Abstract
The SAD (synapses of amphids defective) kinases, including SAD-A and SAD-B, play important roles in the regulation of neuronal development, cell cycle, and energy metabolism. Our recent study of mouse SAD-A identified a unique autoinhibitory sequence (AIS), which binds at the junction of the kinase domain (KD) and the ubiquitin-associated (UBA) domain and exerts autoregulation in cooperation with UBA. Here, we report the crystal structure of the mouse SAD-B C-terminal fragment including the AIS and the kinase-associated domain 1 (KA1) at 2.8 Å resolution. The KA1 domain is structurally conserved, while the isolated AIS sequence is highly flexible and solvent-accessible. Our biochemical studies indicated that the SAD-B AIS exerts the same autoinhibitory role as that in SAD-A. We believe that the flexible isolated AIS sequence is readily available for interaction with KD-UBA and thus inhibits SAD-B activity.
Graphical abstract
The crystal structure and inhibition analysis of the SAD-B AIS-KA1 fragment.
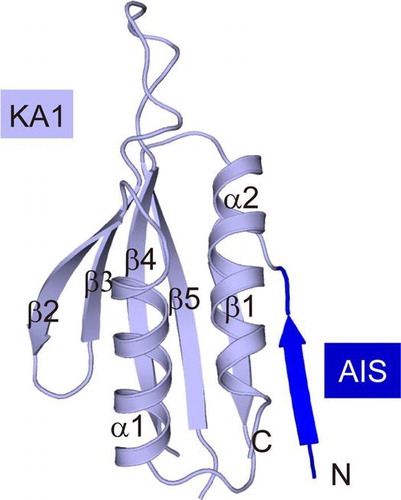
The serine/threonine kinases SAD-B and SAD-A (also named as BRSK1 and BRSK2, respectively) are two close homologues in mammal, which are highly expressed in the brain.Citation1) The pair of SAD kinases plays multiple, sequential roles in neurons, including controlling axonal arborization, promoting the maturation of nerve terminals, and modulating neurotransmitter release.Citation2–6) In addition, SAD-A is found to have a striking role in the energy metabolism in pancreas.Citation7–9) A series of studies have revealed that SAD-B is required for cell cycle progression. SAD-B can phosphorylate Cdc25-B/C and Wee1 to regulate mitosisCitation10); meanwhile SAD-B can control centrosome homeostasis of cell cycle through acting on γ–tubulin.Citation11,12) The yeast orthologs of SAD-B, Cdr2, and Hsl1 are critical mitosis-regulatory kinases for controlling mitotic entry.Citation13–15) The catalytic activities of SAD kinases are required for the output of their functions. Our recent studies have demonstrated that the kinase activity and function of SAD-A is strictly regulated by the C-terminal non-catalytic region.Citation16) However, despite the physiological importance, the regulatory mechanism of SAD-B remains elusive.
SAD-A/B belongs to the AMP-activated protein kinase (AMPK) family, which contains a conserved kinase domain (KD), an adjacent ubiquitin-associated (UBA) domain, and a kinase-associated domain 1 (KA1) in some members.Citation17) The N-terminal catalytic KDs of SAD-A and SAD-B are highly conserved, sharing 90% identical sequence, while the C-terminal non-catalytic region only owns 73% homology (Fig. (A)). In SAD-A, an autoinhibitory sequence (AIS), N-terminal to the KA1 domain, was identified as the truly inhibitory element.Citation16) The AIS sequence, adopting an extended conformation, makes multiple interactions with the KD-UBA fragment and reduces its activity by reinforcing the inactive conformation of the essential αC helix in SAD-A. In addition, the C-terminal region of its yeast ortholog Hsl1 has been suggested not only for intra-molecular inhibition, but also for activation through binding to other proteins, such as septins.Citation18) An equivalent AIS sequence is found in SAD-B. However, it is unclear whether the AIS of SAD-B also exerts an inhibitory role and what conformation would the isolated AIS adopt.
Fig. 1. Biochemical characteristics of SAD-B KD-UBA.
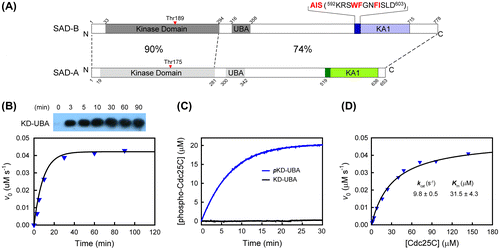
In this study, we first demonstrated that SAD-B could efficiently phosphorylate the downstream cell cycle-related protein Cdc25C. Then we determined the crystal structure of the free AIS-KA1 fragment (residues 592–719) of mouse SAD-B at 2.8 Å resolution (PDB ID: 5IRI). The KA1 domain is highly conserved with impact conformation, while the free AIS sequence in SAD-B is highly flexible, accessible to the KD-UBA interaction. Further biochemical data indicated that the AIS of SAD-B indeed plays an autoinhibitory role and its inhibitory mode is most likely the same as that in SAD-A. Therefore, our results revealed the flexible shift of AIS between the free state and the inhibitory state, implicating its regulatory role for controlling the kinase activity.
Materials and methods
Expression and purification of the proteins
The cDNA of mouse SAD-B was generously provided by Dr. Joshua R. Sanes (Harvard University). Various fragments of SAD-B (KD-UBA, residues 29–358; AIS-KA1, residues 592–719; KA1, residues 604–719) were subcloned into the vector pET21b (Novagen) using NdeI/XhoI restriction sites. The site-specific mutations were generated by overlap PCR and verified by DNA sequencing. The plasmids of human LKB1, STRADα, and MO25α were kindly provided by Dr. Gail Fraser and Dr. Maria Deak (University of Dundee), respectively. The active LKB1-STRAD-MO25 complex was expressed in Sf9 cells and purified as reported.Citation19) Cultures were grown in standard LB medium at 37 °C of an OD600 of approximately 0.6 and then induced with 0.2 mM isopropyl-β-D-thiogalactopyranoside (IPTG) at 18 °C for 12–16 h. The cells were harvested by centrifugation at 2500 g and resuspended in lysis buffer (50 mM Tris–HCl pH 8.0, and 300 mM NaCl) supplemented with 10 mM MgCl2, 200 Units/mL of DNase I, and 1 mM PMSF. The cells were lysed by sonication. The proteins containing a C-terminal His-tag were purified at 4 °C using Ni-NTA chromatography (Qiagen) and eluted with 300 mM imidazole. The eluates were then subjected to ion exchange chromatography (Source 15S, GE Healthcare) and eluted with a linear 0–1 M NaCl gradient. After concentration, the proteins were further purified using size exclusion chromatography (Superdex 200, GE Healthcare) and eluted with a buffer containing 10 mM HEPES (pH 7.0) and 150 mM NaCl in the presence of 2 mM dithiothreitol (DTT). The peak fractions were combined for stored at −80 °C and subjected to other trials.
X-ray crystallography
The crystals of the mouse SAD-B AIS-KA1 (592–719) were grown at 21 °C using the hanging-drop vapor diffusion method. Cubic crystals appeared in drops mixing the protein (approximately 5 mg/ml) with an equal volume of reservoir solution containing 0.1 M HEPES (pH 7.51), 3 M KCl, 0.01 M Urea after 3 days of growth. Crystals were equilibrated in a cryoprotectant buffer containing reservoir solution supplemented with 25% (v/v) ethylene glycol and then flash frozen under a cold nitrogen stream at 100 K. The diffraction data-sets were collected at 2.8 Å at beamline 17U of the Shanghai Synchrotron Radiation Facility (SSRF, Shanghai, China) and processed using HKL2000.Citation20) The structure was solved by molecular replacement and the SAD-A KD-UBA-AIS-KA1 structure (PDB ID: 4YOM) as the search model. Standard refinement was performed using PhenixCitation21) and Coot.Citation22) The data processing and refinement statistics were summarized in Table . All structural representations in this paper were prepared with PYMOL (http://www.pymol.org).
Table 1. Data collection and refinement statistics.
Phosphorylation of SAD-B by LKB1
To determine the phosphorylation time of SAD-B, the SAD-B KD-UBA (5 μM) was incubated with the LKB1 complex (20 nM) in a phosphorylation buffer (pH 7.0) containing 50 mM MOPS, 2 mM DTT, 100 mM NaCl, 10 mM MgCl2, and 1 mM ATP at 25 °C. The SAD-B KD-UBA (5 μM) was incubated with AIS-KA1 (100 μM) for 1 h, and then the complex of KD-UBA and AIS-KA1 was subjected to the LKB1-catalyzed phosphorylation assay. The reaction was initiated by adding LKB1. At indicated time intervals, aliquots were withdrawn and phosphorylation was terminated via adding EDTA to a final concentration of 50 mM. The samples were then subjected to western blot analysis to examine the phosphorylation state of the conserved Thr189 in the kinase activation loop of SAD-B and using an antibody against the phospho-Thr172 of AMPK (1:5,000; Cell Signalling Technologies, #2535). To generate fully phosphorylated proteins, SAD-B KD-UBA fragment was incubated with 20 nM LKB1 and 1 mM ATP for at least 60 min.
Kinetic analysis of SAD kinase activity
The enzymatic activity of SAD kinase was spectrophotometrically determined using the synthetic Cdc25C peptide (201DQEAKVSRSGLYRSPSMPENLNRPRLKQVE230, SciLight Biotechnology) as substrate, which can be phosphorylated at its Ser216.Citation10) The continuous spectrophotometric assay couples the production of ADP with the oxidation of NADH by pyruvate kinase and lactate dehydrogenase.Citation23,24) All experiments were performed at 25 °C in a 1.8 ml reaction mixture (pH 7.0 buffers containing 50 mM MOPS, 100 mM NaCl, 0.1 mM EDTA, 10 mM MgCl2, 0.2 mM NADH, 1 mM ATP, 1 mM phosphoenolpyruvate, 20 units/ml lactate dehydrogenase, 15 units/ml pyruvate kinase, and varying amounts of the Cdc25C peptide, 5 nM SAD-B KD-UBA). The reaction was initiated by the addition of the Cdc25C peptide to the reaction mixture. The progress of the reaction was continuously monitored by measuring the formation of NAD+ at 340 nm on a PerkinElmer Lambda 45 spectrophotometer (PerkinElmer Life Sciences) equipped with a magnetic stirrer in the cuvette holder. The initial rates were determined from the slopes of the progress curves, and the experimental data were analyzed using a nonlinear regression analysis program. The concentrations of ADP formed in the SAD-catalyzed reaction were determined with an extinction coefficient for NADH of 6,220 M−1 cm−1 at 340 nm. The concentration of the Cdc25C peptide was determined by finishing the reaction under conditions of limiting peptide. The kinetic parameters were obtained by fitting the experimental data to the Michaelis–Menten equation.
The trans-inhibition studies were performed at the 25 °C in a 1.8 ml kinase reaction mixture with indicated C-terminal fragments. The AIS peptide (592KRSWFGNFISLD603) was synthesized using standard protocol, purified by reverse-phase preparative HPLC and characterized by MALDI-TOF mass spectrometry by Scilight Biotechnology Ltd. (Beijing, China). The KD-UBA fragment of SAD-B (10 nM) was preincubated with indicated amount of C-terminal fragments in the reaction mixture for 15 min. The reaction was initialized by adding the substrate Cdc25C peptide (20 μM), Each data was fitted to the non-competitive inhibition equation vi/v0 = (Ki + a[I]/(Ki + [I]), where Ki and a are the apparent inhibition constant and residual activity, respectively.
Results and discussion
Biochemical characteristics of SAD-B KD-UBA
To understand the activity regulation of SAD-B, we first cloned and purified the KD-UBA fragment of mouse SAD-B. Like SAD-A, bacterially expressed SAD-B proteins were unphosphorylated. Since LKB1 complex has been reported as the upstream kinase of SAD-A/B and is required for the regulation of neuronal development,Citation6,25) we employed LKB1 for SAD-B phosphorylation (Fig. (B), top). The results showed that SAD-B could be readily phosphorylated at Thr189 by LKB1. The activity of SAD-B KD-UBA was determined using the Cdc25C peptide (201–230) as substrate, which can be phosphorylated by SAD-B at Ser216 (Fig. (B), bottom).Citation10) The unphosphorylated SAD-B were catalytically inactive, while the Thr189-phosphorylated SAD-B KD-UBA protein can efficiently phosphorylate Cdc25C peptide. The activity of SAD-B KD-UBA increased until it was completely phosphorylated (Figs. (B) and (C)).
Then we determined the kinetic parameters of the fully phosphorylated SAD-B KD-UBA fragment (pKD-UBA) toward the substrate Cdc25C peptide. Fig. (D) shows the dependence of the initial rate of the SAD-B-catalyzed phosphorylation on the Cdc25C peptide concentration. It can be seen that the phosphorylation of Cdc25C peptide catalyzed by pKD-UBA of SAD-B obeyed classical Michaelis–Menten kinetics. By fitting the Michaelis–Menten equation to the experimental data, the values of kcat and Km of SAD-B pKD-UBA were determined to be 9.8 ± 0.5 s−1 and 31.5 ± 4.3 μM, respectively. The kcat value is slightly lower than that of SAD-A pKD-UBA, whereas the Km value is three times higher. Therefore, the kcat/Km value for SAD-B KD-UBA was estimated as (0.31 μM−1 s−1) is approximately fivefold lower than that of SAD-A (1.62 μM−1 s−1),Citation16) yet Cdc25C is an efficient substrate for SAD-B, consistent with the critical role of SAD-B in cell cycle.Citation10–12)
Overall structure of AIS-KA1 of mouse SAD-B
We have demonstrated that the KD-UBA catalytic activity of SAD-A kinase can be inhibited by its C-terminal AIS sequence.Citation16) We found that SAD-B contains a highly conserved AIS, and determined the crystal structure of mouse SAD-B AIS-KA1 fragment at 2.8 Å resolution (Fig. (A)). The crystal structure of the AIS-KA1 fragment was solved by molecular replacement using the KA1 fragment structure of mouse SAD-A (PDB ID: 4YOM).Citation16) The crystal belongs to space group P42212. The final Rwork and Rfree were 22.1% and 26.5%, respectively. The root mean square deviations of bond lengths and angles from ideality were calculated to be 0.009 Å and 0.981°. These values are well within the accepted limits, indicating that the structure has tight stereochemical constraints. Ramachandran analysis was carried out using the MolProbity server (http://molprobity.biochem.duke.edu/).Citation26) Approximate 96% and 4% of residues were in the favored and allowed regions, respectively, and no residues were identified as outliers.
Fig. 2. Overall structure of AIS-KA1 of mouse SAD-B.
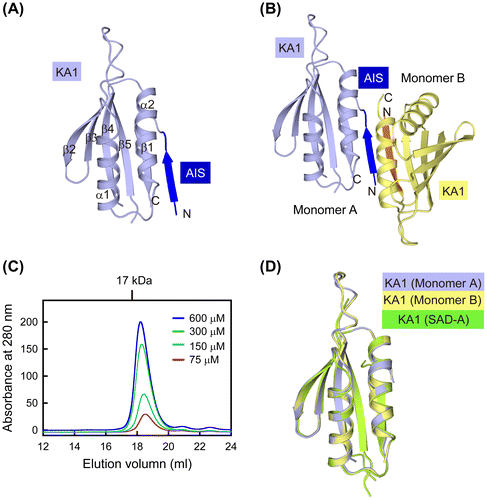
The structure of AIS-KA1 comprises two molecules (A and B) within an asymmetric unit, which forms a head-to-tail dimer (Fig. (B)). However, AIS-KA1 was suggested to be a monomeric protein in solution based on the results of gel filtration. In spite of the different protein concentrations, the retention time of SAD-B AIS-KA1 corresponded to an apparent molecular mass of ~15 kDa, in reasonable agreement with its calculated monomeric molecular mass (Fig. (C)). Therefore, the dimer observed in our crystal was probably due to crystallization artefacts.
The main part of our structure is the KA1 domain, which displays the canonical fold observed in other AMPK family members. The KA1-fold consists of two α-helices and a five-stranded antiparallel β-sheet (β1-α1-β2-β3-β4-β5-α2) (Fig. (A)). As shown in Fig. (D), the KA1 structures of SAD-B (both molecule A and molecule B) and that of SAD-A can be well superimposed with each other, with Cα position rmsd <0.5 Å. This compact KA1 conformation is stabilized by several highly conserved residues to form the hydrophobic core (Fig. (A)).
Fig. 3. Analysis of the AIS sequence in the free AIS-KA1 structure.
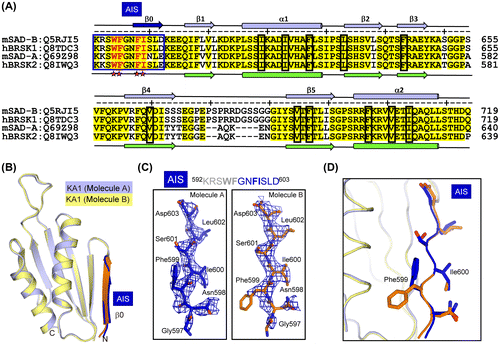
Analysis of the AIS sequence in the free AIS-KA1 structure
The AIS sequence in the SAD-A AIS-KA1 fragment is the key element for autoinhibition.Citation16) Sequence alignment of the AIS-KA1 fragments from SAD-A and SAD-B clearly reveals an equivalent AIS sequence in SAD-B. The inhibitory mechanism of AIS has been demonstrated in SAD-A, which tightly binds to the KD-UBA junction to stabilize the “αC-out” inactive kinase conformation.Citation16) Four key hydrophobic residues (Trp522, Phe523, Phe526, and Ile527) in the AIS of SAD-A have been proved to be indispensable for the binding and autoinhibition toward KD-UBA. In SAD-B, these four residues, Trp595, Phe596, Phe599, and Ile600, are strictly conserved (Fig. (A)).
In the free AIS-KA1 structure, a region of contiguous positive electron density (Fo–Fc) of ~23 Å in length was observed N-terminal to the KA1 domain in both molecules within an asymmetry unit (Figs. (B) and (C)). The C-terminal part of SAD-B AIS (597GNFISLD603) was assigned, while folds into a β-strand. This β strand, along with the α2 helix of the KA1 domain, contributes to the crystal packing within an asymmetry unit (Fig. (B)). Notably, the N-terminal five residues (592KRSWF596) are missing, including two of the key inhibitory residues, Trp595 and Phe596. The other two key inhibitory residues (Phe599 and Ile600) can be modeled based on the strong side-chain features. The side chains of Ile600 in both molecules A and B are pointing outwards and exposed to solvent (Fig. (D)). The aromatic rings of two Phe599 residues orient differently due to crystal packing and are facing the two α2-helices in the crystallographic dimer. Nevertheless, the Phe599 side chains would be solvent accessible in the monomeric AIS-KA1 fragment. Since the four inhibitory residues are either invisible or solvent-accessible, the AIS sequence is largely flexible in isolated the AIS-KA1 fragment and readily available to interact with the KD-UBA domain, thereby inhibiting the SAD activity.
Conserved autoinhibition of AIS
To investigate the regulatory role of AIS in SAD-B activity, we performed the trans-inhibition assay using various C-terminal fragments. The results clearly showed that the AIS-KA1 fragment (residues 592–719) and the chimeric protein containing the AIS sequence (592–603) effectively inhibited the kinase activity of pKD-UBA; however, the KA1 domain alone (residues 604–719) exhibited no inhibitory role on the pKD-UBA activity (Fig. (A)). In addition, the isolated AIS peptide suppressed the pKD-UBA activity effectively as well. These results clearly indicated that it is the AIS sequence, but not the KA1 domain, that down-regulates the SAD-B activity. The enzymatic activity of pKD-UBA decreased with the increase in the concentration of AIS-KA1 and approached a non-zero constant, plateauing at approximately 19%. The apparent dissociation constant, Ki, for this inhibition reaction was 7.57 ± 1.24 μM. These parameters were similar to that of AIS toward KD-UBA in SAD-A. Therefore, we believe that the AIS of SAD-B also non-competitively inhibited KD-UBA kinase activity against Cdc25C.Citation16) In addition, we investigated the LKB1-catalyzed Thr189-phosphorylation of KD-UBA in the presence of AIS-KA1 (Fig. (B)), which is comparable to that of KD-UBA in isolation. These data suggested that the AIS sequence of SAD-B only directly inhibits the catalytic activity of phosphorylated kinase, but has no effects on the LKB1-catalyzed phosphorylation.
Fig. 4. The conserved AIS inhibits the kinase activity of SAD-B.
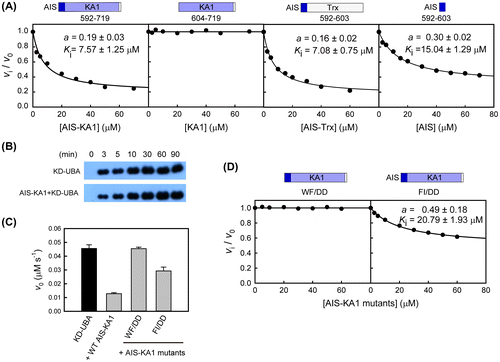
Considering the similar inhibitory effects and the highly conservation, we speculate that the AIS sequences from SAD-A and SAD-B may share the same inhibitory mechanism. To assess this hypothesis, we replaced the hydrophobic key residues by charged side chains and generated two double mutants (W595D/F596D, WF/DD and F599D/I600D, FI/DD) in the background of SAD-B AIS-KA1 fragment. We then examined their inhibitory effects on SAD-B pKD-UBA activity (Fig. (C)). The inhibition of wild type SAD-B AIS was disrupted by the AIS mutations, consist with the results for SAD-A mutations.Citation16) Notably, substitution of Trp595 and Phe596 (WF/DD) completely abolished the AIS inhibition on SAD-B activity (Fig. (D)). A dose-response curve based on increasing concentrations of the double mutant FI/DD demonstrated that the AIS inhibition was largely abolished with the incomplete inhibition plateauing at about 50% and Ki increased to 21.80 ± 1.93 μM. Together, these data demonstrated that these four hydrophobic residues of AIS also exert essential roles in SAD-B autoinhibition, and that the autoinhibitory mechanism of AIS is conserved between SAD-A and SAD-B.
In sum, we determined the crystal structure of the mouse SAD-B AIS-KA1 fragment at 2.8 Å resolution. The structural analyses indicated that the isolated AIS sequence is largely flexible and solvent accessible, and that AIS in the AIS-KA1 fragment is readily available for binding to the KD-UBA junction and inhibiting the SAD activity. Further biochemical studies demonstrated that the AIS in SAD-B uses the same inhibition mechanism as that in SAD-A. Taken together, the AIS sequence of SAD-B is flexible in the isolated state and characterized by the autoinhibitory role.
Author contributions
H.M., J.-X.W. and J.W. performed the experiments and analyzed the data. J.-W.W. and Z.-X.W. designed the research and wrote the manuscript with input from all authors.
Funding
This work was supported in part by the National Natural Science Foundation of China [grant number 31130062], [grant number 31321003] and the Ministry of Science and Technology of China [grant number 2013CB530600].
Disclosure statement
No potential conflict of interest was reported by the authors.
Acknowledgment
We thank the Shanghai Synchrotron Radiation Facility and the Tsinghua University Branch of China National Center for Protein Sciences (Beijing) for providing the facility support.
Notes
Abbreviations: AIS, autoinhibitory sequence; AMPK, AMP-activated protein kinase; BRSK, brain-specific kinase; E. coli, Escherichia coli; KA1, kinase-associated domain 1; KD, kinase domain; SAD, synapses of amphids defective; UBA, ubiquitin-associated domain.
References
- Kishi M, Pan YA, Crump JG, et al. Mammalian SAD kinases are required for neuronal polarization. Science. 2005;307:929–932.10.1126/science.1107403
- Inoue E, Mochida S, Takagi H, et al. SAD: a presynaptic kinase associated with synaptic vesicles and the active zone cytomatrix that regulates neurotransmitter release. Neuron. 2006;50:261–275.10.1016/j.neuron.2006.03.018
- Lilley BN, Krishnaswamy A, Wang Z, et al. SAD kinases control the maturation of nerve terminals in the mammalian peripheral and central nervous systems. Proc. Natl. Acad. Sci. USA. 2014;111:1138–1143.10.1073/pnas.1321990111
- Hagiwara A, Harada K, Hida Y, et al. Distribution of serine/threonine kinase SAD-B in mouse peripheral nerve synapse. NeuroReport. 2011;22:319–325.10.1097/WNR.0b013e328346013c
- Watabe AM, Nagase M, Hagiwara A, et al. SAD-B kinase regulates pre-synaptic vesicular dynamics at hippocampal Schaffer collateral synapses and affects contextual fear memory. J. Neurochem. 2016;136:36–47.10.1111/jnc.2016.136.issue-1
- Barnes AP, Lilley BN, Pan YA, et al. LKB1 and SAD kinases define a pathway required for the polarization of cortical neurons. Cell. 2007;129:549–563.10.1016/j.cell.2007.03.025
- Nie J, Sun C, Faruque O, et al. Synapses of amphids defective (SAD-A) kinase promotes glucose-stimulated insulin secretion through activation of p21-activated kinase (PAK1) in pancreatic β-Cells. J. Biol. Chem. 2012;287:26435–26444.10.1074/jbc.M112.378372
- Nie J, Lilley BN, Pan YA, et al. SAD-A potentiates glucose-stimulated insulin secretion as a mediator of glucagon-like peptide 1 response in pancreatic β cells. Mol. Cell Biol. 2013;33:2527–2534.10.1128/MCB.00285-13
- Nie J, Liu X, Lilley BN, et al. SAD-A kinase controls islet β-cell size and function as a mediator of mTORC1 signaling. Proc. Natl. Acad. Sci. USA. 2013;110:13857–13862.10.1073/pnas.1307698110
- Lu R, Niida H, Nakanishi M. Human SAD1 kinase is involved in UV-induced DNA damage checkpoint function. J. Biol. Chem. 2004;279:31164–31170.10.1074/jbc.M404728200
- Eklund G, Lang S, Glindre J, et al. The nuclear localization of γ-tubulin is regulated by SadB-mediated phosphorylation. J. Biol. Chem. 2014;289:21360–21373.10.1074/jbc.M114.562389
- Alvarado-Kristensson M, Rodríguez MJ, Silió V, et al. SADB phosphorylation of γ-tubulin regulates centrosome duplication. Nat. Cell Biol. 2009;11:1081–1092.10.1038/ncb1921
- Breeding CS, Hudson J, Balasubramanian MK, et al. The cdr2+ gene encodes a regulator of G2/M progression and cytokinesis in Schizosaccharomyces pombe. Mol. Biol. Cell. 1998;9:3399–3415.10.1091/mbc.9.12.3399
- Kanoh J, Russell P. The protein kinase Cdr2, related to Nim1/Cdr1 mitotic inducer, regulates the onset of mitosis in fission yeast. Mol. Biol. Cell. 1998;9:3321–3334.10.1091/mbc.9.12.3321
- Lew DJ. Cell-cycle checkpoints that ensure coordination between nuclear and cytoplasmic events in Saccharomyces cerevisiae. Curr. Opin. Genet. Dev. 2000;10:47–53.10.1016/S0959-437X(99)00051-9
- Wu J-X, Cheng Y-S, Wang J, et al. Structural insight into the mechanism of synergistic autoinhibition of SAD kinases. Nat. Commun. 2015;6:8953–8964.10.1038/ncomms9953
- Jaleel M, Villa F, Deak M, et al. The ubiquitin-associated domain of AMPK-related kinases regulates conformation and LKB1-mediated phosphorylation and activation. Biochem. J. 2006;394:545–555.10.1042/BJ20051844
- Hanrahan J, Snyder M. Cytoskeletal activation of a checkpoint kinase. Mol. Cell. 2003;12:663–673.10.1016/j.molcel.2003.08.006
- Zeqiraj E, Filippi BM, Deak M, et al. Structure of the LKB1-STRAD-MO25 complex reveals an allosteric mechanism of kinase activation. Science. 2009;326:1707–1711.10.1126/science.1178377
- Otwinowski Z, Minor W. [20] Processing of X-ray diffraction data collected in oscillation mode. Methods Enzymol. 1997;276:307–326.10.1016/S0076-6879(97)76066-X
- Adams PD, Grosse-Kunstleve RW, Hung L-W, et al. PHENIX: building new software for automated crystallographic structure determination, Acta Crystallogr. D Biol. Crystallogr 2002;58:1948–1954.10.1107/S0907444902016657
- Emsley P, Cowtan K. Model-building tools for molecular graphics. Acta Crystallogr., Sect D: Biol. Crystallogr. 2004;60:2126–2132.10.1107/S0907444904019158
- McClure W. Kinetic analysis of coupled enzyme assays. Biochemistry. 1969;8:2782–2786.10.1021/bi00835a014
- Roskoski R Jr. Assays of protein kinase. Methods Enzymol. 1983;99:3–6.10.1016/0076-6879(83)99034-1
- Lizcano JM, Göransson O, Toth R, et al. LKB1 is a master kinase that activates 13 kinases of the AMPK subfamily, including MARK/PAR-1. EMBO J. 2004;23:833–843.10.1038/sj.emboj.7600110
- Chen VB, Arendall WB, Headd JJ, et al. MolProbity: all-atom structure validation for macromolecular crystallography. Acta Crystallogr., Sect D: Biol. Crystallogr. 2010;66:12–21.10.1107/S0907444909042073