Abstract
AmfS, a class III lantipeptide serves as a morphogen in Streptomyces griseus. Here, we constructed a high production system of AmfS in S. griseus. We isolated S. griseus Grd1 strain defective in glucose repression of aerial mycelium formation and found it suitable for the overproduction of AmfS. Two expression vectors carrying the strong and constitutive ermE2 promoter were constructed using a multicopy number plasmid, pIJ702. The use of the Grd1 strain combined with the expression vectors enabled high production of AmfS by S. griseus into its culture broth. The expression system was also effective for the generation of abundant AmfS derived from Streptomyces avermitilis. In addition, site-directed mutagenesis revealed the amino acid residues essential for the morphogen activity of AmfS. These results indicate that the constructed system enables efficient production of class III lantipeptides by Streptomyces.
Graphical abstract
High production of a class III lantipeptide AmfS in Streptomyces griseus.
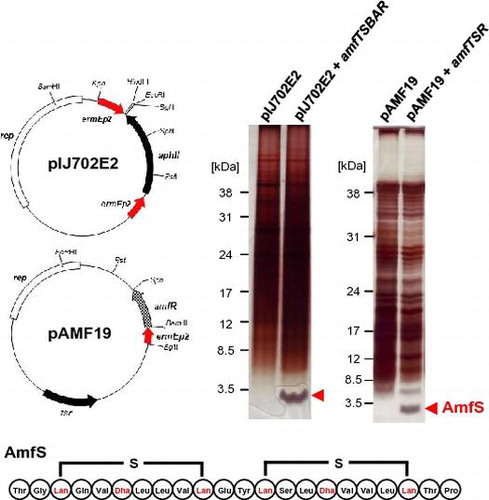
Key words:
Streptomyces, a high G+C content soil-dwelling Gram-positive bacterium, not only produces the diverse secondary metabolites but also undergoes complex morphological differentiation similar to that of fungi.Citation1–3) Diverged bioactive compounds have been found from Streptomyces metabolites including useful antibiotics, pigments, and other biologically active substances with important applications in the pharmaceutical industry.Citation2) Recent increases in available genomic data have revealed that Streptomyces strains retain dormant genes responsible for biosynthesis of diverse secondary metabolites.Citation4,5)
Lantipeptides (lanthionine-containing peptides) are ribosomally synthesized, post-translationally modified peptides that are produced by diverged Gram-positive bacteria including lactococci, staphylococci, and actinomycetes.Citation6) Lantipeptides represented by Nisin (class I lantipeptide) mainly function as an antimicrobial agent, and has application to be a food preservative.Citation7) Lantipeptides are natural polycyclic peptide products consisting of 18–38 amino acids, and include unusual amino acids and thioether rings, which consist of the diamino diacids, lanthionine (Lan), or methyllanthionine.Citation6) These unique structures are synthesized by the dehydration of Ser to 2,3-didehydroalanines (Dha) and Thr to 2,3-didehydrobutyrines (Dhb) followed by Michael-type addition of Cys to the 2,3-didehydro amino acids.
Lantipeptides have been categorized into four classes based on their biosynthetic pathways.Citation6,8) The precursor peptides of class III lantipeptides are modified by LanKC, a bi-functional modifying enzyme responsible for both dehydration and cyclization; LanKC exhibits a three-domain structure: an N-terminal phosphothreonine lyase, a central protein kinase, and a C-terminal putative cyclase domain.Citation6) The most well-known biological activity of a class III lantipeptide is the morphogen activity of SapB from Streptomyces coelicolor A3(2), which functions as a biological surfactant to lower the surface tension of substrate mycelium in order to help erect aerial mycelium.Citation9) Other biological functions of class III lantipeptide that have been reported include: anti-allodynic/antinociceptive (Labyrinthopeptin and NAI-112),Citation10,11) antiviral (Labyrinthopeptin),Citation11) and limited or weak antibiotic activity (SapT and NAI-112).Citation10,12)
AmfS, a SapB counterpart from Streptomyces griseus that produces streptomycin, was originally identified as an extracellular morphogenic peptide that stimulates its aerial growth.Citation13,14) The amfS gene is encoded in the amf cluster (amfTSBAR), which consists of five sequences encoding a lanthionine synthetase similar to LanKC (AmfT), a lantipeptide precursor (AmfS), two HlyB-type ABC transporters (AmfB and AmfA), and a response regulator for a two-component regulatory system (AmfR) (Fig. (A)).Citation13) A homologous gene cluster is distributed to the genome of S. coelicolor (ram cluster)Citation15–17) and S. avermitilis (amf cluster)Citation5). The expression of amfR is induced by the direct binding of AdpA, the A-factor (2-isocapryloyl-3R-hydroxymethyl-γ-butyrolactone) dependent global regulator for morphological differentiation and secondary metabolite production.Citation18) The expressed AmfR protein binds to the promoter region of amfT to direct transcription of the amfTSBA operon. Based on the knowledge regarding SapB and related peptides, the predicted molecular mechanism for AmfS is as follows: the precursor of AmfS undergoes post-translational modification by AmfT, and is exported by the putative exporters AmfA and AmfB. The modified precursor may be processed by a protease, and the mature peptide is then secreted into the substrate mycelium to lower its surface tension, which allows the mycelium to erect into the air.
Fig. 1. Schematic representation of the class III lantipeptide biosynthetic gene clusters in Streptomyces and related bacteria and the amino acid sequences of the precursor AmfS.
