Abstract
This study aims to elucidate the mechanism of sexual development of basidiomycetous mushrooms from mating to fruit body formation. Sequencing analysis showed the TRP1 gene of basidiomycete Schizophyllum commune encoded an enzyme with three catalytic regions of GAT (glutamine amidotransferase), IGPS (indole-3-glycerol phosphate synthase), and PRAI (5-phosphoribosyl anthranilate isomerase); among these three regions, the trp1 mutant (Trp−) had a missense mutation (L→F) of a 338th amino acid residue of the TRP1 protein within the IGPS region. To investigate the function of IGPS region related to sexual development, dikaryons with high, usual, and no expression of the IGPS region of TRP1 gene were made. The dikaryotic mycelia with high expression of the IGPS formed mature fruit bodies earlier than those with usual and no expression of the IGPS. These results showed that the IGPS region in TRP1 gene promoted sexual development of S. commune.
Graphical abstract
The IGPS region of Schizophyllum commune TRP1 gene promoted its sexual development as well as complemented tryptophan auxotrophy originating from the trp1 mutation.
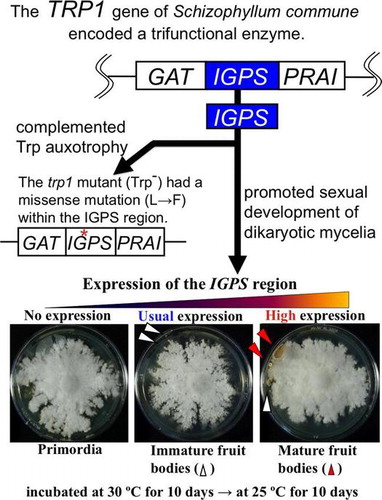
As mushrooms form fruit bodies only under a specific environment, it is not easy to establish their cultivation methods. Therefore, limited kinds of mushrooms are stably supplied. Increasing their yields and shortening of cultivation period are necessary to supply mushrooms more cheaply. Therefore, it is needed to elucidate the molecular mechanism of their sexual development. Sexual development of basidiomycetous mushrooms consists of stages of mating, hyphal aggregation, primordium formation, and fruit body formation. Progression of these stages is controlled by environmental factors and genetic factors. One of the pioneering researches was done by Uno et al.Citation1,2) Several genetic factors related to sexual development or fruit body formation are known, such as the FRT1 geneCitation3,4) in Schizophyllum commune, the ich1 gene,Citation5) and the Cc.rmt1 geneCitation6) in Coprinopsis cinerea as well as basidiomycete mating type genesCitation7,8); fruit body formation of basidiomycetous mushrooms is reviewed.Citation9–11) Furthermore, the signal transduction pathway for fruit body formation has also been studied.Citation10,12–16)
In this study, the function of one of the genetic factors involved in sexual development was investigated. Among basidiomycetes, S. commune is a mushroom whose genetic analysis is easy, and widely used as a model basidiomycete. Focusing on compounds of indole and caffeine involved in fruit body formation, we have isolated S. commune ind1 and cfn1 mutants which are resistant to high concentrations of indole and caffeine, respectively.Citation17) Interestingly, the ind1 and cfn1 mutations show resistances to different compounds. Nevertheless, both mutations are involved in expression of the B mating type factor of tryptophan-requiring (Trp−) strains of S. commune.Citation18) Moreover, the amount of cAMP in wild-type strains increases by the addition of indole and caffeine into media under several conditions.Citation19) Additionally, in order to elucidate the effects of tryptophan on a physiological condition of S. commune, it has been analyzed how extracellular tryptophan is taken into cells.Citation20) Both the ind1 and cfn1 mutations of S. commune cause abnormal sexual development (abnormal mating and abnormal nuclear migration) only in cells of Trp− strain, and conversely, existence of the tryptophan biosynthetic gene TRP1 brings abnormal sexual development by these mutations back to normal.Citation18) Indole is a precursor of tryptophan and a substance inducing fruit body formation; caffeine is an inhibitor of the phosphodiesterase (PDE) which degrades cAMP, another substance inducing fruit body formation. Namely, four compounds of indole, tryptophan, cAMP, and caffeine are key substances involved in signaling of the process of sexual development from mating to fruit body formation of basidiomycetes. Among them, researches on regulation of sexual development concerned by indole and tryptophan are unique, and this study is expected to open up a new area of morphogenesis and signaling.
In this study, the structure of S. commune TRP1 gene (tryptophan biosynthetic gene) and its function in its sexual development were analyzed. This paper reports that high expression of the IGPS region in TRP1 gene promoted sexual development of wild-type strains of S. commune.
Materials and methods
Strains and media
S. commune strains used in this study were shown in Table . S. commune T11 and T40 strains were used to isolate the genomic trp1 and TRP1 cDNA, respectively. All of the S. commune strains except T40 were used for analyzing sexual development. The strains were grown at 30 °C on the CYM,Citation17) supplemented with 4 mM tryptophan and 50 mg/L uracil (CYMTU) to maintain strains, to generate protoplasts, and to prepare their genomic DNA and total RNA from mycelia. The mycelia cultured on the MM supplemented with 50 mg/L tryptophan and 50 mg/L uracil (MMTU)Citation17) were used for an experiment of fruit body formation and preparation of total RNA. Escherichia coli DH10B was used for routine preparation of plasmids.
Table 1. S. commune strain used in this study.
Vectors, primers, and general procedures
The plasmid pGEM7+/TRP1 (kindly provided by Ullrich RC) includes the 4.5-kb EcoRI/HindIII fragment of TRP1 gene from S. commune 4–40 strain (Fig. )Citation21) being ligated into the corresponding sites of the plasmid vector pGEM-7Zf(+) (Promega Co.). The vectors of pGPDH-Zeo and pSC3-Zeo (constructed and kindly provided by YamagishiCitation13)) can express the Zeocin resistance factor (from ZeoCassette vectors, Invitrogen Co.) which is inserted between the promoter and terminator of GPDH (glyceraldehyde 3-phosphate dehydrogenase) geneCitation22) or those of the SC3 gene.Citation23) Primers used in this study were shown in Table .
Fig. 1. Restriction map of an insert DNA in the recombinant plasmid pGEM7+/TRP1.
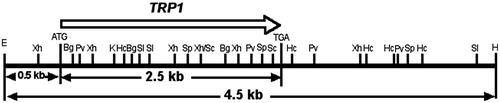
Table 2. Base sequences of primers used in this study.
General manipulations of DNA, such as restriction enzyme treatment, ligation, and dephosphorylation of plasmids were done according to the procedures of Sambrook et al.Citation24)
DNA sequencing
The cloned S. commune TRP1 gene and its subcloned fragments were sequenced using DSQ2000L sequencer (Shimadzu Co.) or ABI PRISM 310 genetic analyzer (PE Applied Biosystems). Nucleotide and amino acid sequences were analyzed using the GENETYX software system (Software Development, Tokyo, Japan). The deduced amino acid sequence of TRP1 was compared to the existing sequences using CLUSTAL W multiple sequence alignment (http://www.ddbj.nig.ac.jp).
Cloning of the TRP1 cDNA and genomic trp1 genes
Total RNA was extracted from mycelia according to the standard method of acid guanidinium thiocyanate-phenol-chloroform extraction, and then mRNA was purified by Oligotex-dT30<Super>(TAKARA Bio Inc.). Complementary DNA (cDNA) was obtained using mRNA with RNA LA PCR kit (TAKARA Bio Inc.) including the avian myeloblastosis virus (AMV) reverse transcriptase and the oligo dT-adaptor primer. On the other hand, genomic DNA for the template of PCR was isolated from lyophilized mycelia.Citation25) Two primers were designed: ScT5′s1 and ScT3′a2. The ScT5′s1 and ScT3′a2 can anneal the TRP1 initiation codon (ATG) and downstream of its termination codon (TGA), respectively, for which binding positions are shown in Fig. . Both the primers were used to amplify coding regions of TRP1 cDNA of T40 strain and trp1 genomic DNA of T11 strain. With each tube containing 120 ng of T40 1st strand cDNA or 100 ng of T11 genomic DNA as templates, PCR amplification was done as shown below; after denaturing at 98 °C for 30 s, 32 cycles of (94 °C for 15 s, 58 °C for 20 s, and 68 °C for 3 min), final incubation at 68 °C for 5 min, using AccuTaq LA DNA polymerase (Sigma Chemical Co.). PCR products from T40 (TRP1 cDNA) and T11 (trp1 genomic DNA) were cloned to the vector pT7Blue-T (Novogen, Co.), and the recombinant plasmids were named pT7-SccT and pT7-Sct, respectively.
Fig. 2. Nucleotide sequence of the S. commune TRP1 with flanking regions and predicted amino acid sequence of the TRP1 protein.
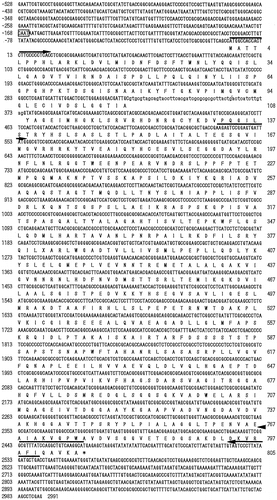
Construction of plasmids expressing the trp1 and TRP1 genes constitutively
The recombinant plasmids, pGEG-GIP and pSES-GIP, include the TRP1 gene in the order of the TRP1 PCR fragment from pGEM7+/TRP1 and the EGFP reporter gene between the promoter and terminator of either GPDH or SC3 genes, respectively. First, the coding region of TRP1 gene was amplified from a template of the pGEM7+/TRP1 plasmid DNA using a primer set of the ScT5′s1 and ScT805a with an addition of restriction sites EcoRI and BamHI, respectively. The antisense primer ScT805a does not include a termination codon. The amplified DNA fragment was digested at the EcoRI site, blunted by Mung Bean nuclease, digested at the BamHI site, and gel purified. Secondly, the EGFP coding region was amplified from the plasmid pEGFP-N1 (CLONTECH Co.) DNA using a primer set of EGFPs1 and EGFPa1 with addition of restriction sites XbaI and SpeI, respectively. The amplified fragment contained ATG initiation and termination codons. This DNA fragment was doubly digested at the native restriction site of BamHI and the SpeI site, and gel purified. Thirdly, vector DNAs of the pGPDH-Zeo and pSC3-Zeo were digested at the NcoI site, blunted by Mung Bean nuclease, digested at the XbaI site, and gel purified. These TRP1 fragment (5′-blunt, 3′-BamHI) and BamHI-SpeI fragment containing EGFP (5′-BamHI, 3′-SpeI) were ligated between the blunted site and the XbaI site of vector DNA of pGPDH-Zeo or pSC3-Zeo in a three-way ligation (both sites of XbaI and SpeI have the same sticky ends, 5′-CTAG-3′). Eventually, the Zeocin-resistant gene in the pGPDH-Zeo and pSC3-Zeo was replaced by the fusion gene of TRP1-EGFP.
The pSES-cGIP, including TRP1 cDNA and EGFP genes between the promoter and terminator of SC3 gene, could express the TRP1-EGFP fusion protein. This plasmid was constructed by replacing the chromosomal TRP1 fragment of pSES-GIP DNA with the TRP1 cDNA fragment of PvuII digest of the pT7-SccT plasmid DNA.
The pSES-mfGIP#7 was derived from pGEG-mGIP#4 replacing the trp1- specific mutation with same position of the TRP1 gene. Moreover, the trp1-specific mutation was introduced into the wild-type TRP1gene of pSES-GIP, giving the pSES-GImP.
Construction of the plasmid pAGA-I3 highly expressing the IGPS region
The DNA fragment containing the whole IGPS region of S. commune TRP1 gene was amplified from a template of the pGEM7+/TRP1 by PCR using a primer set of Sct162s-Nco and Sct527a-Nco with an addition of the restriction site NcoI for each. The PCR product was digested at the restriction site of NcoI and gel purified. On the other hand, vector DNA of pScAct-GFP, which was constructed in this study and includes the promoter and terminator of S. commune actin gene and the GFP reporter gene, was digested at the NcoI site and dephosphrylated. The DNA fragment of IGPS region was ligated at the NcoI site of pScAct-GFP, giving the recombinant plasmid pAGA-I3 (Fig. ). After transformation of E. coli by the pAGA-I3, orientation and base sequence of insert DNA (IGPS region) were verified. The pAGA-I3 can express the IGPS–GFP fusion protein under the control of the S. commune actin promoter.
Transformation of S. commune protoplast and confirmation of transformants
Transformation of S. commune was done according to the procedure of Froeliger et al.Citation26) and Yamagishi et al.Citation13) with some modification. For each transformation, 4 mg of plasmid DNA was cotransformed with 4 mg of the pGPDH-Zeo or pSC3-Zeo including the Zeocin selectable marker. In the case of selecting tryptophan-nonrequiring transformants, the equal volumes of 1 M MgSO4 and MMU containing 1% melted agar were mixed; the mixture was cooled to 42 °C and added to regenerated protoplast suspension, and the suspension was plated on the MMU agar and incubated at 30 °C for 3 days in the dark. In the case of selecting Zeocin-resistant transformants, the mixture of the equal volumes of 1 M MgSO4 and MMTU containing 1% melted agar was added to regenerated protoplast suspension with 200 mg/L Zeocin, and the suspension was plated to the MMTU agar containing the same concentration of Zeocin and incubated at 30 °C for 5 days in the dark.
Introduction of exogenously supplied DNA into transformants was confirmed by PCR. A fragment amplified from the transformant DNA was observed using a primer set of GPDH5′s and ScT1 ka annealing to the vector’s promoter and TRP1 region of the recombinant plasmids. Moreover, the expression of EGFP was directly observed under a fluorescence microscope (BX50 system, OLYMPUS Optical Co. Ltd.) with the fluorescence WIB cube.
When the highly expressed IGPS region of TRP1 gene was introduced to S. commune, transformation was done according to the methods of Bartholomew et al.Citation27) using S. commune protoplasts. Introduction of exogenously supplied DNA into transformants was confirmed by PCR, and observation of fluorescence of GFP was done in the same ways mentioned above.
Analysis of expression of the TRP1 and trp1 genes by RT-PCR
The aerial hyphae cultured on the CYMTU agar were collected, inoculated in the MMTU liquid medium, and then cultured at 30 °C for 5 days. The mycelia were filtered and inoculated to the MMU liquid media supplemented with 50 mg/L or 1000 mg/L tryptophan, and then cultured at 30 °C for 2 days. The 100 mg of the wet mycelia were triturated completely by a mortar and a pestle with 0.1 g of sea sand C in liquid nitrogen, and dried by an evaporator. Total RNA was prepared using the RNeasy plant mini kit (QIAGEN). DNA contaminated in 1 mg of total RNA was removed by the treatment of DNase I, and remaining RNA was reverse transcribed and cDNA was obtained using the RNA LA PCR Kit (AMV)(TAKARA Bio Inc.). RT-PCR fragments from the GPDH gene which is transcribed constitutively in vivo were amplified using a primer set of GPDH5′s and GPDH3′a, and then the amount of template RNA was adjusted so that the control band (RT-PCR products of GPDH transcript) of each sample gave the same brightness. In the same condition, the TRP1 or trp1 gene was amplified using a primer set of ScT162s and ScT805a, and then the brightness of each band amplified was compared.
Crossing and fruit body formation of S. commune
Crossing of monokaryotic strains of S. commune was done on the CYMTU agar at 30 °C in the dark,Citation17) and dikaryotic strains obtained from the side of nucleus recipient were used for an experiment of fruit body formation. Dikaryotic mycelia were inoculated on the MMTU or MMU agar, cultured at 30 °C for 10 days in the dark, and then cultured at 25 °C for further 20 days under laboratory lighting. Progression of sexual development of dikaryotic mycelia was evaluated (non-aggregated hyphae → semi-aggregated hyphae → aggregated hyphae → primordia → immature fruit bodies → mature fruit bodies).
Results
Sequencing analysis of S. commune TRP1
The sequence of insertion fragment of S. commune-E. coli shuttle vector pGEM7+/TRP1 was determined, and a restriction map was made (Fig. ). There was an open reading frame (ORF) of 2466 bases (529–2994th bases of the insertion fragment). The ORF and the flanking regions are shown in Fig. .
The ORF of genomic TRP1 and the cDNA of TRP1 from T40 (Trp+) strain were compared, and a single intron was found in the cloned TRP1 sequence at the position of nucleotides 324–374th bases from initiation codon. The cDNA fragment of TRP1 obtained from T40 (Trp+) strain was cloned into the pT7Blue T-vector, and the sequence of TRP1 cDNA was determined. Its length, position, splice junctions, and flanking sequences of the single intron were highly homologous to those of Phanerochaete chrysosporium trpC and Flammulina velutipes TRP1 (data not shown).
The ORF of 805 amino acid residues of S. commune TRP1 was homologous to Coprinus bilanatus TRP2 (70.7%),Citation28) F. velutipes TRP1 (64.9%),Citation29) Agaricus bisporus TRP2 (64.5%),Citation28) and P. chrysosporium trpC (60.9%).Citation30) The predicted amino acid sequence deduced from S. commune TRP1 was compared with those from the F. velutipes TRP1Citation29) and P. chrysosporium trpCCitation30) genes, and then it was confirmed that highly conserved sequences existed through the whole coding region (Fig. ). This result strongly suggested that the TRP1 gene in S. commune encoded a trifunctional enzyme consisting of three domains which corresponded to sequences encoding the GAT, IGPS, and PRAI.
Fig. 4. Comparison of the predicted amino acid sequence deduced for S. commune TRP1 protein to the corresponding sequences from other basidiomycetes.
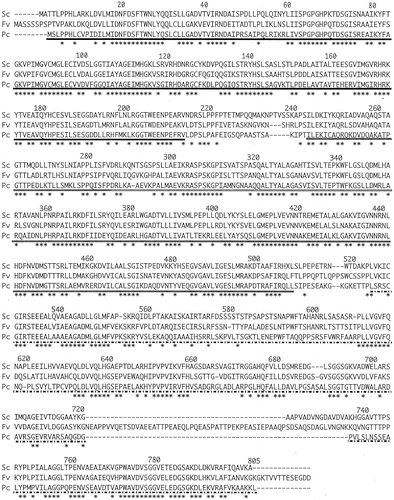
Cloning and sequencing analysis of the trp1 mutant gene of S. commune
Six clones of the trp1 were obtained as follows. A PCR product was amplified using a primer set of ScT5′s1 and ScT3′a2 that could amplify coding region of the trp1 gene from genomic DNA of S. commune T11 strain (Trp−), and ligated with the pT7Blue T-vector. As technical mutations sometimes occur during PCR amplification, three clones (trp1-2, trp1-4, and trp1-36) were completely sequenced, and their sequences were compared with the wild-type TRP1 sequence. This comparative analysis revealed a single point mutation (C → T) that produced a single amino acid replacement (L → F) at a 338th amino acid position within the IGPS region which was observed in all of the trp1 clones analyzed.
Effect of amino acid substitution of the TRP1 and trp1 gene products on their metabolic function
The pGEG-GIP and pSES-GIP including the genomic TRP1 were introduced into tryptophan-auxotrophic T11 strain. Transformants were screened on the MMU selection agar medium; a number of the tryptophan-nonrequiring transformants (Trp+) were obtained (Fig. ). Existence of the EGFP reporter gene in these recombinant plasmids was also confirmed by observation of glittering green fluorescence under a fluorescence microscope (data not shown). Moreover, the pSES-cGIP without the intron was introduced into T11 strain, and then transformants were screened on the MMU selection agar medium. As a result, six colonies of transformants were obtained. Among them, three transformants showed a clear appearance of EGFP.
Fig. 5. Six constructs of the S. commune TRP1 or trp1 genes/cDNA flanked with the GPDH or SC3 promoter and terminator.
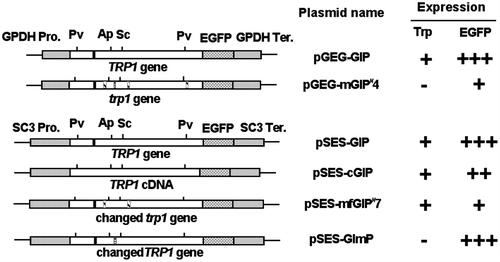
On the other hand, the pGEG-mGIP#4 that had a trp1-4 clone did not provide Trp+ transformants. The pGEG-mGIP#4 and pGPDH-Zeo were introduced into T11 strain by cotransformation, and six transformants (150 mg/L Zeocin-resistant strains) were obtained. Among them, two transformants showed a clear appearance of EGFP. Moreover, a single fragment was amplified by PCR using a primer set of GPDinsF (on the region of GPDH promoter) and ScT238a (on the TRP1 of S. commune) from genomic DNA of the two transformants, showing that pGEG-mGIP#4 was certainly introduced by cotransformation (data not shown). However, it was confirmed that a trp1-4 fragment cloned was not possible to complement the Trp− phenotype because these two transformants did not grow on the tryptophan-restricted MMU agar.
In order to disclose that a phenotype of tryptophan auxotrophy occurs by a missense mutation found in trp1 gene, the pSES-mfGIP#7 was constructed that was repaired only at the position of trp1-specific mutation. The restriction fragment between ApaI and SacI on pGEG-mGIP#4 did not include PCR mutations but the trp1-specific mutation. Therefore, 298 bp of ApaI-SacI fragment within the TRP1 gene on pSES-GIP and that within the trp1 gene on pGEG-mGIP#4 were replaced, and then the pSES-mfGIP#7 was constructed that could express the repaired trp1 under the control of SC3 promoter and terminator. The reason why the GPDH promoter was not used is that there were troublesome restriction sites in this promoter. The pSES-mfGIP#7 was introduced into T11 strain, giving five transformants which could grow on the MMU selection medium. These transformants showed weak fluorescence of EGFP, however, introduction of pSES-mfGIP#7 was confirmed by PCR.
By the same procedure above, the pSES-GImP was constructed that had the TRP1 gene with the trp1-specific mutation. Introduction of the pSES-GImP into T11 strain was tried thrice, but no transformants were obtained. Therefore, this experiment was done by cotransformation with the pGPDH-Zeo, giving four cotransformants which were 200 mg/L Zeocin resistant and also showed fluorescence of EGFP, however were still tryptophan requiring (Fig. ).
Transcriptional activity of the S. commune TRP1 and trp1 genes
The expression of tryptophan biosynthetic gene in Trp+ (T40) and Trp− (T11) strains, when added tryptophan adequately or too much, was evaluated by semi-quantitative RT-PCR (Fig. ). The GPDH gene is usually used as an internal standard. As for RT-PCR of the GPDH transcript, each of the cycles of PCR gave a single band when amplified using 1st strand cDNA of T11 and T40. These bands after 30 cycles reached a plateau for all samples of 1st strand cDNA. A quantity of 1st strand cDNA as template was adjusted to give the same brightness of DNA band after 25 cycles. Under this condition, RT-PCR of the TRP1 transcript (T40 strain) and the trp1 transcript (T11 strain) was done, giving a single band corresponding to full length of the TRP1 or trp1 gene. Here, all cDNA samples did not reach a plateau after 30 cycles. The intensity of the band after 30 cycles was same regardless of Trp+ or Trp− strains at the same concentration of tryptophan in medium, showing that the trp1 gene was transcribed in the same way as the TRP1 gene. Transcriptional level of both the TRP1 gene in T40 stain and the trp1 gene in T11 strain cultured at 1000 mg/L tryptophan was lower than that at 50 mg/L tryptophan.
Fig. 6. Comparison of the trp1 and TRP1 gene expression between Trp− and Trp+ strains by RT-PCR.
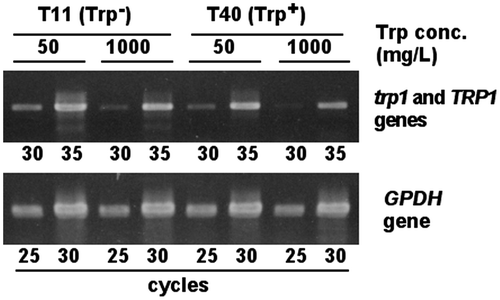
Comparison of secondary structure of the TRP1 and trp1 proteins
Secondary structure of the trp1 protein was estimated by the Chou-Fasman method and the Robson method, and was almost as the same structure of wild-type TRP1 protein (Fig. ).
Sexual development-promoting ability of the IGPS region of TRP1 gene
Preliminary experiments showed that a transcriptional activity of the actin gene promoter of S. commune was higher than that of the GPDH promoter, and that expression of both the GAT region and the PRAI region of S. commune TRP1 gene could not complement the tryptophan-requiring phenotype of trp1 mutation but the IGPS region could, under the control of the GPDH promoter (Fig. ). Therefore, pAGA-I3 plasmid bearing the highly expressed IGPS region of TRP1 gene driven by the actin promoter was introduced into S. commune monokaryotic strains T11 and T29. Tryptophan-nonrequiring transformants were screened and named T11/Act-IGPS transformants and T29/Act-IGPS transformants, respectively. Among them, T11/Act-IGPS#2, T11/Act-IGPS#3, T29/Act-IGPS#3, and T29/Act-IGPS#6 were provided for further analyses. Gene introduction into these monokaryotic transformants was confirmed by the PCR analysis using a primer set of ScActinF and ScActinR which anneals the promoter and terminator regions of actin gene, respectively. Mating types of the transformants were verified by the cross test.
Fig. 8. Complementation test of the tryptophan-requiring phenotype of trp1 mutation.
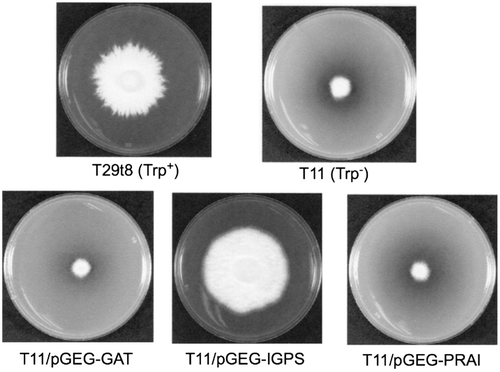
Dikaryotic strains (T11/Act-IGPS#2 × T29/Act-IGPS#3; T11/Act-IGPS#3 × T29/Act-IGPS#6) with high expression of the IGPS was made by crossing, and then an effect of the highly expressed IGPS region of TRP1 gene on sexual development of S. commune was investigated, being compared with controls of dikaryotic strains with no expression of the IGPS (trp1/trp1) and usual expression of the IGPS (TRP1/TRP1). The former was T11 × T29, and the latter was T11t17 × T29t8. The dikaryotic mycelia with high expression of the IGPS (T11/Act-IGPS#2 × T29/Act-IGPS#3) formed the mature fruit body at 10th day during 25 °C incubation, while the dikaryotic mycelia with no and usual expression of the IGPS formed it at 20th day. Another dikaryotic mycelia with high expression of the IGPS (T11/Act-IGPS#3 × T29/Act-IGPS#6) did not form the mature fruit body at 10th day during 25 °C incubation yet, however, the mycelia became thick and condensed same as T11/ Act-IGPS#2 x T29/Act-IGPS#3 (Fig. ). These results showed that high expression of the IGPS region in TRP1 gene promoted sexual development of S. commune.
Fig. 9. Sexual development of dikaryotic strains of S. commune with different levels of the IGPS expression.
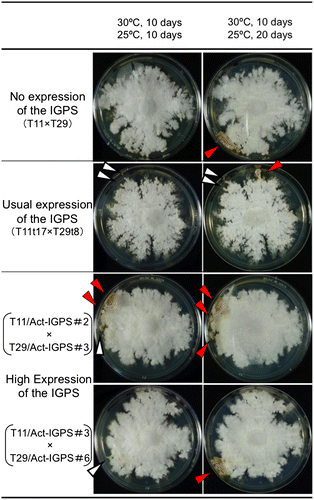
Effects of tryptophan, indole, and 3-indoleacetic acid on three types of dikaryotic mycelia
Three compounds on the tryptophan biosynthetic pathway (tryptophan, indole, 3-indoleacetic acid) were added to the MMU agar in case of tryptophan or the MMTU agar in case of the other two compounds, and sexual development of three types of dikaryotic mycelia with no, usual, and high expression of the IGPS was compared. When these compounds were added at high concentrations (equal or more than 1 mM), sexual development of all types of mycelia was repressed. On the other hand, when indole was added at a low concentration (0.05 mM), sexual development of mycelia with no IGPS expression was promoted, while that of mycelia with usual or high expression of the IGPS was repressed (Table ).
Table 3. Effect of indole on sexual development of dikaryotic mycelia of S. commune with different levels of the IGPS expression.
Discussion
The TRP1 gene of S. commune cloned by Muñoz-Rivas et al.Citation21) encoded the trifunctional enzyme of glutamine amidotransferase (GAT), indole-3-glycerol phosphate synthase (IGPS), and N-(5-phosphoribosyl) anthranilate isomerase (PRAI). Striking similarity in the GAT regions was found among the S. commune TRP1, P. chrysosporium trpC, and F. velutipes TRP1 genes. Moreover, introns in the GAT regions were highly conserved among these basidiomycetes. As for the other regions, the IGPS regions were also highly homologous, while the PRAI regions were lowlier homologous through the whole regions. However, we judged that this region of S. commune encoded PRAI because the TRP1 gene of S. commune can complement not only the defect of IGPS but also PRAI of E. coli,Citation21) the length of PRAI regions was similar, and high homology was observed at both the N and C termini of the regions between the S. commune TRP1 and P. chrysosporium trpC genes.
The gene expression of the S. commune TRP1 was not inhibited completely when tryptophan existed excessively (1000 mg/L) in a culture medium, but was clearly lower when 50 mg/L tryptophan was added. It is reported that a feedback inhibition by tryptophan does not exist in P. chrysosporium.Citation30) However, S. commune may have a system of feedback inhibition by tryptophan.
It was concluded that the trp1 mutation of S. commune Trp− mutant was a single missense mutation in the TRP1 gene. The amount of transcripts of the TRP1 gene in Trp+ strain was same as that of the trp1 gene in Trp− strain at the same concentrations of tryptophan in media. Moreover, it was certain that the trp1 protein must have been biosynthesized in S. commune cells because the trp1-EGFP fusion protein gave the fluorescence. It is indistinct whether the trp1 protein (mutant protein) has the GAT and/or PRAI activities, but it is clear that at least the wild-type IGPS region was necessary to transform trp1 mutant strain (Trp−) to Trp+ strain. Secondary structure of the trp1 protein was almost as the same structure of wild-type TRP1 protein. The information of missense mutation within the IGPS region of trp1 found out in this study is very useful for specifying an active center of IGPS.
The S. commune TRP1 proteins were expressed, even when the TRP1 gene did not have its single intron, and the TRP1 protein and the EGFP reporter protein were fused. Lugones et al.Citation31) reported that introns are necessary for transcription from DNA fragments used for transformation of S. commune. In this study, the introduction of TRP1 DNA without an intron could transform Trp− S. commune cells to Trp+.
The S. commune TRP1 gene not only has one function related to tryptophan biosynthesis, but also another function affecting the mating and fruit body formation of S. commune.Citation18) Here, we discuss the function of IGPS region related to sexual development. In this study, it was revealed that the high expression of IGPS region complemented a tryptophan-requiring phenotype originating from the trp1 mutation expectedly and also promoted sexual development of S. commune. How did it promote sexual development? One possibility is that the IGPS was one of the members on the signal transduction pathway for fruit body formation. However, it seems likely that the IGPS changed concentrations of some indole derivatives, thereby positively regulating sexual development. One of the candidates is indole because addition of 0.05 mM indole into the MMTU agar promoted sexual development of mycelia with no IGPS expression, while its addition repressed that with usual or high expression of the IGPS. In this case, a concentration of intracellular indole of mycelia with usual or high expression of the IGPS might become too high for desirable sexual development because the enzyme IGPS produces indole-3-glycerol phosphate which can be converted to indole and tryptophan by the tryptophan synthase (TS). Though addition of indole into the medium certainly promoted sexual development of S. commune, its improving effect was limited and smaller than that by introduction of the highly expressed IGPS region of TRP1 gene into S. commune cells. These phenomena might be due to some kind of the cross-pathway control. It is suggested that induction of the cross-pathway control inhibits sexual development in ascomycete Aspergillus nidluns.Citation32) It is also reported that the cross-pathway control is superimposed on leucine-specific regulation of fruiting body development in the leu1 mutant of ascomycete Sordaria macrospora.Citation33)
Indole-3-glycerol phosphate is a branchpoint of indole-3-acetic acid (IAA) biosynthesis from the tryptophan biosynthetic pathway in plants.Citation34) And, IAA is well known as a growth hormone of plants. This makes us speculate that indole itself or indole derivatives might play an important role also in basidiomycetes. Further analyses of the sexual development-promoting ability of the IGPS region of TRP1 gene and the signaling pathway related with indole derivatives will be done in order to elucidate the molecular mechanism of fruit body formation.
Author contributions
Kikuo Sen, Hideki Kinoshita, Toshiharu Yakushi, Hiroshiro Shibai, and Shin-ichi Kurosawa conceived and designed the experiments. Hideki Kinoshita, Kazuyuki Tazuke, Yoshinori Maki, and Yumi Yoshiura performed the experiments and analyzed the data. Kikuo Sen and Hideki Kinoshita wrote the paper.
Disclosure statement
No potential conflict of interest was reported by the authors.
Funding
This work was supported by Grant-in-Aid for Scientific Research (C) [Project number 15580073] from the Japan Society for the Promotion of Science (JSPS).
Acknowledgments
We wish to thank Robert C. Ullrich (University of Vermont, USA) for his kind supply of S. commune strains and the plasmid pGEM7+/TRP1; Kenji Yamagishi (National Agricultural Research Center for Tohoku Region, National Agricultural Research Organization, Japan) for his kind supply of the vectors of pGPDH-Zeo and pSC3-Zeo; and Yasuyoshi Sakai (Kyoto University, Japan) for his valuable discussion and suggestion about the EGFP marker.
References
- Uno I, Ishikawa T. Chemical and genetical control of induction of monokaryotic fruiting bodies in Coprinus macrorhizus. Mol. Gen. Genet. 1971;113:228–239.10.1007/BF00339543
- Uno I, Yamaguchi M, Ishikawa T. The effect of light on fruiting body formation and adenosine 3′:5′-cyclic monophosphate metabolism in Coprinus macrorhizus. Proc. Natl. Acad. Sci. USA. 1974;71:479–483.10.1073/pnas.71.2.479
- Horton JS, Raper CA. The mushroom-inducing gene Frt1 of Schizophyllum commune encodes a putative nucleotide-binding protein. Mol. Gen. Genet. 1995;247:358–366.10.1007/BF00293204
- Horton JS, Palmer GE, Smith WJ. Regulation of dikaryon-expressed genes by FRT1 in the basidiomycete Schizophyllum commune. Fung. Genet. Biol. 1999;26:33–47.10.1006/fgbi.1998.1104
- Muraguchi H, Kamada T. The ich1 gene of the mushroom Coprinus cinerea is essential for pileus formation in fruiting. Development. 1998;125:3133–3141.
- Nakazawa T, Tatsuta Y, Fujita T, et al. Mutations in the Cc.rmt1 gene encoding a putative protein arginine methyltransferase alter developmental programs in the basidiomycete Coprinopsis cinerea. Curr. Genet. 2010;56:361–367.10.1007/s00294-010-0307-1
- Kothe E. Mating-type genes for basidiomycete strain improvement in mushroom farming. Appl. Microbiol. Biotechnol. 2001;56:602–612.10.1007/s002530100763
- Raudaskoski M, Kothe E. Basidiomycete mating type genes and pheromone signaling. Eukaryot. Cell. 2010;9:847–859.10.1128/EC.00319-09
- Kues U. Life history and developmental process in the basidiomycete Coprinus cinereus. Microbiol. Mol. Biol. Rev. 2000;64:316–353.10.1128/MMBR.64.2.316-353.2000
- Palmer GE, Horton JS. Mushrooms by magic: making connections between signal transduction and fruiting body development in the basidiomycete fungus Schizophyllum commune. FEMS Microbiol. Lett. 2006;262:1–8.10.1111/fml.2006.262.issue-1
- Shishido K. Molecular mechanism of fruiting-body formation in the basidiomycete mushroom. Nippon Nogei Kagaku Kaishi. 2002;76:951–953. [Japanese] 10.1271/nogeikagaku1924.76.951
- Ishibashi O, Shishido K. Nucleotide sequence of a ras gene from the basidiomycete Coprinus cinereus. Gene. 1993;125:233–234.10.1016/0378-1119(93)90335-Z
- Yamagishi K, Kimura T, Suzuki M, et al. Suppression of fruit-body formation by constitutively active G-protein α-subunits ScGP-A and ScGP-C in the homobasidiomycete Schizophyllum commune. Microbiology. 2002;148:2797–2809.10.1099/00221287-148-9-2797
- Yamagishi K, Kimura T, Suzuki M, et al. Elevation of intracellular cAMP levels by dominant active heterotrimeric G protein alpha subunits ScGP-A and ScGP-C in homobasidiomycete Schizophyllum commune. Biosci. Biotechnol. Biochem. 2004;68:1017–1026.10.1271/bbb.68.1017
- Yamagishi K, Kimura T, Suzuki M, et al. Identification and overexpression of genes encoding cAMP-dependent protein kinase catalytic subunits in homobasidiomycete Schizophyllum commune. Biosci. Biotechnol. Biochem. 2005;69:2333–2342.10.1271/bbb.69.2333
- Knabe N, Jung E, Freihorst D, et al. A central role for Ras1 in morphogenesis of the basidiomycete Schizophyllum commune. Eukaryot. Cell. 2013;12:941–952.10.1128/EC.00355-12
- Samadder PP, Sen K, Kurosawa S. Isolation and characterization of Schizophyllum commune mutants resistant to indole and caffeine. FEMS Microbiol. Lett. 1997;150:277–282.10.1016/S0378-1097(97)00131-6
- Samadder PP, Sen K, Kurosawa S. Indole- and caffeine-resistant mutations of Schizophyllum commune are involved in the behavior of a class III B mating-type factor in trp1 cells. FEMS Microbiol. Lett. 1998;163:113–120.10.1111/fml.1998.163.issue-2
- Kinoshita H, Sen K, Iwama H, et al. Effects of indole and caffeine on cAMP in the ind1 and cfn1 mutant strains of Schizophyllum commune during sexual development. FEMS Microbiol. Lett. 2002;206:247–251.10.1111/fml.2002.206.issue-2
- Kinoshita H, Maki Y, Nakai R, et al. Competitive amino acid transport between l-tryptophan and other amino acids in Schizophyllum commune. J. Biosci. Bioeng. 2001;92:556–559.10.1016/S1389-1723(01)80315-7
- Muñoz-Rivas AM, Specht CA, Ullrich RC, et al. Isolation of the DNA sequence coding indole-3-glycerol phosphate synthetase and Phosphoribosylanthranilate isomerase of Schizophyllum commune. Curr. Genet. 1986;10:909–913.10.1007/BF00398288
- Harmsen MC, Schuren FHJ, Moukha SM, et al. Sequence analysis of the glyceraldehyde-3-phosphate dehydrogenase genes from the basidiomycetes Schizophyllum commune, Phanerochaete chrysosporium and Agaricus bisporus. Curr. Genet. 1992;22:447–454.10.1007/BF00326409
- Schuren FH, Wessels JG. Two genes specifically expressed in fruiting dikaryons of Schizophyllum commune: homologies with a gene not regulated by mating-type genes. Gene. 1990;90:199–205.10.1016/0378-1119(90)90180-Y
- Sambrook J, Fritsch EF, Maniatis T. Molecular cloning: a laboratory manual. 2nd ed. Cold Spring Harbor, NY: Cold Spring Harbor Laboratory Press; 1989.
- Lichtenstein C, Draper J. Genetic engineering of plants. In: Glover DM, editor. DNA Cloning: a practical approach. Vol. 2. Oxford: IRL Press; 1986. p. 67–119.
- Froeliger EH, Muñoz-Rivas AM, Specht CA, et al. The isolation of specific genes from the basidiomycete Schizophyllum commune. Curr. Genet. 1987;12:547–554.10.1007/BF00419565
- Bartholomew KA, Marion AL, Novotny CP, et al. A case study in fungal development and genetics: Schizophyllum commune. In: Bos CJ, editor. Fungal genetics: principles and practice. New York, NY: Marcel Dekker, Inc.; 1996. p. 371–384.
- Challen MP, Zhang C, Elliott TJ. Agaricus bisporus and Coprinus bilanatus TRP2 genes are tri-functional with conserved intron and domain organisations. FEMS Microbiol. Lett. 2002;208:269–274.10.1111/fml.2002.208.issue-2
- Nakai R, Sen K, Kurosawa S, et al. Cloning and sequencing analysis of TRP1 gene of Flammulina velutipes. FEMS Microbiol. Lett. 2000;190:51–56.10.1111/fml.2000.190.issue-1
- Schrank A, Tempelaars C, Sims PF, et al. The trpC gene of Phanerochaete chrysosporium is unique in containing an intron but nevertheless maintains the order of functional domains seen in other fungi. Mol. Microbiol. 1991;5:467–476.
- Lugones LG, Scholtmeijer K, Klootwijk R, et al. Introns are necessary for mRNA accumulation in Schizophyllum commune. Mol. Microbiol. 1999;32:681–689.10.1046/j.1365-2958.1999.01373.x
- Eckert SE, Hoffmann B, Wanke C, et al. Sexual development of Aspergillus nidulans in tryptophan auxotrophic strains. Arch. Microbiol. 1999;172:157–166.10.1007/s002030050755
- Ulrich K. A Sordaria macrospora mutant lacking the leu1 gene shows a developmental arrest during fruiting body formation. Mol. Genet. Genomics. 2005;274:307–315.
- Ouyang J, Shao X, Li J. Indole-3-glycerol phosphate, a branchpoint of indole-3-acetic acid biosynthesis from the tryptophan biosynthetic pathway in Arabidopsis thaliana. Plant J. 2000;24:327–333.10.1046/j.1365-313x.2000.00883.x