Abstract
Two kinds of oligosaccharides, N-acetylraffinosamine (RafNAc) and N-acetylplanteosamine (PlaNAc), were synthesized from N-acetylsucrosamine and melibiose using the transgalactosylation activity of Aspergillus niger α-galactosidase. RafNAc and PlaNAc are novel trisaccharides in which d-glucopyranose residues in raffinose (Raf) and planteose are replaced with N-acetyl-d-glucosamine. These trisaccharides were more stable in acidic solution than Raf. RafNAc was hydrolyzed more rapidly than Raf by α-galactosidase of green coffee bean. In contrast, RafNAc was not hydrolyzed by Saccharomyces cerevisiae invertase, although Raf was hydrolyzed well by this enzyme. These results indicate that the physicochemical properties and steric structure of RafNAc differ considerably from those of Raf.
Graphical Abstract
Two kinds of novel trisaccharides were synthesized from N-acetylsucrosamine and melibiose, using transgalactosylation action of Aspergillus niger α-galactosidase.
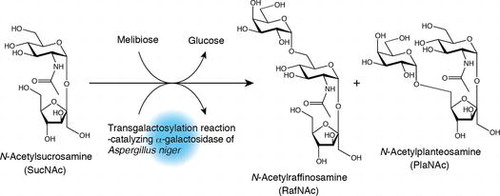
Introduction
Several oligosaccharides obtained by the hydrolysis of chitin, a β-(1,4)-linked polymer of N-acetyl-d-glucosamine (2-acetamido-2-deoxy-d-glucopyranose, GlcNAc), exhibit preventative effects against several diseases. For example, the disaccharide (di-N-acetylchitobiose) and the trisaccharide (tri-N-acetylchitotriose) are promising candidates for preventing celiac disease, which is caused by gliadin-derived peptides.Citation1) The hexasaccharide (hexa-N-acetylchitohexaose) exhibits antitumorCitation2–4) and antimicrobial activityCitation5,6) in mice by enhancing immunological defenses. Moreover, several heterodisaccharides consisting of d-galactopyranose (Gal) and GlcNAc have shown physiologically beneficial functions. For example, lacto-N-biose [β-d-galactopyranosyl-(1→3)-2-acetamido-2-deoxy-d-glucopyranose] is a candidate bifidus factor,Citation7) and lacto-N-biose and N-acetyllactosamine [β-d-galactopyranosyl-(1→4)-2-acetamido-2-deoxy-d-glucopyranose] inhibit the attachment of a range of pathogens to alveolar epithelial cells.Citation8,9)
Based on these findings, we hypothesized that other GlcNAc-containing oligosaccharides may also exhibit physiologically beneficial effects. We, therefore, attempted to produce new types of functional GlcNAc-containing oligosaccharides. Several years ago, we produced N-acetylsucrosamine [β-d-fructofuranosyl-(2↔1)-2-acetamido-2-deoxy-α-d-glucopyranoside, SucNAc] from sucrose (Suc) and GlcNAc using dried Aspergillus oryzae mycelia containing the transfructosylation-catalyzing enzyme β-fructofuranosidase (EC.2.4.1.99) as a whole-cell catalyst (Fig. (A)).Citation10) SucNAc is a disaccharide in which d-glucopyranose (Glc) residue in Suc is replaced with GlcNAc. We also developed a methodology for large-scale production of SucNAc, using a column reactor packed with dried A. oryzae mycelia.Citation11) More recently, we produced N-acetyl-1-kestosamine [β-d-fructofuranosyl-(2→1)-β-d-fructofuranosyl-(2↔1)-2-acetamido-2-deoxy-α-d-glucopyranoside, 1-KesNAc] and N-acetylnystosamine [β-d-fructofuranosyl-(2→1)-β-d-fructofuranosyl-(2→1)-β-d-fructofuranosyl-(2↔1)-2-acetamido-2-deoxy-α-d-glucopyranoside, NysNAc] from SucNAc, using above-dried A. oryzae mycelia (Fig. (B)).Citation12) 1-KesNAc and NysNAc are novel trisaccharides in which Glc residues in 1-kestose and nystose, which are being used as functional oligosaccharide, are replaced with GlcNAc. We are currently evaluating the efficacy of SucNAc, 1-KesNAc, and NysNAc as prebiotics. We are also enzymatically synthesizing additional novel GlcNAc-containing oligosaccharides.
Fig. 1. Schematic representation of the synthesis of several GlcNAc-containing oligosaccharides using transglycosylation-catalyzing glycosidases.
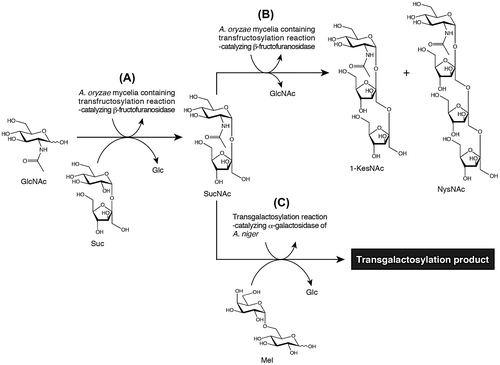
In the present report, we describe the synthesis of oligosaccharides from SucNAc and melibiose (Mel) via transgalactosylation catalyzed by the α-galactosidase [EC 3.2.1.22] (Fig. (C)). We aim at utilizing the oligosaccharide that is provided by this study as functional oligosaccharide. To perform this task, it is desirable to use cheaper enzyme preparation. In this study, we used commercially available industrial grade α-galactosidase preparation that was made from culture broth of Aspergillus niger, because it was provided at lower cost.
Materials and methods
Materials
SucNAc was prepared according to previously reported proceduresCitation11) GlcNAc, Gal, Glc, d-fructofuranose (Fru), Suc, Mel, and raffinose (Raf) were purchased from Wako Pure Chemical Industries (Osaka, Japan). p-Nitrophenyl α-d-galactopyranoside (pNP α-Gal) was obtained from Sigma-Aldrich (St Louis, MO, USA) All other chemicals were of analytical- grade Charcoal (particle size, 63–300 μm) for column chromatography was purchased from Wako Pure Chemical Industries Industrial-grade α-galactosidase preparation (Sumizyme AGS), which is produced using A niger, was purchased from Shin Nihon Chemical (Aichi, Japan) Highly purified green coffee bean α-galactosidase and Saccharomyces cerevisiae invertase (β-fructofuranosidase) were purchased from Sigma-Aldrich.
Analysis of carbohydrates
Qualitative and quantitative analyses of carbohydrates were carried out using thin-layer chromatography (TLC) and high-performance liquid chromatography (HPLC), respectively. TLC plates (silica gel 60, 0.25 mm; Merck KGaA, Darmstadt, Germany) were developed twice using 1-butanol/pyridine/H2O 8:3:1 (v/v/v) or four times using acetonitrile/H2O 5:1 (v/v) as the mobile phase, and resolved compounds were visualized by spraying the plates with an aqueous solution containing 2.4% H3(PMo12O40)•nH2O, 5% H2SO4, and 1.5% H3PO4, followed by heating. HPLC was carried out using an LC-10AS pump (Shimadzu, Kyoto, Japan) equipped with a Shodex RI-101 differential refractometer (Showa Denko, Tokyo, Japan) and a COSMOSIL Sugar-D column (Nacalai Tesque, Kyoto, Japan; size, 4.6 × 250 mm). The system was operated under isocratic conditions using acetonitrile/H2O 77:23 (v/v) as the mobile phase (flow rate, 0.8 mL min−1). The amounts of various oligosaccharides in the reaction mixture were estimated by comparing the area of each oligosaccharide peak with the peak areas of standard samples. Transgalactosylation products were characterized using 1H and 13C nuclear magnetic resonance (NMR) spectroscopy as well as electrospray ionization–mass spectrometry (ESI-MS). The 1H (600MHz) and 13C (150 MHz) NMR spectra were recorded in D2O at 25°C using a Varian NMR system 600 spectrometer (Varian, Palo Alto, CA, USA). 2D 1H-1H DQF-COSY spectra, 1H-1H TOCSY spectra, 1H-13C HSQC spectra, 1H-13C HSQC-TOCSY spectra, and 1H-13C HMBC spectra were acquired for the complete 1H and 13C assignments. The 1H and 13C chemical shifts were relative to sodium 2,2-dimethyl-2-silapentane-5-sulfonate-d6 as an external standard. ESI-MS measurements were carried out using a JMS-T100 time-of-flight mass spectrometer (JEOL, Tokyo, Japan). Optical rotation was measured in H2O at 23°C using a P-1030 polarimeter (JASCO, Tokyo, Japan).
Assay of enzyme activity
Hydrolytic activity of α-galactosidase from A. niger and green coffee bean was assayed using pNP α-Gal as a substrate and was monitored by measuring the amount of p-nitrophenol released from this glycoside by the enzymatic reaction. After 500 μL of 20 mM sodium phosphate buffer (pH 7.0) containing the enzyme and pNP α-Gal (1 mM) was incubated at 30°C, the enzymatic reaction was stopped by adding 500 μL of aqueous 0.3 M Na2CO3. The amount of p-nitrophenol liberated in the reaction mixture was determined by measuring the absorbance at 405 nm. One unit (U) of enzyme activity was defined as the amount of enzyme required to liberate 1 μmol of p-nitrophenol/min under the assay conditions used.
Hydrolytic activity of S. cerevisiae invertase was assayed using Suc as a substrate and was monitored by measuring the amount of reducing sugar released from Suc. The enzyme solution (50 μL) was added to 50 μL of 0.4% Suc in 20 mM sodium citrate buffer (pH 5.0) and the mixture was incubated at 30°C. The amount of reducing sugar in the reaction mixture was determined by the Somogyi–Nelson method using Glc as a standard.Citation13) One U of Suc-hydrolyzing activity of invertase was defined as the amount of enzyme required to hydrolyze 1 μmol of Suc/min under the assay conditions used.
Production and purification of oligosaccharides
Sumizyme AGS (400 mg) was dissolved in 2 mL of 20 mM sodium citrate buffer (pH 5.5), and the resulting solution was dialyzed against the same buffer to remove low-molecular weight impurities. The α-galactosidase activity in the enzyme solution was 720 U/mL. A 6-mL reaction mixture containing 5.04 g of SucNAc (13.15 mM), 1.5 g of Mel (4.382 mM), and 1,100 U of α-galactosidase in 20 mM sodium citrate buffer (pH 5.5) was stirred with a magnetic stirrer at 40°C. To monitor the progress of the reaction, 10-μL aliquots were withdrawn from the reaction mixture at designated time points and heated at 95°C for 5 min using a hot dry bath, and then the compounds in each collected reaction mixture were analyzed by TLC.
To purify the reaction products, the enzymatic reaction was performed for 4 h under the conditions described above, after which the reaction mixture was heated for 5 min in boiling water and then loaded onto a charcoal column (size, 5.5 × 60 cm; solvent, H2O). The monosaccharides contained in the reaction mixture were eluted with water, and oligosaccharides adhering to the charcoal were eluted with 2-propanol/H2O 1:9 (v/v). Fractions containing oligosaccharides were collected and concentrated by evaporation, and target oligosaccharides were further purified by chromatography using a UNIVERSAL Amino 5L column [Yamazen, Osaka, Japan; size,
6.4 × 24 cm; solvent, 2-propanol/H2O 7:3 (v/v); flow rate, 2 mL min−1]. After fractions containing target oligosaccharides were collected and concentrated by evaporation, the samples were dissolved in a small amount of H2O and lyophilized. After the lyophilized material was dissolved in 10 mL of acetic anhydride/pyridine (1:1, v/v), the mixture was stirred overnight with a magnetic stirrer and then poured into 100 mL of water. The peracetylated oligosaccharides generated in this reaction was extracted using ethyl acetate (100 mL), and the organic layer was washed with water (3 × 100 mL), 1N HCl solution (100 mL), saturated aqueous NaHCO3, and brine, and finally, the solvent was removed by evaporation after drying over anhydrous Na2SO4. The peracetylated oligosaccharides were purified by silica gel chromatography using two concatenated ULTRA PACK SI-40D columns [Yamazen; size of one column,
5 × 30 cm; solvent, dichloromethane/ethyl acetate/methanol 25:25:1 (v/v); flow rate, 7 mL min−1]. Fractions containing the purified compounds were combined, and the solvent was removed by evaporation. For deacetylation, the resulting residue was dissolved in 40 mL of triethylamine/H2O/methanol (1:2:7, v/v) and stirred at room temperature for 24 h. After removal of solvent from the reaction mixture by evaporation, the resulting residue was dissolved in 20 mL of ethanol, and this solution was concentrated by evaporation (this operation was repeated three times). After the deacetylated sample was sufficiently dried in vacuo, it was dissolved in a small amount of H2O and lyophilized, resulting in a white powder as the final product.
Characterization of oligosaccharides
Oligosaccharide stability was evaluated at several temperatures using the following buffers: 20 mM glycine–HCl (pH 3.0), 20 mM sodium citrate (pH 5.0), 20 mM sodium phosphate (pH 7.0), and 20 mM glycine–NaOH (pH 9.0). Buffer solutions (100 μL) containing each oligosaccharide (1%, w/v) were incubated at various temperatures (25, 50, 75, and 100°C) for 1 h, and then the compounds in the solutions were analyzed by TLC.
Hydrolysis of the oligosaccharides was investigated using two kinds of exo-type glycosidases, green coffee bean α-galactosidase or S. cerevisiae invertase. α-Galactosidase activity was evaluated at 30°C in 500 μL of 20 mM sodium phosphate buffer (pH 7.0) containing 5 mg of each oligosaccharide and 0.74 U of enzyme. Invertase activity was evaluated at 30°C in 500 μL of 20 mM sodium citrate buffer (pH 5.0) containing 5 mg of each oligosaccharide and 0.2 U of enzyme. For both enzymes, reaction progress was monitored by withdrawal of a 100-μL aliquot of reaction mixture at designated time points. The enzymatic reaction was stopped by heating at 95°C for 5 min using a hot dry bath, and the compounds in each reaction mixture were then analyzed by TLC and HPLC.
Results
Production, purification, and identification of oligosaccharides
Oligosaccharides were synthesized in 6 mL of 20 mM sodium phosphate buffer containing 1,100 U of A. niger α-galactosidase, 5.04 g of SucNAc, and 1.5 g of Mel (3:1 M ratio of SucNAc and Mel), and the reaction products were confirmed by TLC. A spot thought to be a transgalactosylation product (indicated by an arrow in Fig. (A)) became larger with the disappearance of the Mel spot. Although we conducted the transgalactosylation reaction at various concentrations of SucNAc and Mel, above reaction condition was most suitable to obtain the product (data not shown). The product obtained by the above reaction was purified from the reaction mixture using two types of column chromatography until it became a single spot on the TLC plate. HPLC analysis of the purified sample indicated that two products (compounds 1 and 2) were contained in the sample (Fig. (B)). The ratio of compound 1 (first peak in Fig. (B)) to compound 2 (second peak in Fig. (B)) was approximately 4:1. Attempts to purify these compounds using normal or reversed-phase column chromatography were unsuccessful. Consequently, these compounds were isolated as peracetylated derivatives using silica gel column chromatography. TLC analysis of the acetylated compounds revealed two neighboring spots (data not shown), indicating that the compounds were separable by silica gel column chromatography. After separation by column chromatography, the compounds were deacetylated and lyophilized, yielding white powder as the final product.
Fig. 2. Compounds generated by the transgalactosylation activity of A. niger α-galactosidase.
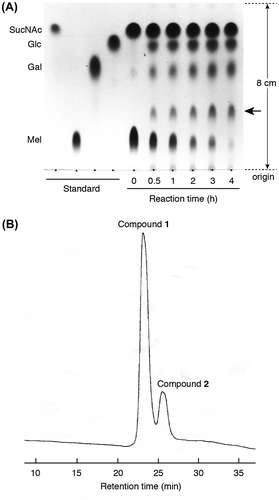
The structures of compounds 1 and 2 were determined using MS and NMR spectroscopy. Two ion peaks, corresponding to [M + H]+ and [M + Na]+, were observed at m/z 546 and m/z 568 (Fig. S1), respectively, suggests that the compounds are trisaccharides consisting of Fru, Gal, and GlcNAc. The assignments of compounds 1 and 2 from 1H- and 13C-NMR spectra (Figs. S2 and S3) are shown in Figs. (A) and (B) and Table S1. The positions of the glycosidic bonds in these compounds were determined using HMBC NMR experiments. The GlcNAc residue anomeric hydrogen atom (GlcNAc H1) coupling constants (J1,2) for compounds 1 and 2 were 3.8 and 3.6 Hz (Figs. (A) and (B)), respectively, indicating that the GlcNAc residue in each compound is connected by an α-glycosidic bond. The GlcNAc H1 of each compound exhibited a cross peak with the second carbon atom of the Fru residue (Fru C2) (Fig. S4). These results indicate that the structure of both compounds contains a SucNAc residue. The Gal residue anomeric hydrogen atom (Gal H1) coupling constants (J1,2) for compounds 1 and 2 were 3.9 and 3.7 Hz (Figs. (A) and (B)), respectively, indicating that the Gal and SucNAc residues are linked by an α-glycosidic bond. The Gal H1 of compound 1 exhibited a cross-peak with the sixth carbon atom of the GlcNAc residue (GlcNAc C6) (Fig. S4A). In contrast, the Gal H1 of compound 2 exhibited a cross-peak with the sixth carbon atom of the Fru residue (Fru C6) (Fig. S4B). The MS and NMR results described above enabled us to identify compounds 1 and 2 as β-d-fructofuranosyl α-d-galactopyranosyl-(1→6)-2-acetamido-2-deoxy-α-d-glucopyranoside (Fig. (A)) and α-d-galactopyranosyl-(1→6)-β-d-fructofuranosyl-(2↔1)-2-acetamido-2-deoxy-α-d-glucopyranoside (Fig. (B)), respectively. We designated the former oligosaccharide N-acetylraffinosamine (RafNAc) and the latter oligosaccharide N-acetylplanteosamine (PlaNAc). RafNAc and PlaNAc are novel trisaccharides in which Glc residues in Raf and planteose (Pla), which are being used as functional oligosaccharide, were replaced with GlcNAc.
Fig. 3. Structures and NMR data of compounds 1 (A) and 2 (B).
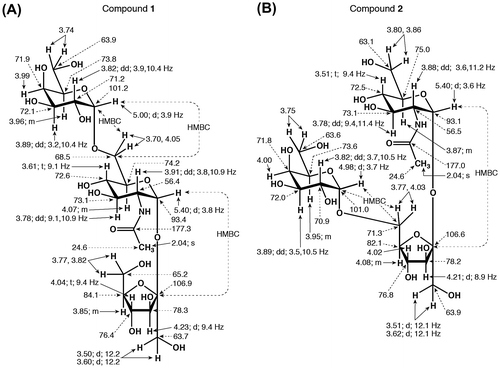
After 4 h of stirring, 231 mg of RafNAc (molar yield = 9.66% from Mel) and 42.0 mg of PlaNAc (molar yield = 1.76% from Mel) were obtained from the respective reaction mixtures. No other carbohydrate peaks were detected in HPLC analyses of obtained oligosaccharide samples.
Property of oligosaccharides
The specific rotation ([α]D23) values for RafNAc and PlaNAc obtained using constant weight samples were 91.8° (c = 0.486, H2O) and 118.6° (c = 0.490, H2O), respectively. As crystals of these trisaccharides were not obtained, their melting points were not determined.
The pH and thermal stability of RafNAc and PlaNAc were also investigated, and the results were compared with those for Raf. As Pla was not available, we could not use this trisaccharide for comparison. Raf, RafNAc, and PlaNAc did not decompose upon incubation at 100°C for 1 h in buffer solutions at pH 5.0, 7.0, or 9.0 (data not shown). Although Raf was partially cleaved to Mel and Fru by incubation at 75 or 100°C for 1 h in buffer solution at pH 3.0 (Fig. (A)), negligible decomposition of RafNAc and PlaNAc were observed under the same conditions (Figs. (B) and (C)), indicating that both GlcNAc-containing trisaccharides are more stable in acidic solution than Raf.
Fig. 4. TLC analyses demonstrating decomposition of Raf (A), RafNAc (B), and PlaNAc (C) in 20 mM glycine-HCl buffer (pH 3.0) at various temperatures. TLC plates were developed four times using acetonitrile/H2O 5:1 (v/v) as the mobile phase.
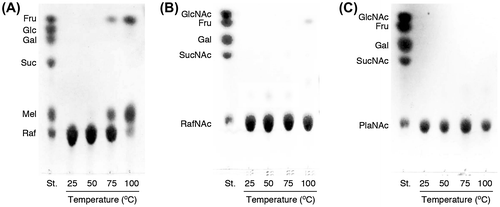
Enzymatic hydrolysis of RafNAc and PlaNAc by two kinds of exo-type glycosidases (green coffee bean α-galactosidase and S. cerevisiae invertase) was investigated, using Raf for comparison. All three trisaccharides were cleaved to Gal and Suc by the α-galactosidase (Fig. S5A), and the degradation rates were in the order RafNAc > Raf > PlaNAc (Fig. (A)). In contrast, the invertase did not hydrolyze RafNAc, but did cleave Raf to Mel and Fru (Fig. (B) and Fig. S5B).
Discussion
In the present study, we synthesized two novel oligosaccharides (RafNAc and PlaNAc) consisting of Gal, Fru, and GlcNAc from SucNAc and Mel via transgalactosylation catalyzed by A. niger α-galactosidase. However, the yields of these oligosaccharides were low. The highest yields of RafNAc and PlaNAc were obtained using a reaction mixture containing 85% (w/v) SucNAc and 25% (w/v) Mel (3:1 M ratio of SucNAc and Mel). However, even under these conditions, a considerable quantity of Gal (released from Mel by α-galactosidase-catalyzed hydrolysis) appeared (Fig. (A)). It is clear that the lower yield of the transgalactosylation products was associated with the hydrolytic reaction catalyzed by α-galactosidase. Usually, as water contents in the reaction mixture increase, a hydrolytic reaction by α-galactosidase becomes easy to progress. Therefore, to make transgalactosylation reaction progress effectively, it is necessary to perform the reaction under low water content or high substrate concentration. However, in our reaction system, the concentrations of the substrates SucNAc and Mel could not be increased further because they were almost at saturation. Fortunately, glycosidases that exhibit high transglycosylation activity in reaction mixtures with a relatively high water content are known. To produce RafNAc or PlaNAc at higher yield in the future, we will seek an α-galactosidase that catalyzes transgalactosylation reactions more efficiently in aqueous reaction mixtures from various micro-organisms. In above reaction, RafNAc was produced more than PlaNAc, indicating that Gal is easy to transfer to the primary hydroxyl group on GlcNAc C6 of SucNAc than that on Fru C6 of SucNAc. We think that such regioselective reaction is caused by the substrate specificity of A. niger α-galactosidase.
Although Raf was hydrolyzed to Mel and Fru under acidic and high-temperature conditions (Fig. (A)), negligible hydrolysis of RafNAc and PlaNAc were observed under the same conditions (Figs. (B) and (C)), indicating that these GlcNAc-containing trisaccharides are more stable under acidic conditions than Raf. Previously, we confirmed that SucNAc is more resistant than Suc to hydrolysis in acidic solution.Citation10) Our findings indicate that the acetamide group of GlcNAc stabilizes the glycosidic bond between Fru and GlcNAc. Green coffee bean α-galactosidase showed hydrolytic activity against RafNAc and PlaNAc as well as Raf, which is the common substrate for assays of α-galactosidase activity, and the activity against RafNAc was higher than that against Raf. In contrast, the activity of the enzyme against PlaNAc was very low (Fig. (A)). These results indicate that RafNAc is more suitable than Raf as a substrate for green coffee bean α-galactosidase and that PlaNAc is not readily recognized by this enzyme. Although Raf was hydrolyzed to Mel and Fru by S. cerevisiae invertase, this enzyme did not hydrolyze RafNAc (Fig. (B)). Previously, we confirmed that S. cerevisiae invertase does not hydrolyze SucNAc.Citation11,14) These results suggest that substrate recognition by S. cerevisiae invertase is hindered by the acetamido group of the GlcNAc residue in each oligosaccharide.
Although we were unable to synthesize RafNAc and PlaNAc at high yield in the present study, in future studies we will evaluate the effectiveness of RafNAc and PlaNAc as prebiotics using samples obtained in this work.
Funding
This study was supported by the grant [grant number 26450166] for Creative Scientific Research (C) from the Ministry of Education, Science, Sports, and Culture (Japan) from 2009 to 2011. This study was also supported by a grant [grant number 15A-001] from the College of Bioresearch Sciences of Nihon University.
Author contributions
Y. Sakaki and M. Katou performed the enzymatic synthesis of oligosaccharides and the purification of them. M. Tashiro, C. Sakuma, and W. Hakamata were in charge of instrumental analysis of the oligosaccharides. T. Hirano investigated the properties of the oligosaccharides. T. Nishio designed and supervised the experiments. For writing manuscripts, T. Nishio and M. Tashiro contributed by 85 and 15%, respectively.
Disclosure statement
No potential conflict of interest was reported by the authors.
TBBB_1189316_Supplemental_data.docx
Download MS Word (5.2 MB)Notes
Abbreviations: Gal, d-galactopyranose; Glc, d-glucopyranose; Fru, d-fructofuranose; GlcNAc, N-acetyl-d-glucosamine; Suc, sucrose; Mel, melibiose; SucNAc, N-acetylsucrosamine; Raf; raffinose; Pla, planteose; 1-KesNAc, N-acetyl-1-kestosamine; NysNAc, N-acetylnystosamine; RafNAc, N-acetylraffinosamine; PlaNAc, N-acetylplanteosamine; DQF-COSY, double-quantum filtered-correlation spectroscopy; TOCSY, total correlation spectroscopy; HSQC heteronuclear single-quantum correlation; HSQC-TOCSY, heteronuclear single-quantum correlation-total correlation spectroscopy; and HMBC, heteronuclear multiple bond correlation.
References
- Vincentini O, De Angelis I, Iannuccelli R, et al. Protective effects of mannan in Caco-2/TC7 cells treated with wheat-derived peptides. Carbohydr. Polym. 2005;62:338–343.10.1016/j.carbpol.2005.08.008
- Suzuki K, Mikami T, Okawa Y, et al. Antitumor effect of hexa-N-acetylchitohexaose and chitohexaose. Carbohydr. Res. 1986;151:403–408.10.1016/S0008-6215(00)90359-8
- Tokoro A, Takewaki N, Suzuki K, et al. Growth-inhibitory effect of hexa-N-acetylchitohexaose and chitohexaose against Meth-A solid tumor. Chem. Pharm. Bull. 1988;36:784–790.10.1248/cpb.36.784
- Tsukada K, Matsumoto T, Aizawa K, et al. Antimetastatic and growth-inhibitory effects of N-acetylchitohexaose in mice bearing Lewis lung carcinoma. Jap. J. Cancer Res. 1990;81:259–265.10.1111/cas.1990.81.issue-3
- Tokoro A, Kobayashi M, Tatewaki N, et al. Protective effect of N-acetyl chitohexaose on Listeria monocytogenes infection in mice. Microbiol. Immunol. 1989;33:357–367.10.1111/mim.1989.33.issue-4
- Kobayashi M, Watanabe T, Suzuki S, et al. Effect of N-acetylchitohexaose against Candida albicans infection of tumor-bearing mice. Microbiol. Immunol. 1990;34:413–426.10.1111/mim.1990.34.issue-5
- Nishimoto M, Kitaoka M. Practical preparation of lacto-N-biose I, a candidate for the bifidus factor in human milk. Biosci. Biotechnol. Biochem. 2007;71:2101–2104.10.1271/bbb.70320
- Thomas R, Brooks T. Common oligosaccharide moieties inhibit the adherence of typical and atypical respiratory pathogens. J. Med. Microbiol. 2004;53:833–840.10.1099/jmm.0.45643-0
- Thomas R, Brooks T. Attachment of Yersinia pestis to human respiratory cell lines is inhibited by certain oligosaccharides. J. Med. Microbiol. 2006;55:309–315.10.1099/jmm.0.46102-0
- Hirano T, Wada T, Iwai S, et al. Synthesis of β-d-fructofuranosyl-(2↔1)-2-acetamide-2-deoxy -α-d-glucopyranoside (N-acetylsucrosamine) using β-fructofuranosidase-containing Aspergillus oryzae mycelia as a whole-cell catalyst. Carbohydr. Res. 2012;353:27–32.10.1016/j.carres.2012.03.037
- Sato H, Yokochi S, Kasama T, et al. Continuous production of β-d-fructofuranosyl-(2↔1)-2-acetamide-2-deoxy-α-d-glucopyranoside (N-acetylsucrosamine) using a column reactor packed with β-fructofuranosidase-containing mycelia of Aspergillus oryzae immobilized on a porous carrier. J. Appl. Glycosci. 2012;59:153–160.10.5458/jag.jag.JAG-2012_003
- Nishio T, Juami M, Wada T, et al. Enzymatic synthesis of novel oligosaccharides from N-acetylsucrosamine using β-fructofuranosidase from Aspergillus oryzae. Carbohydr. Res. 2013;382:108–112.10.1016/j.carres.2013.10.006
- Somogi M. Notes of sugar determination. J. Biol. Chem. 1952;195:19–23.
- Sakuma C, Furihata K, Nishio T, et al. Analysis of weak affinity of β-d-fructofuranosyl-(2,1)-2-acetamide-2-deoxy-α-d-glucopyranoside for yeast β-fructofuranosidase using NMR spectroscopy. J. Carbohydr. Chem. 2014;33:498–505.10.1080/07328303.2014.964407