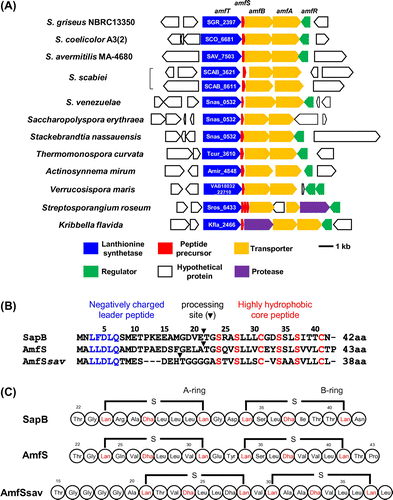
Genomic information accumulating in recent years uncovered a finding that about half of biosynthesis genes of Streptomyces secondary metabolites are cryptic. This suggests that the gene clusters for useful bioactive compounds have not yet been fully identified in Streptomyces and related bacteria. Several approaches to stimulate or express Streptomyces dormant genes have been developed, including: (1) ribosome engineering,Citation19) (2) elicitation,Citation20–22) (3) heterologous production,Citation4,23–25) (4) constitutive expression of master regulator,Citation26,27) and (5) co-cultivation.Citation28) While the biosynthesis and morphogen activities of class III lantipeptides have been well studied, few investigations have focused on improving the efficiency of lantipeptide production.Citation29) A boost in their production would greatly facilitate further scientific discoveries and advancements surrounding the use of these lantipeptides. Thus, we believe that the construction of a system for high production of AmfS contributes to furthering the discovery of lantipeptides produced by Streptomyces and related bacteria. Herein, we report a system for high production of AmfS derived from S. griseus and S. avermitilis.
Materials and methods
Bacterial strains, plasmids, chemicals, and culture media
S. griseus NBRC13350 (formerly known as IFO13350) and S. avermitilis MA-4680 were used as wild-type strains, and were obtained from the Institute of Fermentation, Osaka, Japan, and Kitasato Institute culture collection, respectively. The amfS mutant (designated ΔamfS) carrying a nonsense mutation in amfS was constructed in a previous study.Citation14) Escherichia coli K-12 strains, HST08, and GM2163 were used for general cloning and plasmids propagating. E. coli BL21(DE3) carrying pUB307-aph::Tn7 was used for E. coli/S. avermitilis conjugation.Citation30) pIJ702, a Streptomyces high-copy number plasmid (approximately 40–100 copies per chromosome) harboring thiostrepton resistance and melanin biosynthesis gene was used as a cloning and expression vector in S. griseus.Citation31) pKU250 (an SCP2*-derived E. coli-Streptomyces shuttle vector carrying thiostrepton resistance gene), has a copy number of ~1 per genome, and was used for gene disruption by allelic replacement owing to its unstable replication in Streptomyces.Citation23) A phiC31 integrase-based chromosome integration plasmid, pIJ8660,Citation32) was used to construct pNU10. For general cloning in E. coli, pMD19, pUC19, and pUC19BglII (with HincII site substituted with a BglII site), were used. pSPO1 plasmid carrying the 9-kb Sau3AI fragment containing the entire amf cluster of S. griseus at the BamHI site of pIJ487 enabled recovery of the Sau3AI region as an EcoRI-HindIII fragment.Citation33) Cosmid clones, CL_238_H01, and CL_215_B03, carrying the entire amf cluster of S. avermitilis, were used as a DNA source for the amf cluster. The culture conditions and genetic manipulation protocols used for E. coli and Streptomyces spp. were performed as described by Maniatis et al. (1982) and Kieser et al. (2000),Citation34,35) respectively. Strains of Streptomyces spp. were grown in Bennett’s/sugar medium (containing [g/L]: yeast extract [Becton, Dickinson and Co., Sparks, MD], 1; fish extract [Kyokuto, Tokyo, Japan], 1; NZ amine [Wako Pure Chemical Industries, Ltd., Osaka City, Osaka Pref., Japan], 2; and an appropriate sugar [Kokusan, Tokyo, Japan], 10 [pH 7.2]), YMP/sugar medium (containing [g/L]: yeast extract [Becton, Dickinson and Co.], 2; meat extract [Kyokuto], 2; Bacto-peptone [Becton, Dickinson and Co.], 4; NaCl, 5; MgSO4.7H2O, 2; and an appropriate sugar [Kokusan], 10), Algae-maltose medium (containing [g/L]: algae extract [Chlorella industry, Tokyo, Japan], 10; NaCl, 5; MgSO4.7H2O, 2; maltose, 10 [pH 7.2]), Minimal medium (containing [g/L]: urea, 1; K2HPO4, 1; NaCl, 0.5; MgSO4.7H2O, 0.5; FeSO4.7H2O, 0.01; glucose, 10 [pH 7.2]), YMS medium supplemented with 10 mM MgCl2 and 8 mM Ca(NO3)2 (PMID: 3680172), Nutrient broth (DIFCO), and R2YE medium.Citation34) Agar (1.5%; Kokusan) was added to the above mixtures to obtain solid media. To select the transformants and maintaining the plasmids in Streptomyces and E. coli, antibiotics were used at the following concentrations: kanamycin (Wako Pure Chemical) 25 μg/mL; ampicillin (Wako Pure Chemical Ind.) 25 μg/mL; thiostrepton (SIGMA-ALDRICH Corp, St. Louis, MO, USA) 20 μg/mL. All oligonucleotides were purchased from Eurofins Genomics K.K. (Tokyo, Japan). Enzymes used for DNA manipulation were purchased from Takara Bio Inc. (Shiga, Japan) and New England BioLabs (Ipswich, MA).
UV mutagenesis
Spores of S. griseus wild type were irradiated with long wavelength UV light to give approximately 1% survival. The cells were spread on YMP-10% glucose medium and incubated at 28 °C for 7 days. Among ~5000 colonies, 20 spore-forming mutants were selected. A strain out of 20 mutants that efficiently produced AmfS on minimal 1% glucose medium was selected and designated as Grd1 (glucose-repression deficient) strain.
Construction of pSPO vectors
The pIJ702E2 expression vector was constructed as follows. This vector carries two ermE2 promoters (ermEp2) and a kanamycin resistance gene aphII. To fuse the aphII sequence with the ermEp2 derived from pIJ2923ermE-pro, aphII, and the ermEp2 were individually amplified by polymerase chain reaction (PCR) with the primer sets, aphII-F/aphII-R, and PermE2-FB/PermE2-RB (Supplementary Table S1), respectively. The DNA fragments of the ermEp2 and aphII were cut by BglII and BamHI, respectively. After the ligation of both fragments, the ermEp2-aphII fusion was selectively amplified using PermE2-FB/aphII-R primers, and cloned into the NdeI-BglII sites in pIJ702, yielding pIJ702E. Another ermEp2 was amplified by PCR using the PermE2-FA/PermE2-RA primers. The amplicon was digested by KpnI-BglII, and inserted into the same sites in pIJ702E, giving rising to pIJ702E2.
The expression vector pAMF19 was constructed as follows. The wild-type ermEp2 and amfR were amplified by PCR with PermE2-FC/PermE2-RC and amfR-F/amfR-R primer sets, respectively. The amplicons were digested by BamHI, and then ligated. The fused DNA fragment consisting of ermEp2-amfR was selectively amplified using the PermE2-FC/amfR-R primers. The BglII and SphI-digested amplicon was inserted into the same sites in pIJ702E, yielding pAMF19.
Three AmfS expression vectors, pSPO10, pSPO100, and pSPO10SAV were constructed as follows. To generate pSPO10, the EcoRI and HindIII-digested DNA fragment containing the entire amf cluster derived from pSPO1 was inserted into the same site in pIJ702E2. To construct pSPO100, the amfT-amfS region was amplified by the PCR primer pair, amfTS-F/amfTS-R. After the digestion by SphI and PstI, the fragment was introduced into the same sites in pAMF19, giving rise to pSPO100. In order to generate pSPO10SAV, an expression vector of S. avermitilis amfS, cosmid clones, CL_238_H01, and CL_215_B03, containing the entire S. avermitilis amf gene cluster were partially digested by Sau3AI, and randomly cloned into the BglII site in pUC19BglII. The plasmid carrying the entire amf cluster was selected by PCR with the appropriate primer pairs. The EcoRI and HindIII-digested DNA fragment of pUC19BglII carrying the amf cluster was inserted into the same site in pIJ702E2 to give rise to pSPO10SAV.
Detection and bioassay of AmfS produced by liquid culture
S. grisues transformants were grown in shaking culture for 48 h in a 500-mL baffled flask containing 100-mL YMP-1% glucose medium. After centrifugation at 3700 × g for 10 min, 2 × volume of 99.5% ethanol was added to the 5-mL filtrated culture supernatant, and incubated at −80 °C for 2 h. After centrifugation at 6600 × g for 10 min, the resultant precipitate was dissolved in 50–100 μL of 10 mM Tris–HCl (pH 7.2) buffer or dimethyl sulfoxide (DMSO). To examine morphogen activity, 10 μL of the solution was directly spotted onto the amfS mutant inoculated on YMP-glucose solid medium to form a confluent lawn. The remaining sample (10–20 μL) was subjected to 15% sodium dodecyl sulfate polyacrylamide gel electrophoresis (SDS-PAGE) with 1 × SDS Tris–tricine buffer (NACALAI TESQUE, INC. Kyoto, Japan).Citation36) About 10 μL of a low-range rainbow molecular weight marker (GE Healthcare UK Ltd., Buckinghamshire, England) was used as a visible marker. Protein was detected by silver staining. The band intensity was measured by Image J (https://imagej.nih.gov/ij/), and the yield of the produced AmfS was estimated based on the intensity of SDS-PAGE molecular weight marker. The molecular weight of AmfS was measured using a MALDI-TOF MS Voyager-DE Pro (Thermo Fisher Scientific Inc., Waltham, MA, USA) according to the manufacturer’s instruction. The N-terminal sequence was analyzed with Protein Sequencer model 492 (Thermo Fisher Scientific).
Detection and bioassay of AmfS produced by solid culture
S. griseus was grown on a cellophane seat-placed minimal medium or YMP-1% glucose solid medium at 28 °C for 4 days. The 200–500 mg mycelium were scraped off the surface of the plates using a bent spatula, and suspended in 1 mL of 10 mM Tris–HCl (pH 7.2) containing 2 M LiCl. After vigorous vortexing, the samples were incubated on ice for 2 h. After centrifugation at 21,500 × g for 5 min, the recovered supernatant was filtered through a 0.45-μm pore filter (Merck Millipore Corp., Darmstadt, Germany) to remove cells. A 2 × volume of 99.5% ethanol was added, and the samples were incubated at −80 °C for 1 h. After centrifugation at 21,500 × g for 10 min, the precipitate was dissolved in 50–100 μL of 10 mM Tris–HCl (pH7.2) buffer or DMSO. To examine morphogen activity, 10 μL of the solution was directly spotted onto the ΔamfS spread on YMP-glucose solid medium.
Production, isolation, and analysis of AmfS peptides
The S. griseus transformants were grown in a shaking culture for 48 h in a 500-mL baffled flask containing 100-mL YMP-1% glucose medium with appropriate antibiotics. A total 3 L of culture was required to purify AmfS to near-homogeneity. After centrifugation at 3700 × g for 10 min, a 2 × volume of 99.5% ethanol was added to the filtrated culture supernatant, and the samples were incubated at −80 °C overnight. After centrifugation at 6600 × g for 30 min, the precipitate was suspended in 10-mM Tris–HCl (pH 7.2) buffer. The sample was applied to an octadecyl silane (ODS) reverse phase column chromatography (Senshu Scientific Co., Tokyo, Japan). The morphogen activity was mainly included in the eluted fraction with 40% acetonitrile (CH3CN) in H2O. The fraction was then applied to RESOURCE RPC 3 mL (GE Healthcare), and the purified activity principle was obtained by the elution with a linear gradient of CH3CN (0–50%) in H2O. Molecular mass was confirmed by MALDI-TOF MS.
Gene disruption of S. avermitilis amfS
The gene disruption of the amf gene of S. avermitilis (designated as amfSsav) was carried out as described in a previous report.Citation23) To construct a disruption vector for amfS, the upstream and downstream regions of the gene were amplified by PCR using the primer set DisamfS-F/DisamfS-MR and DisamfS-MF/DisamfS-R (Supplementary Table S1), respectively. Both PCR products were digested by BglII, and then ligated. The fused DNA fragment consisting of the upstream and downstream regions was selectively amplified using the PCR primer set DisamfS-F/DisamfS-R. The amplified DNA fragment digested by HindIII was introduced into the same site in pKU250, yielding pKU250updwn. A kanamycin resistance gene, aphII, flanked with loxP sites was amplified using Km-F and Km-R primer pair. The BamHI-digested aphII was inserted into the BglII site of pKU250updwn, which is located in the central site between the upstream and downstream regions of amfS, yielding the disruption vector pKU250ΔamfS. The sequence of the disruption vector was verified by sequencing using an ABI3730 Genetic Analyzer (Thermo Fisher Scientific) or by Eurofins Genomics K.K. The pKU250ΔamfS was introduced into S. avermitlis by conjugation with E. coli BL21 retaining pUB307 as a helper plasmid as described previously.Citation23) The expected double crossover-mediated homologous recombination was verified by PCR using the appropriate primer sets.
Electron microscopy
Cell morphology of S. avermitilis was assessed using a VE8800 scanning electron microscope (Keyence, Tokyo, Japan). Cells were grown at 30 °C for 4 days on YMS medium. To prepare the specimens, agar blocks were fixed with 2% osmium tetroxide for 30 h and then dehydrated by freeze-drying. Each specimen was sputter-coated with palladium/gold using an E-1010 ion sputter (Hitachi, Tokyo, Japan).
In vivo mutagenesis of AmfS
A Streptomyces chromosome integrative vector pNU10 was constructed by removing the GFP gene and terminator regions from pIJ8660. The DNA fragment containing pMB1 ori, aac3(IV) and attP was excised as an EcoRI/SphI-digested fragment from pIJ8660 and ligated with a phiC31 integrase gene cassette amplified by PCR using phiC31-F/phiC31-R primer set. Thus, formed chimeric plasmid was inserted with a multiple-cloning site cassette prepared from pIJ8660 by PCR using MCS-F/MCS-R primers at its EcoRI site to yield pNU10. The amfT coding sequence and its promoter region were amplified by PCR using the primer pair amfT-F/amfT-R. The resulting amplicon was digested by EcoRV and XbaI, and inserted into the same site in pNU10, giving rise to pNU10-amfT. The DNA fragments containing the wild type and mutated amfS constructs described below were inserted into XbaI-KpnI sites of pNU10-amfT to generate a series of the mutated amfS retaining vectors. The nucleotide sequences were verified using an ABI3730 sequencer or sequenced by Eurofins Genomics.
Nucleotide substitution of amfS was carried out with Mutan-Super Express Km (Takara Bio) using the oligonucleotides listed in Supplementary Table S1. To generate a pKF18k vector carrying the wild-type amfS cassette, the DNA fragment retaining amfS was amplified using amfS-F/amfS-R primers, and the amplicon was cloned into the XbaI-KpnI sites in the pKF18k vector. The resultant plasmid was subjected to nucleotide substitutions according to the manufacturer’s instruction. The N-terminally 6-His-tagged amfS (His6-AmfS), with a structure of T-His6-GSQVSLLVCEYSSLSVVLCTP was constructed by overlap PCR. The upstream and downstream regions of T22 of amfS were amplified with amfS-F/pTS-M-M and pTS-M-MF/amfS-R primer pairs, respectively. These amplicons were fused by a second PCR using the amfS-F/amfS-R primer pair. The resultant amplicon was cloned into the XbaI-KpnI sites in pNU10-amfT to yield His6-AmfS. For the construction of a C-terminally 6-His-fused AmfS-His6 (having a structure of TGSQVSLLVCEYSSLSVVLCTP-His6), overlap PCR was performed with amfS-F/pTS-C-MR and pTS-C-MF/amfS-R primer pairs, respectively. The overlap PCR product was inserted into the XbaI and KpnI sites in pNU10-amfT to yield AmfS-His6. The nucleotide sequences were verified using an ABI3730 sequencer or sequenced by Eurofins Genomics. The series of pNU10-amfT retaining the mutated and tag-fused amfS recovered from the methylation-free E. coli strain GM2163 were introduced into the ΔamfS of S. griseus using PEG-protoplast transformation methods. The transformants were selected by the overlay of nutrient broth soft agar containing apramycin. The aerial mycelium formation of transformants was observed after 4 days cultivation on YMP-glucose agar medium.
Results
Wide distribution of class III lantipeptides biosynthesis gene cluster in actinomycetes
Accumulating genetic information shows the wide distribution of a putative biosynthetic gene clusters for class III lantipeptides in actinomycetes. Among gene clusters encoding class III lantipeptides, the amf cluster consisting of amfT, amfS, amfB, and amfA is highly conserved in the genome of Streptomyces and related bacteria (Fig. (A) and Supplementary Table S2). In contrast, the amfR homolog is semi-conserved, and no protease to remove the leader peptide could be found within the gene cluster or in the neighboring region except for that in Streptosporangium roseum and Kribbella flavida.
AmfS and its homologs in Streptomyces and related bacteria have conserved amino acid residues in both N- and C-terminal regions (Fig. (B) and Supplementary Table S2). The N-terminal region of AmfS is negatively charged and retains a conserved Leu-Leu-Asp-Leu-Gln motif. The C-terminal core peptide region is highly hydrophobic, and retains conserved Ser-(Xxx)2-Ser-(Xxx)3-Cys motifs (Xxx, any amino acid). As shown in Supplementary Table S2, both N- and C-terminal motifs are highly conserved among class III lantipeptides. On the other hand, the amino acid sequence around the processing site located in the central region of precursor peptides is relatively divergent between species. Fig. (C) shows the predicted structures of AmfS of S. griseus and S. avermitilis based on that of SapB of S. coelicolor A3(2).Citation37,38)
The high conservation of class III lantipeptide gene cluster suggests that bacteria belonging to actinomycetes retain general capability of producing class III lantipeptides. Thus, genetically engineered actinomycetes may be a suitable host for achieving high-level production of lantipeptides. We selected S. griseus as an expression host for class III lantipeptides because the host-vector system has been established. In addition, the molecular mechanism of morphological differentiation and secondary metabolite production has been well studied, as represented by knowledge regarding the A-factor regulatory cascade.Citation39)
Isolation of a S. griseus mutant suitable for overproduction of AmfS
Morphological differentiation and secondary metabolites production by Streptomyces are largely affected by culture conditions, especially the concentration of catabolite as a carbon source.Citation40) The catabolite-driven inhibition of aerial mycelium formation and secondary metabolite production is a phenomenon known as a carbon catabolite repression. To examine which types of sugar affect the productivity of AmfS, we carried out a morphogen assay using AmfS partially purified from the S. griseus wild-type strain grown on a minimal solid medium. An extracellular protein fraction recovered from the S. griseus cultured on the minimal medium containing glucose had no activity to restore the aerial mycelium formation of the amfS mutant (ΔamfS) (Supplementary Fig. S1). In contrast, an extracellular fraction recovered from the S. griseus grown on minimal medium-containing galactose, maltose, and mannitol had a morphogen activity to ΔamfS (Supplementary Fig. S1). We confirmed that the morphogen activity was not observed when ΔamfS was used as a donor strain (data not shown). This result indicates that AmfS production by S. griseus undergoes glucose repression.
To construct a host suitable for production of class III lantipeptides in S. griseus, we screened for a mutant strain relieved from glucose repression. A glucose repression-lacking strain was selected from UV-irradiated S. griseus mutant libraries, since the detailed molecular mechanism underlying the glucose repression of aerial mycelium formation remains unclear in this genus. Of 5000 colonies, 20 mutants were able to form spores on YMP-10% glucose solid medium. Among these, a mutant designated as Grd1 reproducibly formed abundant aerial mycelium on YMP-10% glucose medium (Fig. ). Therefore, the Grd1 mutant was chosen as the host for the following studies.
Construction of expression vectors for AmfS
To obtain high production levels of AmfS in S. griseus, an expression vector pIJ702E2 (Fig. (A)) was constructed. This vector was based on a multi-copy number plasmid pIJ702, which has been used for general cloning and protein expression in Streptomyces.Citation31) pIJ702E2 contains two convergent ermEp2, a strong and constitutive promoter derived from the erythromycin biosynthesis gene cluster of S. erythraea. The S. griseus amfTSBAR cluster from pSPO1 was inserted into the EcoRI and HindIII sites between the two convergent ermEp2 flanking to aphII of pIJ702E2, yielding pSPO10 (Fig. (A)). In this expression vector, the transcription of both amfR and amfTSBA operon was expected to be directed by the ermEp2 as well as their native promoters. pSPO10 is expected to direct the constitutive expression of AmfR, which activates the transcription of the amfTSBA operon. We also constructed a pSPO100 vector (Fig. (A)), which contains a single ermEp2 and amfR, but lacks the putative AmfS exporters genes, amfA and amfB. The fragment containing amfT and amfS was inserted between the PstI and SphI sites of pAMF19, which was constructed by cloning ermEp2 and amfR onto pIJ702E2 (Fig. (B)) (see also Materials and methods). On pSPO100, the transcription of amfT-amfS is expected to be directed by two promoters; (1) the ermEp2 preceding amfR, via read-through transcription, and (2) the amfT promoter activated by the constitutively expressed AmfR.
Fig. 3. Physical structures of the expression vectors pIJ702E2 and pAMF19.
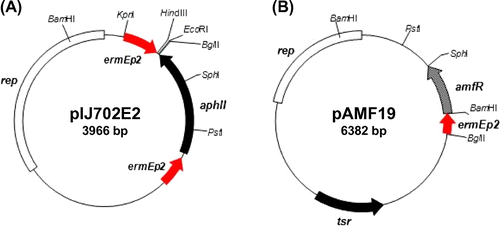
Fig. 4. Schematic representation of AmfS expression vectors and the expression analyses of AmfS in S. griseus by SDS-PAGE.
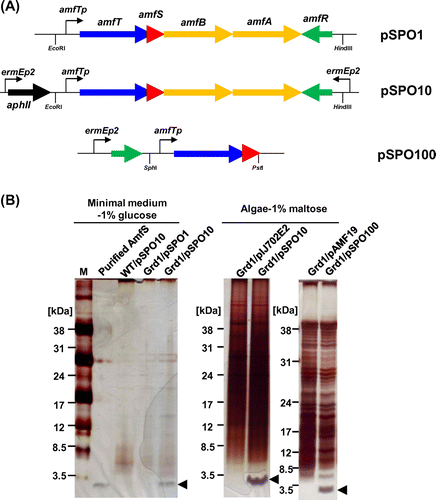
Production of S. griseus AmfS in Grd1 strain
The vectors, pSPO1, pSPO10, and pSPO100, were introduced into S. griseus wild-type and the Grd1 mutant. These transformants were cultured, and the proteins secreted into the supernatant were concentrated by ethanol precipitation. The total extracellular proteins were separated by SDS-PAGE and detected by silver staining (see Materials and methods). An approximately 2-kDa protein band corresponding to the predicted mature AmfS was detected when Grd1 strain harboring pSPO10 was cultured in the minimal medium containing 1% glucose (Fig. (B)). In contrast, the corresponding band was not detected for the wild-type S. griseus carrying pSPO10 and Grd1 carrying pSPO1 (Fig. (B)). In a medium containing algal extract, Grd1 harboring pSPO10 produced a large amount of AmfS into the culture broth, while Grd1 carrying empty vector pIJ702E2 did not exhibit high production (Fig. (B)). The N-terminal amino acid sequence of the isolated AmfS was Thr-Gly-Xxx-Gln (Xxx indicates an unidentified residue), corresponding to 22–25 amino acid residues of the precursor (Fig. (B)). MALDI-TOF analysis revealed that the molecular mass of the overproduced AmfS was 2214 Da, 72 Da smaller than that of the calculated molecular mass of the corresponding C-terminal region (2286 Da). This indicates that the mature AmfS forms the SapB-like ring structure due to the dehydration at the four Ser residues (Fig. (C)). These results clearly indicate that: (1) S. griseus Grd1 strain is a suitable host for high production of AmfS and (2) pIJ702E2 works as an efficient expression vector for AmfS.
We also studied whether pSPO100 serves as an efficient expression vector for AmfS (Fig. (A)). S. griseus Grd1 carrying pSPO100 secreted a large amount of AmfS into the culture broth. In contrast, S. griseus Grd1 carrying an empty vector pAMF19 vector did not show overproduction (Fig. (B)). This result indicates that the overexpression of AmfA and AmfB, putative exporters of AmfS, is not required for overproduction of AmfS into culture medium. The pSPO100 vector (total length = 9185 bp) is smaller than the pSPO10 (total length = 11,310 bp). Thus, we expect that pSPO100 will be more useful since the smaller size makes possible easy handling of the plasmid in terms of its genetic stability in the host strain.
Production of S. avermitilis AmfSsav in Grd1 strain
AmfSsav of S. avermitilis, a producer of the anthelmintic agent avermectin, has conserved N- and C-terminal regions (Fig. (B) and Supplementary Table S2) similar to those of AmfS. In contrast, the central processing region of AmfSsav and its length are different from those of AmfS. This suggests that AmfSsav is an appropriate model to evaluate the versatility and functionality of this expression system. Prior to the evaluation of AmfSsav production, we constructed a null mutant for amfSsav (ΔamfSsav) to clarify its role in aerial mycelium formation in S. avermitilis. The constructed ΔamfSsav was defective in the formation of aerial mycelium (bald phenotype) on YMS medium (Fig. (A)). The bald phenotype of ΔamfSsav was restored to the wild-type phenotype by the exogenous addition of an AmfSsav-containing fraction recovered from the cell surface of wild-type S. avermilitis (Fig. (A)). Hence, the bald phenotype conferred by the amfSsav mutation is due to the inability to produce AmfSsav. This indicates that AmfSsav also serves as a morphogen.
Fig. 5. Morphology of S. avermitilis ΔamfSsav and production of S. avermitilis AmfSsav in the S. griseus Grd1 strain.
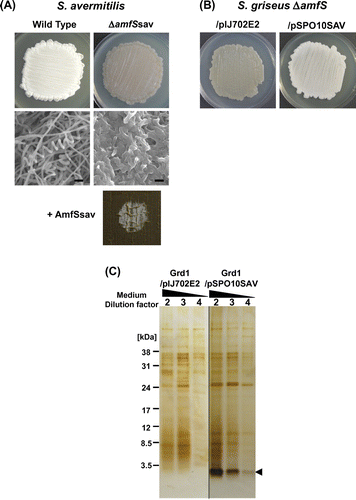
The AmfSsav expression vector, pSPO10SAV, was constructed by the insertion of the amf cluster from S. avermitilis into pIJ702E2 (see Materials and methods). The introduction of pSPO10SAV restored aerial mycelium formation in S. griseus ΔamfS (Fig. (B)). This indicates that AmfSsav serves as an active morphogen even in a heterologous host, S. griseus. We also discovered that AmfSsav partially purified from the S. griseus transformant restored aerial mycelium formation when it was exogenously supplied onto the colony of both ΔamfS of S. avermitilis and S. griseus (data not shown). This verified that AmfSsav is also produced into the culture broth in the S. griseus.
To examine AmfSsav productivity in the S. griseus expression system, the extracellular proteins of the Grd1 strain harboring pSPO10SAV were separated by SDS-PAGE and detected by silver staining. As shown in Fig. (C), AmfSsav production was not observed in the Grd1 carrying the empty vector, pIJ702E2. Meanwhile, a remarkable 2- kDa protein band probably corresponding to AmfSsav was detected from the Grd1 carrying pSPO10SAV. We examined various medium conditions and discovered that use of Bennett’s-maltose medium diluted for 2–4-fold was effective (Fig. (C)).
To confirm that the expressed protein was indeed AmfSsav, we purified AmfSsav from Grd1 harboring pSPO10SAV using several rounds of column chromatography (see Materials and methods). The N-terminal amino acid sequence of the purified AmfSsav was Thr-Gly-Gly-Gly-Gly-Ala, which perfectly matched the 15–20 amino acid residues of its precursor (Fig. (B)). The calculated molecular weight of the corresponding C-terminal region was 2167 Da. On the other hand, the mass measured by MALDI-TOF MS was 2095 Da, 72 Da smaller than the calculated weight. This supported the view that AmfSsav also forms the SapB-type ring structure (Fig. (C)) due to the dehydration at the four conserved Ser residues. These results indicate that: (1) the highly produced small peptide was AmfSsav, and (2) this expression system can be applied for high-level production of AmfS derived from other Streptomyces.
Identification of amino acid residues essential for AmfS activity
The identification of amino acid residues affecting AmfS morphogen activity provides important information regarding the correlation between structure and activity. Therefore, we constructed various substitution mutant cassettes and introduced them into the ΔamfS to observe the effect on aerial mycelium formation. The mutated amfS was introduced into the chromosome of ΔamfS via a chromosome integrative vector, pNU10, to avoid the gene dosage effect. The aerial mycelium formation of ΔamfS was partially restored by the introduction of pNU10 carrying amfS alone. However, the co-introduction of amfS and amfT on pNU10 completely restored the phenotype of ΔamfS to a wild-type level (corresponding to the wild-type shown in Fig. ). Therefore, we co-introduced the series of mutated amfS with amfT (see Materials and methods).
Fig. 6. Genetic and extracellular-complementation tests of S. griseus ΔamfS.
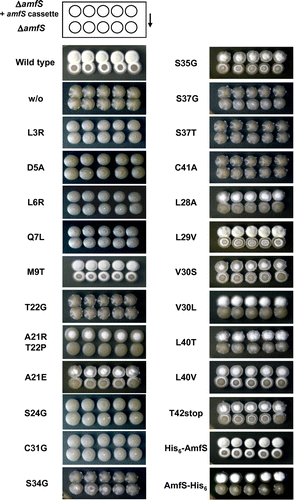
The leader peptide of the Streptomyces class III lantipeptide precursor is negatively charged, and has a highly conserved Leu-Leu-Asp-Leu-Gln motif (Fig. (B) and Supplementary Table S2), which is essential for modifying enzymatic processes. As shown in Fig. , the ΔamfS carrying the substitution of L3R, D5A, L6R, and Q7L was unable to form aerial mycelium and to restore aerial mycelium in ΔamfS, indicating that these conserved residues are essential for the biosynthesis and excretion of the active morphogenic peptide. In contrast, the M9T mutant was able to restore the aerial mycelium formation of its own and adjacent colonies. This indicates that M9 is not essential for biosynthesis or morphogen activity.
We next analyzed protease cutting sites required for AmfS maturation. The amino acid residues around the processing region are not conserved among class III lantipeptides of Streptomyces (Fig. (B) and Supplementary Table S2). As mentioned above, the N-terminal sequencing indicated that the processing site of S. griseus AmfS is located between A21 and T22. The T22G mutant was unable to induce aerial mycelium (Fig. ). On the other hand, the mutations, A21R, T22P, and A21E, had partial restoration activity for the own aerial mycelium formation, but they did not restore aerial mycelium formation of the adjacent colonies of ΔamfS (Fig. ). These results indicate that the amino acid residues at the processing site are required for correct excretion of AmfS.
The core peptide of the Streptomyces class III lantipeptide is highly hydrophobic, and has two consensus motifs Ser-(Xxx)2-Ser-(Xxx)2–5-Cys (Fig. (B) and Supplementary Table S2). The conserved Ser and Cys predicted to be the sites of modification hence play an important role for the structure of AmfS. To verify the significance of these amino acids, we constructed the mutants, S24G, C31G, S34G, S37G, S37T, and C41A in the B-ring. All of these mutations resulted in complete loss of aerial mycelium formation (Fig. ), indicating that the conserved amino acid residues in the core peptides are essential for AmfS activity and/or its biosynthetic process. In contrast, S35G in the B-ring restored aerial mycelium formation. This indicates that this residue is not involved in its morphogen activity. This is supported by the fact that S35 is a non-conserved amino acid residue. We also analyzed the mutations in non-conserved amino acid residues within A-ring (L28A, L29V, V30S, V30L), B-ring (L40T, L40V), and in tailored region (T42stop). These mutants were able to restore their own aerial mycelium formation, but some of them were unable to restore aerial mycelium in adjacent colonies (Fig. ). Interestingly, L29V led to abundant aerial mycelium formation compared to the wild type, indicating that the valine at this position confers more effective morphogen activity to the peptide.
We also constructed an N-terminally 6-His-fused AmfS (His6-AmfS) to examine whether the presence of the tag affects morphogen activity. The ΔamfS retaining His6-AmfS formed aerial mycelium, and restored aerial mycelium of the adjacent ΔamfS (Fig. ). This result indicates that an N-terminal fusion tag does not affect the biosynthesis and activity of AmfS. On the other hand, the C-terminally 6-His–fused AmfS (AmfS-His6) was able to restore own aerial mycelium formation of ΔamfS, but not in the adjacent colonies (Fig. ).
Discussion
The study of lantipetides produced by Streptomyces has mainly been confined to the study of morphogen peptides; however, the increased availability of genomic information has opened the potential ability to produce diverse lantipeptides. Among the four classes of lantipetides, class III lantipeptides have been documented to have low productivity, and are thus often overlooked. Herein, we constructed a high production system of AmfS, a class III lantipeptide. The production system consists of the S. griseus host, Grd1 strain, and expression vectors. Our genetic study also identified the amino acid residues essential for AmfS morphogen activity. We believe that this expression system will become a useful tool for the discovery of a class III lantipeptide with a novel biological function.
The Grd1 strain, a mutant deficient in glucose repression was obtained from S. griseus UV mutated libraries. This mutant is capable of producing abundant aerial mycelium even when cultured under high glucose conditions (Fig. ). In S. griseus, the molecular mechanism for the initiation of development is well studied, as known by the A-factor regulatory cascade. However, the mechanism for glucose repression is not fully understood; a central gene involved in glucose repression has not been identified in Streptomyces strains.Citation40) Our future work will identify the mutation point(s) in the Grd1 strain in order to identify the molecular mechanism responsible for glucose repression in this genus.
Two expression vectors were constructed in this study. The unique feature of pIJ702E2 is the retention of two convergent ermEp2. In general, two identical promoters on a single high copy vector tend to cause instability, but pIJ702E2 was relatively stable under the culture conditions used in this study. We believe that the successful overproduction of AmfS is largely due to the following: (1) the use of two emrE2 promoters on a single plasmid, and (2) the strong promoter activity of amfT. With respect to the latter, our S1 nuclease mapping verified that amfT promoter exhibits robust transcription activity compared to other promoters, such as the hrdB promoter (our unpublished data). This indicates that AmfR serves as a strong activator for the amfT promoter. Therefore, we assume that constitutive overexpression of AmfR is another important factor driving high production of AmfS.
S. griseus wild-type harboring pSPO10 was unable to overproduce AmfS, while Grd1 strain harboring pSPO10 overproduced AmfS (Fig. (B)). The fact suggests that the activity of amfTp is low in the wild type, but elevated in the Grd1 mutant. Probably, ermEp2, the heterogeneous promoter, is active in both strains and expresses AmfR to the same level. This raises the possibility that amfTp is under the control of not only AmfR but also catabolite repression hence upregulated in the genetic background of Grd1 relieving the formation of aerial mycelium from glucose repression. This could be the reason why Grd1 mutant is suitable for the overexpression of AmfS.
The Grd1 transformant retaining pSPO100 vector produced a large amount of AmfS despite the absence of the ABC transporters genes amfA and amfB on the plasmid. AmfA and AmfB are thought to drive transport of AmfS to the outside of the cells. Our previous study showed that the mutant lacking both amfA and amfB was capable of normally forming aerial mycelium, but lacked the activity to secrete AmfS, indicating that AmfA and AmfB are essential for extracellular AmfS activity. The effective AmfS secretion by Grd1 harboring pSPO100 suggests that the chromosomally encoded AmfA and AmfB are highly capable of AmfS export.
The S. griseus expression system constructed in this study was also able to drive overproduction of AmfSsav derived from S. avermitilis. AmfSsav differs from AmfS in that: (1) the AmfSsav amino acid residues around the processing sites are largely different from those of S. griseus AmfS, and (2) the length of the precursor of AmfSsav (38 amino acids) is shorter than that of AmfS (43 amino acids). The successful overproduction of AmfSsav indicates that the S. griseus system provides versatility for generation of diverse lantipeptides. This result also indicates that the expression of lantipeptide homologous to AmfS will be successful even without the introduction of any protease for the generation of mature peptides. This is supported by the absence of coding sequence for protease in the flanking region of amf cluster. As reported by a previous study, it is assumed that unidentified aminopeptidase-like proteases may be involved in the maturation of lantipeptides since the detected peptides have different N-terminal sequences.Citation29) However, we should be careful about the possibility that co-expression of protease gene with lantipepetide biosynthesis genes is required for the expression of other types of lantipeptides.
Our mutagenesis study on AmfS enabled identification of the amino acid residues involved in its morphogen activity. The result suggested that the amino acid residues conserved in the class of AmfS/SapB lantipeptide family are required for the expression of the morphogen activity. The amino acid replacement changes the structure of the peptide, but may also affect the transcriptional level of amfSBA and the substrate recognition by modifying enzyme and transporter. Interestingly, the L29V mutation caused abundant aerial mycelium formation compared to the wild type. This infers that the amino acid residue at this position within the A-ring structure is critical for the morphogen activity and that valine at this position make the peptide more active in the induction of aerial hyphae structure. The information may help understanding the mechanism of aerial hyphae formation based on the association with the hydrophobic peptide.
We also showed that the addition of a His-tag to the N-terminus of the mature peptide does not affect AmfS activity. The use of the tag facilitates purification and further biochemical analysis of the AmfS peptide.
We expect that the constructed expression system will be used to further explore the biological function of lantipeptides, including: (1) their morphogen activity and (2) antibacterial activity. The use of the ΔamfS as a host facilitates evaluation of whether the expressed lantipeptides have morphogen activity. Further improvement of the vectors for an inducible expression system will enable assessment of whether the expressed lantipeptides have antibacterial activity based on growth inhibition of the host. The combination of genomic information with the expression system presented in this study will promote the discovery of lantipeptides with structures and biological activities that are not yet known.
Author contributions
Hideaki Takano and Kenji Ueda are responsible for project planning. H. Takano designed the study and wrote the paper. H. Takano, Yuhei Matsui, Junpei Nomura, Masahiro Fujimoto, Naoto Katsumata, Takafumi Koyama, Isamu Mizuno, Shoichi Amano, Hatsumi Shiratori-Takano performed the experiments. Mamoru Komatsu and Haruo Ikeda supported H. Takano in performing gene disruption experiments in S. avermitilis. H. Takano and K. Ueda analyzed the experiments. All authors reviewed the results and approved the final version of the manuscript.
Disclosure statement
No potential conflict of interest was reported by the authors.
Funding
This study was supported by the grant-in-aid for scientific research from the Ministry of Education, Culture, