Abstract
Among three MAPK disruptants of Neurospora crassa, Δmak-1 was sensitive and Δmak-2 was hypersensitive to micafungin, a beta-1,3-glucan synthase inhibitor, than the wild-type or Δos-2 strains. We identified six micafungin-inducible genes that are involved in cell wall integrity (CWI) and found that MAK-1 regulated the transcription of non-anchored cell wall protein gene, ncw-1, and the beta-1,3-endoglucanase gene, bgt-2, whereas MAK-2 controlled the expression of the glycosylhydrolase-like protein gene, gh76-5, and the C4-dicarboxylate transporter gene, tdt-1. Western blotting analysis revealed that, in the wild-type strain, MAK-1 was constitutively phosphorylated from conidial germination to hyphal development. In contrast, the phosphorylation of MAK-2 was growth phase-dependent, and micafungin induced the phosphorylation of unphosphorylated MAK-2. It should be noted that the phosphorylation of MAK-1 was virtually abolished in the Δmak-2 strain, but was significantly induced by micafungin, suggesting functional cross talk between MAK-1 and MAK-2 signalling pathway in CWI.
Graphical abstract
Both MAP kinases, MAK-1 and MAK-2, are activated by micafungin, and upregulate the expression of cell wall-related genes.

Filamentous fungi possess a structurally dynamic cell wall that protects the cell from changes in nutrient conditions, osmotic pressure, cell wall damage, and other environmental stresses.Citation1) The fungal cell wall has been shown to be primarily composed of glucans, chitin, glycoproteins, and mannans,Citation2–5) and the unique structure of the cell wall, which is different from those of bacteria or plants, has been considered to be an ideal target for the development of fungicides. Filamentous fungi, including Neurospora crassa, undergo multicellular developmental phases, e.g. conidial germination, hyphal fusion, and sexual morphogenesis, such as ascospore formation. Thus, fungal cells continuously undergo biogenesis and remodeling of the cell wall polysaccharide network; these processes are regulated by cell wall integrity (CWI) signalling pathway(s) in response to cell wall damage, heat shock, and hyperosmolality in the budding yeast.Citation6)
Mitogen-activated protein (MAP) kinase pathways, consisting MAP kinase (MAPK), MAPK kinase (MAPKK), and MAPKK kinase (MAPKKK), play an important role in the differentiation, cell division, and stress response in eukaryotic organisms.Citation1,7) The Saccharomyces cerevisiae Mpk1 MAPK cascade has been shown to be involved in a CWI pathway.Citation6,8,9) Cell surface sensors, such as Wsc1/Wsc2/Wsc3, Mid2, and Cwh43, receive stress signals and transmit information regarding the cell wall status to a Rho family GTP-binding protein, Rho1. The Mpk1 MAPK cascade is then activated by a signal from Rho1 via protein kinase C (PKC), and in turn leads to activation of transcription factors, such as Rlm1 and the Swi4/Swi6 complex. This pathway controls the transcription level of cell wall biogenesis enzyme-encoding genes, such as FKS1 and FKS2 that encode components of the beta-1,3-glucan synthases, CHS3 that encodes a chitin synthase, BGL2 that encodes an endo-beta-1,3-glucanase, and DFG5 that encodes a putative GPI-anchored mannosidase.Citation8,10)
Mpk1-like MAPK pathways have been shown to play crucial roles in pathogenesis in filamentous fungi. In the rice blast fungus Magnaporthe oryzae, Mpk1-like MAPK MPS1 is essential for pathogen penetration.Citation11,12) In Aspergillus fumigatus, a deletion mutant of mpkA, a homolog of S. cerevisiae Mpk1-encoding gene, showed severe sensitivity to cell wall active compounds and marked alterations in morphology.Citation13) A Mpk1-like MAPK pathway controls not only sexual development, but also accumulation of deoxynivalenol toxin in plant pathogen Fusarium graminearum.Citation14) Aspergillus nidulans MpkA MAPK is involved in germination of conidial spores and polarized growth, and transcription of alpha-1,3-glucan synthase genes (agsA, agsB) is dependent on MpkA and its downstream transcription factor RlmA.Citation15,16)
N. crassa possesses three MAPK cascades, namely OS-2, MAK-1, and MAK-2 pathways.Citation17–19) Each component of these MAPK cascades in N. crassa shows high homology to those of S. cerevisiae: The OS-4(MAPKKK)−OS-5(MAPKK)−OS-2(MAPK) pathway is homologous to the Ssk2/22−Pbs2−Hog1 osmosensing (OS) pathway; NRC-1−MEK-2−MAK-2 is homologous to the Ste11−Ste7−Fus3/Kss1 mating/filamentation pathway, and MIK-1−MEK-1−MAK-1 is homologous to the Bck1−Mkk1/2−Mpk1 CWI pathway. The N. crassa OS-2 MAPK pathway plays an important role in the osmotic stress response.Citation20,21)
All of the N. crassa OS-2 MAPK pathway mutants show high osmotic sensitivities and resistance to certain fungicides, such as dicarboximides and phenylpyrroles. The OS pathway is also involved in the output pathway(s) of the circadian clock by regulating various genes that function in relation to the osmotic stress response.Citation22–26)
The MAK-2 MAPK pathway is comprised of NRC-1 MAPKKK, MEK-2 MAPKK, and MAK-2 MAPK. A mak-2 mutant exhibited complete female sterility, and its ascospores could not germinate; therefore, the MAK-2 pathway appears to be essential for mating.Citation27,28) In addition, MAK-2 plays an important role in cell–cell communication between genetically identical cells.Citation29) It has recently been shown that the MAK-2 MAPK pathway participates in phosphate signalling.Citation30) The MAK-1 MAPK pathway, composed of MIK-1 MAPKKK, MEK-1 MAPKK, and MAK-1 MAPK, is known to participate in secondary metabolism by regulating the expression of a tyrosinase gene, and plays a role as an output pathway of the circadian clock.Citation31,32) It is genetically assumed that the MAK-1 pathway contributes to CWI. Cell wall stress is sensed at the cell surface by certain stress sensors such as WSC-1. These sensors induce GDP-to-GTP exchange at Rho GTPases, RHO-1 and RHO-2. The activated RHO protein transfers the signals to a PKC, which in turn activates the MAK-1 pathway.Citation33,34) However, involvement of the MAK-1 MAPK in CWI is not fully understood.
Few investigations have focused on the involvement of MAPKs in CWI; in particular, on the mechanism underlying transcriptional regulation of cell wall-related genes in N. crassa.Citation1) We have previously reported that N. crassa possesses five putative beta-(1,3)-glucanosyltransferases, and that the expression of gel-4 was induced by micafungin, a beta-1,3-glucan synthase inhibitor, independently of mak-1 and mak-2.Citation26) In this study, to examine whether any of MAPKs are involved in its CWI, we investigated the phenotypes of N. crassa MAPK gene disruptants, namely Δmak-1, Δmak-2, and Δos-2. Among these, Δmak-1 was sensitive, and Δmak-2 was far more sensitive to micafungin than the wild-type strain. Western blotting analysis revealed the increased phosphorylation of MAK-1 and MAK-2 by micafungin treatment. Furthermore, using microarray-based analysis and quantitative RT-PCR, we identified six micafungin-inducible genes that could be involved in cell wall degradation. Among these, the expression of ncw-1, encoding a non-anchored cell wall protein, and bgt-2, encoding a GPI-anchored endo-glucanase, was regulated by MAK-1 MAPK. MAK-2 MAPK was shown to control the expression of a putative mannosidase gene, gh76-5, and a C4-dicarboxylate transport protein gene, tdt-1. These results suggest that MAK-1 and MAK-2 MAPK pathways are involved in the regulation of CWI in response to cell wall damage.
Materials and methods
Fungal strains, growth media, and sensitivity test
The N. crassa strains used in this study are listed in Table . N. crassa C1-T10-37A and C1-T10-19a are wild-type strains that are closely related to the standard Oak Ridge wild-type strain.Citation35) The knockout strains from the functional genomics program were obtained from the Fungal Genetics Stock Center (FGSC) (Kansas City, MO).Citation36) The strains were grown on agar-solidified Vogel’s medium N (VN) supplemented with 1.2% (w/v) sucrose at 28 °C.Citation37)
Table 1. The Neurospora crassa strains used in this study.
In order to assess the sensitivity of strains to cell wall stress, we used micafungin, a beta-1,3-glucan synthase inhibitor (Astellas Pharma, Tokyo, Japan), and sodium dodecyl sulfate (SDS; Wako Pure Chemical, Osaka, Japan). A series of 10-fold dilutions, derived from a starting conidial suspension (5 × 107 conidia), of each strain were spotted on VN media plates supplemented with 0.2% (w/v) sucrose and 1% (w/v) sorbose, and were then incubated for 48 h at 28 °C.
Western blotting analysis
Conidia (3.3 × 106/mL) were germinated in liquid VN medium in Sakaguchi flask for 4, 8, 12, 16, 18 h at 25 °C. For micafungin treatment, cultured mycelia (16 h) were transferred to VN medium containing micafungin (1 μg/ml) for 2 h. After cultured cells were harvested by filtration and then disrupted by bead beating, total cell protein was extracted using protein extraction buffer (50 mM HEPES buffer, pH7.6, 10% glycerol, 137 mM NaCl, 25 mM NaF, 10 mM Na4P2O7, 2 mM Na3VO4, complete protease inhibitor cocktail, Roche Diagnostics, Tokyo, Japan). About 50 μg of cell extracts was denatured with Laemmli sample buffer and separated using 10% SDS-polyacrylamide gel electrophoresis. Proteins on gel were blotted onto nitrocellulose membranes using Tris-glycine buffer (25 mM Tris-HCl, 192 mM glycine) with 20% methanol. After being blocked in 1xTBST (25 mM Tris-HCl, pH 7.4, 137 mM NaCl, 2.68 mM KCl, and 0.2% Tween 20) with 1% bovine serum albumin for 2 h at room temperature, membrane was incubated with either anti-ERK1/2 (Sigma Aldrich, St. Louis, MO, M5670) or phospho-p44/42 MAPK antibody (Cell Signaling Technology, Danvers, MA, #9101) in the blocking solution overnight, with gentle shaking, at 4 °C. The anti-ERK1/2 and phospho-p44/42 MAPK antibodies were used for detecting total MAK-2 and both phosphorylated MAK-1 and MAK-2, respectively. After incubation with primary antibody, membrane was washed three times with 1xTBST and then incubated with alkaline phosphatase (AP)-conjugated anti-rabbit immunoglobulin G (Promega, Fitchburg, WI, S3731) in 1xTBST for 2 h at room temperature. The membrane was subsequently washed three times with 1xTBST and then washed twice with alkaline buffer (100 mM Tris-HCl, pH9.5, 100 mM NaCl, 50 mM MgCl2). To detect signals, membrane was incubated in the alkaline buffer including 0.34 mg/ml NBT (nitro-blue tetrazolium chloride) and 0.18 mg/ml BCIP (5-bromo-4-chloro-3′-indolylphosphatase p-toluidine salt), followed by rinsing in reagent quality water to stop AP enzyme reaction.
Gene expression analysis by quantitative real-time reverse transcription PCR
Total RNA was isolated as previously described by Noguchi et al.Citation22). The conidia (3.3 × 106/mL) were germinated for 16 h at 25 °C and then transferred to VN medium containing micafungin (1 μg/mL) for 2 h. Total RNA was isolated from each sample using a FastRNA Pro Red kit (MP Biomedicals, Tokyo, Japan). Each RNA sample (1 μg of total RNA) was subjected to cDNA synthesis and quantitative reverse transcription PCR (qRT-PCR) using the LightCycler system (Roche Diagnostics, Tokyo, Japan), as described by Yamashita et al.Citation38). mRNA levels of target genes were quantified using Universal ProbeLibrary (Roche Diagnostics, Tokyo, Japan). Primers and probes in this study were summarized in Table S1. The relative values of gene expression were calculated by comparing threshold cycle (Ct) between Ctcontrol and Ctmicafungin in each strain. Actin was used as reference gene in the qPCR analysis. To indicate the effect of micafungin treatment on gene expression, ΔΔCt were calculated by the following calculation formula: ΔΔCt: {Ct(control)−Ct(actin at control)}−{Ct(micafungin)−Ct(actin at micafungin treated sample)}. Three independent replicated experiments (cell culture, RNA extraction, reverse transcription, and qPCR) were performed.
Microarray-based analysis of micafungin-inducible genes
In microarray analysis, conidia (3.3 × 106/mL) were cultured in liquid VN medium for 16 h at 25 °C and then treated with micafungin (1 μg/mL) for 2 h. Total RNA was isolated from each sample using a FastRNA Pro Red kit (MP Biomedicals, Tokyo, Japan). mRNA was isolated from each sample using the Dynabeads mRNA Direct Kit (Invitrogen/Thermo Fisher Scientific Inc., Waltham, MA, USA) according to the manufacturer’s protocol.
Microarray experiments were carried out as previously described by Takahashi et al.Citation39). Each mRNA sample was subjected to cDNA synthesis, and labeling of cDNA and hybridization were performed using an Array 50 Expression Array Detection kit (Genisphere, Hatfield, PA) according to the manufacturer’s protocol. Neurospora 70-mer oligonucleotide microarray slides, which were developed by the Neurospora research community, were obtained from FGSC. Detailed information about these slides can be found online at the Filamentous Fungal Microarray Database (http://www.yale.edu/townsend/Links/ffdatabase/downloads.html). Microarrays were scanned at two wavelengths to detect Cy3 and Cy5 signals using a Dual-Laser DNA Microarray Scanner (Agilent Technologies, Santa Clara, CA, G2565AA), and the data were extracted with Feature Extraction Software version 9.5 (Agilent Technologies, Santa Clara, CA). For microarray hybridization analysis, triplicate independent experiments were performed.
Results
Sensitivity of individual MAPK gene-knockout strains to cell wall stress
Nine MAPK pathway gene-knockout mutants, namely Δmik-1, Δmek-1, and Δmak-1 (MAK-1 MAPK pathway), Δnrc-1, Δmek-2, and Δmak-2 (MAK-2 MAPK pathway), Δos-4, Δos-5, and Δos-2 (OS-2 MAPK pathway), which were isolated by genomic knockout using a high-throughput gene replacement protocol,Citation36) were characterized phenotypically. Of three MAPK disruptants (Δmak-1, Δmak-2, and Δos-2), Δmak-1 and Δmak-2 showed abnormal colony morphologies (Fig. (A)). As previously reported, Δmak-1 showed a rosette-like colony growth,Citation19,31,40) whereas Δmak-2 exhibited conidiation that started from the colony center.Citation27,28) These colony phenotypes of Δmak-1 or Δmak-2 were conserved within each MAPK pathway (data not shown), which was also consistent with previous findings.Citation19,27,28,31,40) The relative radial growth rates of these disruptants were reduced to less than 40% of that of the wild-type strain grown on agar-solidified VN medium for 24 h at 28 °C (Fig. (B)).
Fig. 1. Comparison of the growth and morphology of mak-1, mak-2, and os-2 disruptants of Neurospora crassa.
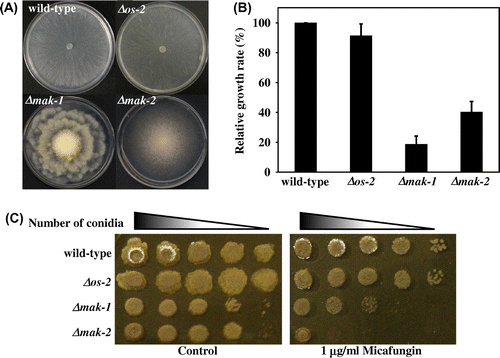
To explore the contribution of the three MAPK pathways to CWI, we examined the effect of cell wall-damaging compounds on Δmak-1, Δmak-2, and Δos-2. Both Δmak-1 and Δmak-2 were sensitive to micafungin, a beta-1,3-glucan synthase inhibitor, than the wild-type, and Δmak-2 was even more sensitive than Δmak-1 (Fig. (C)). Sensitivities of upstream gene disruptants of MAK-1 and MAK-2 pathways to micafungin were highly similar to that of Δmak-1 and Δmak-2, respectively (Fig. S2). Both deletion mutants, Δmak-1and Δmak-2, exhibited hypersensitivity to SDS as well, than wild-type strain (Fig. S1). The sensitivity level of Δmak-2 to SDS was also higher than Δmak-1. Δos-2 did not show hypersensitivities to micafungin and SDS in this study (data not shown).
Phosphorylation levels of MAK-1 and MAK-2 and response to cell wall stress
S. cerevisiae Mpk1 MAPK can be phosphorylated by cell wall-damaging compounds and leads to activation of the CWI signalling pathway by controlling the expression of cell wall biosynthetic enzymes at a transcriptional level.Citation6,10) We tested the basal phosphorylation levels of MAK-1 and MAK-2 in the N. crassa wild-type strain from the conidial germination stage to hyphal development in liquid VN medium by means of a Western blotting assay using an anti-phospho p44/42 MAPK antibody. As shown in Fig. (A), both MAK-1 and MAK-2 were phosphorylated in germinating conidia, but MAK-2 was dephosphorylated in growing hyphae; however, phosphorylation of MAK-2 was significantly induced upon micafungin treatment. Although the total amount MAK-1 could not be estimated, some level of MAK-1 phosphorylation was observed throughout these growth stages. These data suggest that MAK-1 was constitutively activated during vegetative growth. MAK-2 was intrinsically phosphorylated only during the early growth stages and was dephosphorylated in hyphae; however, it was activated when the cell wall was damaged.
Fig. 2. Phosphorylation of MAK-1 and MAK-2 MAPKs is induced by micafungin.
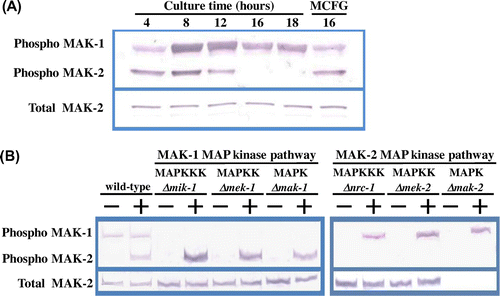
The phosphorylation levels of MAK-1 and MAK-2 were estimated in all of the MAK-1 and MAK-2 pathway gene deletion mutants (Fig. (B)). It is noteworthy that the basal phosphorylation of MAK-1 was virtually abolished in all MAK-2 MAPK pathway mutants. However, when mycelia of the MAK-2 MAPK pathway mutants were treated with micafungin, phosphorylation of MAK-1 was significantly induced in the Δnrc-1, Δmek-2, and Δmak-2 strains (Fig. (B)). Furthermore, MAK-2 proteins in the MAK-1 pathway mutants as well as the wild-type strain were phosphorylated in the presence of micafungin.
Expression level of cell wall biogenesis-related genes
We examined the transcriptional level of 12 cell wall biogenesis-related genes in the wild-type, Δmak-1, and Δmak-2 strains using real-time RT-PCR. These genes were chosen because their homeologues in A. nidulans are known to be involved in the cell wall chitin or glucan synthesis (Table S2). We confirmed that PCR efficacies, determined for each gene using specific primers and TaqMan probe sets, were similar (data not shown); therefore, cycle threshold (Ct) values, defined as the number of cycles required for the fluorescent signal to cross a threshold (i.e. the lower the Ct level the greater the amount of target mRNA in the sample), were used for direct comparison of the basal expression of each gene. The basal transcription levels of each cell wall biogenesis-related genes in the untreated mycelia of three tested strains were substantially the same (Table ). Most of these genes were considered to be highly expressed (Ct values <27), suggesting that their encoded protein products are continuously required for cell wall biosynthesis in N. crassa. The effect of micafungin on the expression of these 12 cell wall biogenesis-related genes was not marked, yet the transcriptional levels of these genes in the wild-type mycelia were somewhat increased after the treatment, except for those of ags-2, gel-2, and chs-1, along with those of the MAPK genes, mak-1 and mak-2. In the wild-type, micafungin upregulated ags-1, fks-1, gfa-1, chs-2, and chs-3 two- to threefold, and gel-1, chs-4, chs-5, and chs-7 less than twofold (Table ). In Δmak-1, this induction was minimal (e.g. ags-1, fks-1, gfa-1, chs-2, chs-4, chs-5, and chs-7) or absent in most cases. These results suggested that both MAK-1 and MAK-2 may control cell wall glucan or chitin synthesis-related genes under certain stress conditions, but do not primarily participate in regulation of these genes.
Table 2. Expression analysis of 12 cell wall biogenesis-related genes by quantitative real-time RT-PCR.
Microarray-based analyses
We carried out a genome-wide search for genes that are involved in the CWI in response to micafungin treatment, by measuring the transcriptional level of all genes in growing hyphae of N. crassa using microarray-based analysis. To minimize artifacts arising from low expression values in the microarray data, only genes with raw intensity values of >600 counts for micafungin-treated mRNA were chosen, and genes with absolute values of the natural logarithm of the ratios (treated vs. untreated) of >0.4 were considered as candidates for micafungin-inducible genes.
Twenty-three genes were selected based on the criteria, and these candidates included chs-2 and gel-4 (Table ). The expression level of chs-2 was increased by micafungin, as described above (Table ). Moreover, we had already reported that gel-4 was significantly upregulated by micafungin treatment in the wild-type, Δmak-1, and Δmak-2 strains (Fig. ).Citation26) Among these twenty-one candidates, besides chs-2 and gel-4, the expression levels of 7 selected genes (dga-1, ncw-3, tdt-1, phiA, gh76-5, bgt-2, and ncw-1), which may be involved in CWI, were quantitated by real-time RT-PCR in the wild-type, Δmak-1, and Δmak-2 strains. We found that six genes, besides dga-1, were induced at least twofold by micafungin treatment in the wild-type mycelia. Micafungin significantly upregulated phiA, encoding cell wall protein PhiA, and ncw-3, encoding non-anchored cell wall protein-3, at more than 20-fold in the wild-type; however, this upregulation was not completely abolished in Δmak-1 or Δmak-2 strains (Fig. ), indicating that MAK-1 and MAK-2 may not be primarily involved in the upregulation of these genes. However, the expression levels of these genes were lessened considerably in Δmak-2, and even more so in Δmak-1.
Table 3. Microarray analysis of genes upregulated by micafungin treatment in wild-type strain.
Fig. 3. Relative mRNA levels of selected genes in response to micafungin.
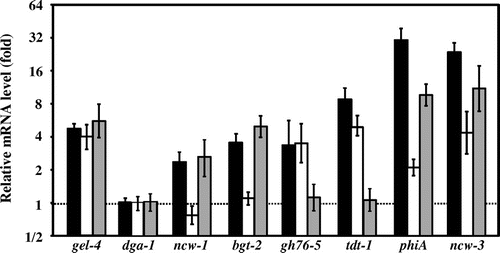
Expression of ncw-1, encoding non-anchored cell wall protein-1, and bgt-2, encoding predicted GPI-anchored glycoside hydrolase, were also induced by micafungin, and the induction was almost completely abolished in the Δmak-1 but not in the Δmak-2 strain, indicating that the induction is MAK-1-dependent. On the other hand, upregulation of gh76-5, encoding alpha-1,6-mannanase, and tdt-1, encoding a putative C4-dicarboxylate transporter/malic acid transport protein, by this chemical were almost completely abolished in Δmak-2, but not in Δmak-1, indicating that the induction of these genes is MAK-2-dependent. These results suggested that, when the cell wall is damaged, MAK-1 induces the expression of ncw-1 and bgt-2, and that MAK-2 induces gh76-5 and tdt-1, most likely for maintaining CWI.
Discussion
MAPK cascades are highly conserved and are well-characterized signalling pathways in eukaryotes.Citation41,42) In this study, we attempted to evaluate the contributions of three MAP kinases, MAK-1, MAK-2, and OS-2, to CWI in N. crassa. We found that both MAK-1 and MAK-2 are activated by cell wall stress, and that MAK-1, MAK-2, and OS-2, participate in regulation of genes encoding cell wall-related proteins in different ways in N. crassa.
Three-tiered MAPK modules, comprised of nine orthologues to equivalent MAPK modules of S. cerevisiae, have been identified in N. crassa.Citation7,17–19) Three N. crassa MAPKs, namely MAK-1, MAK-2, and OS-2 are homologous to Mpk1, Fus3/Kss1, and Hog1 in S. cerevisiae, respectively. Although S. cerevisiae Mpk1 MAPK is known to control CWI by regulating a number of cell wall-related genes, such as FKS1, FKS2, CHS3, BGL2, and DFG5,Citation6,8) N. crassa MAK-1 has been reported to contribute to the morphogenesis, sexual development, secondary metabolism, and circadian clock.Citation31,32,40) On the other hand, N. crassa MAK-2 MAPK has been shown to be involved in hyphal fusion, sexual development, and phosphate signalling.Citation27–30)
We herein showed that basal transcription levels of several glycopolymer synthase genes, such as ags-1, ags-2, fks-1, gfa-1, chs-2, chs-3, chs-4, chs-5, and chs-7, which should be important for cell wall biogenesis and remodeling, were high in the growing mycelia of the wild-type strain, and the loss of mak-1 or mak-2 hardly influenced the expression of these genes (Table ). These results may suggest that N. crassa MAK-1 and MAK-2 are not primarily involved in the regulation of these cell wall biogenesis-related genes. However, it should be noted that micafungin treatment slightly upregulated most of the glycopolymer synthase genes tested and, in some cases, the upregulation was diminished in Δmak-1 or Δmak-2 strains (Table ). N. crassa MAK-1 and MAK-2 kinases may not be involved in the basic expression of these glycopolymer synthase genes, but may regulate some of them in response to cell wall damage. For example, the alpha-1,3-glucan synthase gene ags-1 and the beta-1,3-glucan synthase gene fks-1 were slightly upregulated by micafungin, probably in a MAK-1-dependent manner (Table ). In A. nidulans, micafungin increases the expression of agsB (alpha-1,3-glucan synthase) and fksA (beta-1,3-glucan synthase). MpkA of A. nidulans, a Mpk1-like MAPK, regulates the transcription of agsB, but not of fksA.Citation16) It has been reported that alpha-1,3-glucan, one of the major cell wall polysaccharides, is not responsible for fungal viability, but seems to protect against cell wall stresses in A. nidulans.Citation43)
We showed that a deletion mutant of mak-1 was slightly sensitive to micafungin. MAK-1 may be continuously involved in cell wall construction and remodeling, since some level of MAK-1 phosphorylation was observed in wild-type strain during spore germination, and the total level of phosphorylated MAK-1 seemed to be constant from conidial germination to hyphal development in the absence of micafungin (Fig. (A)). It is known that Δmak-1 shows hypersensitivity to cell wall-damaging agents, Calcofluor white and Congo red.Citation44,45) On the other hand, we found that Δmak-2 strain was significantly more sensitive to micafungin and SDS than the wild-type, Δos-2, and Δmak-1 (Fig. (C), Fig. S1). MAK-2 may act to combat cell wall perturbation. MAK-2 was significantly phosphorylated in the wild-type strain only during the early stages of growth under normal cultivation conditions (Fig. (A)). N. crassa forms conidial anastomosis tubes (CATs), which are produced in any type of conidia and conidial germ tubes.Citation46) Previous reports have revealed that MAK-2 plays an important role in the cell–cell communication between CAT tips.Citation29,47,48) In addition, MAK-1 and MAK-2 are phosphorylated in the wild-type strain during germ tubes/CATs fusion; however, both phosphorylations are altered in mutants lacking HAM-6 and HAM-8, which are predicted membrane proteins, and also GPI-anchored cell wall protein, HAM-7.Citation49,50) Considering the functional importance of MAK-2 in cell fusion between CAT tips, the phosphorylation of MAK-2 in the early growth stages suggested that MAK-2 probably controls the expression of a set of enzymes and/or proteins that functions during the conidial germination or cell–cell fusion.
Maddi et al. have revealed that MAK-1 regulates two distinct cellular activities, maintenance of CWI and hyphal anastomosis, and that these activities are controlled by two major sensor proteins,Citation44) WSC-1 and HAM-7, respectively, in N. crassa. In this study, absence of MAK-2 did not prevent phosphorylation of MAK-1, because a fraction of mycelial MAK-1 was phosphorylated upon exposure to micafungin (Fig. (B)). Park et al. reported that the phosphorylation level of MAK-1 in N. crassa is increased by Aculeacin A, another beta-(1,3)-glucan synthase inhibitor.Citation31) Taken together, these findings suggest that MAK-1 is activated by dual signals, nutrient signalling, and cell wall stress, and probably some of the nutrient signals are transmitted through the MAK-2 pathway. The phosphorylation patterns of MAK-1 and MAK-2 in the deletion mutants of mik-1, mek-1, nrc-1, or mek-2 gene were very similar to those in the Δmak-1 or Δmak-2 strains (Fig. (B)). These results suggest that a cross talk occurs between the MAK-1 and MAK-2 pathways in response to cell wall perturbation. There have been several studies that may suggest cross talks between MAK-1 and MAK-2 pathways in N. crassa. Functional links have been reported between the nuclear Dbf2p-related kinase COT1 and MAK-1 and MAK-2 MAPK signallings in the regulation of filamentous growth, hyphal fusion, and sexual development.Citation19,47,48) In addition, it has been reported that two components of the STRIPAK complex, HAM-2 and HAM-3, regulate the nuclear accumulation of MAK-1 in a MAK-2-dependent manner.Citation51) Recently, the activities of MAK-1 and MAK-2 have been shown to be under control of the circadian clock.Citation32)
In this study, we identified six micafungin-inducible genes: ncw-3 (NCU07817), tdt-1 (NCU07517), phiA (NCU00399), gh76-5 (NCU09937), bgt-2 (NCU09175), and ncw-1 (NCU05137). Comparing the effect of micafungin on the expression of these genes in the wild-type, Δmak-1, and Δmak-2 strains, the expression patterns of genes could be categorized into three groups: (i) MAK-1- or MAK-2-independent, (ii) MAK-1-dependent, and (iii) MAK-2-dependent. Both phiA (NCU00399) and ncw-3 (NCU07817) were significantly upregulated by micafungin in MAK-1 or MAK-2 independent manner. Our previous work indicated that gel-4, which encodes a beta-1,3-glucanosyltransferase, was increased by micafungin treatment independently of MAK-1 or MAK-2 (Fig. , Kamei, Yamashita, et al., 2013). These genes belong to the first category.
On the other hand, ncw-1 (NCU05137) and bgt-2 (NCU09175) belong to the second category. Their expression levels increased, possibly in response to impaired cell wall synthesis, and this induction was virtually abolished only in the Δmak-1 strain, suggesting that MAK-1 controls their upregulation. The former gene encodes a non-anchored cell wall protein and the latter a GPI-anchored endo-glucanase. NCW-1 and BGT-2 have been shown to be localized in the cell wall.Citation52) Integral cell wall glycoproteins, including cell wall cross-linking enzymes needed for cell wall biogenesis, remodeling, and reinforcement, are cross-linked into the cell wall matrix in filamentous fungi.Citation2,4,53) S. cerevisiae endo-beta-1,3-glucanase Bgl2 is a major cell wall protein that is involved in cell wall maintenance,Citation54) and expression of the corresponding gene is regulated by the CWI pathway.Citation6) Although N. crassa BGT-2 is not homologous to S. cerevisiae Bgl2, it is interesting that an endo-beta-1,3-glucanase gene was also regulated by the Mpk1-like MAPK in N. crassa. A recent study showed that BGT-2 is localized at the site involving cell wall remodeling such as at the leading edge of developmental septa, hyphal fusion sites, and conidial poles.Citation55)
In contrast, the induction of both gh76-5 (NCU09937) and tdt-1 (NCU07517) were virtually abolished in the Δmak-2 but not the Δmak-1 strain, suggesting that MAK-2 controls the upregulation of gh76-5 and tdt-1. GH76-5 belongs to the glycoside hydrolase family 76, which is a family of alpha-1,6-mannanases. Two other alpha-1,6-mannanases belong to this family, which are encoded by dfg-5 and dcw-1 and are required for the efficient cross-linking of cell wall proteins into the cell wall in N. crassa,Citation44) but their expression was not induced by micafungin (result not shown). Their homologues in S. cerevisiae are GPI-anchored membrane proteins and are required for cell growth and cell wall biogenesis.Citation56) DFG5 is one of the genes regulated by Mpk1 in S. cerevisiae.Citation8,10) The transcription of N. crassa gh76-5 was regulated by MAK-2, but not MAK-1, in N. crassa. Additionally, tdt-1 encodes a putative C4-dicarboxylate transporter/malic acid transport protein, but its function in N. crassa remains unclear. A C4-dicarboxylate transporter/malic acid transport protein, Mae1, functions in the uptake of malate and other dicarboxylates by means of a proton symport in Schizosaccharomyces pombe.Citation57,58) Recently, in addition to the metabolic roles, MAK-2 was found to participate in the Pi-signalling pathway and may affect metabolic modulation.Citation30) Our finding that MAK-2 controls tdt-1 expression seems to be consistent with this finding.
The importance of alpha-1,3-glucan in relation to growth and virulence has also been studied in several pathogenic fungi.Citation59,60) The OS pathway in N. crassa consists of a His-Asp phosphorelay system mediated by the OS-1 osmosensor-like histidine kinase and MAP kinase cascade (OS-4−OS-5−OS-2).Citation20,21,61) It is known that OS-2 MAP kinase is phosphorylated by osmotic stress and fludioxonil, and that this controls various stress response-related genes, including those involved in glycerol synthesis (gcy-1, gcy-3, and dak-1), encoding catalases (ctt-1 and cat-1), as well as clock-controlled genes (ccg-1 and bli-3).Citation22,24,25,62) Most of the OS-2-dependent genes are regulated by the transcription activator ATF-1/ASL-1.Citation38,63) In this study, the Δos-2 strains did not show sensitivity to micafungin (Fig. (C)), and OS-2 MAP kinase was not activated by micafungin treatment (result not shown). However, fludioxonil, a fungicide, had been shown to induce gel-1, which encodes a beta-1,3-glucanosyltransferase that is possibly involved in cell wall biogenesis, and this induction was shown to be mediated by OS-2 and ATF-1/ASL-1.Citation26) These results suggested that the OS-2 pathway could participate in the regulation of cell wall remodeling or reinforcement in an adaptation to osmotic stress. Although further studies are necessary to clarify the function of above-mentioned proteins which may be involved in CWI, our findings indicate that not only MAK-1 but also MAK-2 are activated by cell wall stress, and that all MAP kinases, MAK-1, MAK-2, and OS-2, participate in regulation of genes encoding cell wall-related proteins in different ways in N. crassa.
Author contributions
MK and MF designed the research plan with assistance from AI and FF. MK, KY, and MT performed experiments. MK, FF, and MF analyzed data and wrote the manuscript. All authors read and approved the manuscript.
Disclosure statement
No potential conflict of interest was reported by the authors.
Funding
This work was supported by a Japan Society for the Promotion of Science (JSPS) KAKENHI [grant number 258011].
Supplemental material
The supplemental material for this paper is available at http://dx.doi.org/10.1080/09168451.2016.1189321.
TBBB_1189321_Table_S2.doc
Download MS Word (34.5 KB)TBBB_1189321_Table_S1.doc
Download MS Word (32.5 KB)TBBB_1189321_Fig_S1.pptx
Download MS Power Point (3.7 MB)Acknowledgments
We thank Dr Shinpei Banno (Japan Plant Protection Association) for useful discussions and suggestions in the course of our research. We are grateful to the Fungal Genetics Stock Center and the Neurospora Functional Genomics Program Project for microarray slides and strains.
References
- Borkovich KA, Ebbole DJ. Cellular and molecular biology of filamentous fungi. Washington, DC: ASM Press; 2010.10.1128/9781555816636
- Klis FM. Cell wall assembly in yeast. Yeast. 1994;10:851–869.10.1002/(ISSN)1097-0061
- Hartland RP, Vermeulen CA, Klis FM, et al. The linkage of (1-3)-β-glucan to chitin during cell wall assembly in Saccharomyces cerevisiae. Yeast. 1994;10:1591–1599.10.1002/(ISSN)1097-0061
- Bowman SM, Free SJ. The structure and synthesis of the fungal cell wall. BioEssays. 2006;28:799–808.10.1002/(ISSN)1521-1878
- Free SJ. Fungal cell wall organization and biosynthesis. Adv. Genet. 2013;81:33–82.10.1016/B978-0-12-407677-8.00002-6
- Levin DE. Cell wall integrity signaling in Saccharomyces cerevisiae. Microbiol. Mol. Biol. Rev. 2005;69:262–291.10.1128/MMBR.69.2.262-291.2005
- Kamei M, Banno S, Takahashi M, et al. Regulation and physiological function of MAP kinase and cAMP-PKA pathways. In: Kasbekar DP, McCluskey K, editors. Neurospora: genomics and molecular biology. Caister Academic Press: Norfolk; 2013. p. 171–192.
- Levin DE. Regulation of cell wall biogenesis in Saccharomyces cerevisiae: the cell wall integrity signaling pathway. Genetics. 2011;189:1145–1175.10.1534/genetics.111.128264
- Rispail N, Soanes DM, Ant C, et al. Comparative genomics of MAP kinase and calcium-calcineurin signalling components in plant and human pathogenic fungi. Fungal Genet. Biol. 2009;46:287–298.10.1016/j.fgb.2009.01.002
- Jung US, Levin DE. Genome-wide analysis of gene expression regulated by the yeast cell wall integrity signalling pathway. Mol. Microbiol. 1999;34:1049–1057.10.1046/j.1365-2958.1999.01667.x
- Xu JR, Staiger CJ, Hamer JE. Inactivation of the mitogen-activated protein kinase Mps1 from the rice blast fungus prevents penetration of host cells but allows activation of plant defense responses. Proc. Natl. Acad. Sci. USA. 1998;95:12713–12718.10.1073/pnas.95.21.12713
- Fujikawa T, Kuga Y, Yano S, et al. Dynamics of cell wall components of Magnaporthe grisea during infectious structure development. Mol. Microbiol. 2009;73:553–570.10.1111/mmi.2009.73.issue-4
- Valiante V, Heinekamp T, Jain R, et al. The mitogen-activated protein kinase MpkA of Aspergillus fumigatus regulates cell wall signaling and oxidative stress response. Fungal Genet. Biol. 2008;45:618–627.10.1016/j.fgb.2007.09.006
- Hou Z, Xue C, Peng Y, et al. A mitogen-activated protein kinase gene (MGV1) in Fusarium graminearum is required for female fertility, heterokaryon formation, and plant infection. Mol. Plant-Microbe Interact. 2002;15:1119–1127.10.1094/MPMI.2002.15.11.1119
- Bussink HJ, Osmani SA. A mitogen-activated protein kinase (MPKA) is involved in polarized growth in the filamentous fungus, Aspergillus nidulans. FEMS Microbiol. Lett. 1999;173:117–125.10.1111/fml.1999.173.issue-1
- Fujioka T, Mizutani O, Furukawa K, et al. MpkA-dependent and -independent cell wall integrity signaling in Aspergillus nidulans. Eukaryot. Cell. 2007;6:1497–1510.10.1128/EC.00281-06
- Galagan JE, Calvo SE, Borkovich KA, et al. The genome sequence of the filamentous fungus Neurospora crassa. Nature. 2003;422:859–868.10.1038/nature01554
- Borkovich KA, Alex LA, Yarden O, et al. Lessons from the genome sequence of Neurospora crassa: tracing the path from genomic blueprint to multicellular organism. Microbiol. Mol. Biol. Rev. 2004;68:1–108.10.1128/MMBR.68.1.1-108.2004
- Maerz S, Ziv C, Vogt N, et al. The nuclear Dbf2-related kinase COT1 and the mitogen-activated protein kinases MAK1 and MAK2 genetically interact to regulate filamentous growth, hyphal fusion and sexual development in Neurospora crassa. Genetics. 2008;179:1313–1325.10.1534/genetics.108.089425
- Zhang Y, Lamm R, Pillonel C, et al. Osmoregulation and fungicide resistance: the Neurospora crassa os-2 gene encodes a HOG1 mitogen-activated protein kinase homologue. Appl. Environ. Microbiol. 2002;68:532–538.10.1128/AEM.68.2.532-538.2002
- Fujimura M, Ochiai N, Oshima M, et al. Putative homologs of SSK22 MAPKK kinase and PBS2 MAPK kinase of Saccharomyces cerevisiae encoded by os-4 and os-5 genes for osmotic sensitivity and fungicide resistance in Neurospora crassa. Biosci. Biotechnol. Biochem. 2003;67:186–191.10.1271/bbb.67.186
- Noguchi R, Banno S, Ichikawa R, et al. Identification of OS-2 MAP kinase-dependent genes induced in response to osmotic stress, antifungal agent fludioxonil, and heat shock in Neurospora crassa. Fungal Genet. Biol. 2007;44:208–218.10.1016/j.fgb.2006.08.003
- Vitalini MW, de Paula RM, Goldsmith CS, et al. Circadian rhythmicity mediated by temporal regulation of the activity of p38 MAPK. Proc. Natl. Acad. Sci. USA. 2007;104:18223–18228.10.1073/pnas.0704900104
- Watanabe S, Yamashita K, Ochiai N, et al. OS-2 mitogen activated protein kinase regulates the clock-controlled gene ccg-1 in Neurospora crassa. Biosci. Biotechnol. Biochem. 2007;71:2856–2859.10.1271/bbb.70410
- Yamashita K, Banno S, Fukumori F, et al. Involvement of OS-2 MAP kinase in regulation of the large-subunit catalases CAT-1 and CAT-3 in Neurospora crassa. Genes Genet. Syst. 2007;82:301–310.10.1266/ggs.82.301
- Kamei M, Yamashita K, Takahashi M, et al. Deletion and expression analysis of beta-(1,3)-glucanosyltransferase genes in Neurospora crassa. Fungal Genet. Biol. 2013;52:65–72.10.1016/j.fgb.2012.12.001
- Pandey A, Roca MG, Read ND, et al. Role of a mitogen-activated protein kinase pathway during conidial germination and hyphal fusion in Neurospora crassa. Eukaryot. Cell. 2004;3:348–358.10.1128/EC.3.2.348-358.2004
- Li D, Bobrowicz P, Wilkinson HH, et al. A mitogen-activated protein kinase pathway essential for mating and contributing to vegetative growth in Neurospora crassa. Genetics. 2005;170:1091–1104.10.1534/genetics.104.036772
- Fleissner A, Leeder AC, Roca MG, et al. Oscillatory recruitment of signaling proteins to cell tips promotes coordinated behavior during cell fusion. Proc. Natl. Acad. Sci. USA. 2009;106:19387–19392.10.1073/pnas.0907039106
- Gras DE, Persinoti GF, Peres NT, et al. Transcriptional profiling of Neurospora crassa Δmak-2 reveals that mitogen-activated protein kinase MAK-2 participates in the phosphate signaling pathway. Fungal Genet. Biol. 2013;60:140–149.10.1016/j.fgb.2013.05.007
- Park G, Pan S, Borkovich KA. Mitogen-activated protein kinase cascade required for regulation of development and secondary metabolism in Neurospora crassa. Eukaryot. Cell. 2008;7:2113–2122.10.1128/EC.00466-07
- Bennett LD, Beremand P, Thomas TL, et al. Circadian activation of the mitogen-activated protein kinase MAK-1 facilitates rhythms in clock-controlled genes in Neurospora crassa. Eukaryot. Cell. 2013;12:59–69.10.1128/EC.00207-12
- Maddi A, Dettman A, Fu C, et al. WSC-1 and HAM-7 are MAK-1 MAP kinase pathway sensors required for cell wall integrity and hyphal fusion in Neurospora crassa. PLoS ONE. 2012;7:e42374.10.1371/journal.pone.0042374
- Richthammer C, Enseleit M, Sanchez-Leon E, et al. RHO1 and RHO2 share partially overlapping functions in the regulation of cell wall integrity and hyphal polarity in Neurospora crassa. Mol. Microbiol. 2012;85:716–733.10.1111/mmi.2012.85.issue-4
- Tamaru H, Inoue H. Isolation and characterization of a laccase-derepressed mutant of Neurospora crassa. J. Bacteriol. 1989;171:6288–6293.
- Colot HV, Park G, Turner GE, et al. A high-throughput gene knockout procedure for Neurospora reveals functions for multiple transcription factors. Proc. Natl. Acad. Sci. USA. 2006;103:10352–10357.10.1073/pnas.0601456103
- Vogel HJ. Distribution of lysine pathways among fungi: evolutionary implications. Am. Natural. 1964;98:435–446.10.1086/282338
- Yamashita K, Shiozawa A, Watanabe S, et al. ATF-1 transcription factor regulates the expression of ccg-1 and cat-1 genes in response to fludioxonil under OS-2 MAP kinase in Neurospora crassa. Fungal Genet. Biol. 2008;45:1562–1569.10.1016/j.fgb.2008.09.012
- Takahashi M, Yamashita K, Shiozawa A, et al. An AP-1-like transcription factor, NAP-1, regulates expression of the glutathione S-transferase and NADH:flavin oxidoreductase genes in Neurospora crassa. Biosci. Biotechnol. Biochem. 2010;74:746–752.10.1271/bbb.90790
- Lichius A, Lord KM, Jeffree CE, et al. Importance of MAP kinases during protoperithecial morphogenesis in Neurospora crassa. PLoS ONE. 2012;7:e42565.10.1371/journal.pone.0042565
- Chen RE, Thorner J. Function and regulation in MAPK signaling pathways: Lessons learned from the yeast Saccharomyces cerevisiae. Biochim. Biophys. Acta. 2007;1773:1311–1340.10.1016/j.bbamcr.2007.05.003
- Krishna M, Narang H. The complexity of mitogen-activated protein kinases (MAPKs) made simple. Cell. Mol. Life Sci. 2008;65:3525–3544.10.1007/s00018-008-8170-7
- Yoshimi A, Sano M, Inaba A, et al. Functional analysis of the α-1,3-glucan synthase genes agsA and agsB in Aspergillus nidulans: AgsB is the major α-1,3-glucan synthase in this fungus. PLoS ONE. 2013;8:e54893.10.1371/journal.pone.0054893
- Maddi A, Fu C, Free SJ. The Neurospora crassa dfg5 and dcw1 genes encode α-1,6-mannanases that function in the incorporation of glycoproteins into the cell wall. PLoS ONE. 2012;7:e38872.10.1371/journal.pone.0038872
- Montenegro-Montero A, Goity A, Larrondo LF. The bZIP transcription factor HAC-1 is involved in the unfolded protein response and is necessary for growth on cellulose in Neurospora crassa. PLoS ONE. 2015;10:e0131415.10.1371/journal.pone.0131415
- Roca MG, Arlt J, Jeffree CE, et al. Cell biology of conidial anastomosis tubes in Neurospora crassa. Eukaryot. Cell. 2005;4:911–919.10.1128/EC.4.5.911-919.2005
- Fu C, Iyer P, Herkal A, et al. Identification and characterization of genes required for cell-to-cell fusion in Neurospora crassa. Eukaryot. Cell. 2011;10:1100–1109.10.1128/EC.05003-11
- Dettmann A, Illgen J, März S, et al. The NDR kinase scaffold HYM1/MO25 is essential for MAK2 MAP kinase signaling in Neurospora crassa. PLoS Genet. 2012;8:e1002950.10.1371/journal.pgen.1002950
- Fu C, Ao J, Dettmann A, et al. Characterization of the Neurospora crassa cell fusion proteins, HAM-6, HAM-7, HAM-8, HAM-9, HAM-10, AMPH-1 and WHI-2. PLoS ONE. 2014;9:e107773.10.1371/journal.pone.0107773
- Jonkers W, Leeder AC, Ansong C, et al. HAM-5 functions as a MAP kinase scaffold during cell fusion in Neurospora crassa. PLoS Genet. 2014;10:e1004783.10.1371/journal.pgen.1004783
- Dettmann A, Heilig Y, Ludwig S, et al. HAM-2 and HAM-3 are central for the assembly of the Neurospora STRIPAK complex at the nuclear envelope and regulate nuclear accumulation of the MAP kinase MAK-1 in a MAK-2-dependent manner. Mol. Microbiol. 2013;90:796–812.10.1111/mmi.12399
- Maddi A, Free SJ. Alpha-1,6-mannosylation of N-linked oligosaccharide present on cell wall proteins is required for their incorporation into the cell wall in the filamentous fungus Neurospora crassa. Eukaryot. Cell. 2010;9:1766–1775.10.1128/EC.00134-10
- Klis FM, Brul S, De Groot PW. Covalently linked wall proteins in ascomycetous fungi. Yeast. 2010;27:489–493.
- Mrsa V, Klebl F, Tanner W. Purification and characterization of the Saccharomyces cerevisiae BGL2 gene product, a cell wall endo-beta-1,3-glucanase. J. Bacteriol. 1993;175:2102–2106.
- Martínez-Núñez L, Riquelme M. Role of BGT-1 and BGT-2, two predicted GPI-anchored glycoside hydrolases/glycosyltransferases, in cell wall remodeling in Neurospora crassa. Fungal Genet. Biol. 2015;85:58–70.10.1016/j.fgb.2015.11.001
- Kitagaki H, Wu H, Shimoi H, et al. Two homologous genes, DCW1 (YKL046c) and DFG5, are essential for cell growth and encode glycosylphosphatidylinositol (GPI)-anchored membrane proteins required for cell wall biogenesis in Saccharomyces cerevisiae. Mol. Microbiol. 2002;46:1011–1022.10.1046/j.1365-2958.2002.03244.x
- Grobler J, Bauer F, Subden RE, et al. The mae1 gene of Schizosaccharomyces pombe encodes a permease for malate and other C4 dicarboxylic acids. Yeast. 1995;11:1485–1491.10.1002/(ISSN)1097-0061
- Camarasa C, Bidard F, Bony M, et al. Characterization of Schizosaccharomyces pombe malate permease by expression in Saccharomyces cerevisiae. Appl. Environ. Microbiol. 2001;67:4144–4151.10.1128/AEM.67.9.4144-4151.2001
- Reese AJ, Doering TL. Cell wall α-1,3-glucan is required to anchor the Cryptococcus neoformans capsule. Mol. Microbiol. 2003;50:1401–1409.10.1046/j.1365-2958.2003.03780.x
- Reese AJ, Yoneda A, Breger JA, et al. Loss of cell wall alpha(1-3) glucan affects Cryptococcus neoformans from ultrastructure to virulence. Mol. Microbiol. 2007;63:1385–1398.10.1111/mmi.2007.63.issue-5
- Banno S, Noguchi R, Yamashita K, et al. Roles of putative His-to-Asp signaling modules HPT-1 and RRG-2, on viability and sensitivity to osmotic and oxidative stresses in Neurospora crassa. Curr. Genet. 2007;51:197–208.10.1007/s00294-006-0116-8
- Lamb TM, Goldsmith CS, Bennett L, et al. Direct transcriptional control of a p38 MAPK pathway by the circadian clock in Neurospora crassa. PLoS ONE. 2011;6:e27149.10.1371/journal.pone.0027149
- Lamb TM, Finch KE, Bell-Pedersen D. The Neurospora crassa OS MAPK pathway-activated transcription factor ASL-1 contributes to circadian rhythms in pathway responsive clock-controlled genes. Fungal Genet. Biol. 2012;49:180–188.10.1016/j.fgb.2011.12.006