pnas.0509436102
- Levy SF, Siegal ML. Network hubs buffer environmental variation in Saccharomyces cerevisiae. PLoS Biol. 2008;6:2588–2604.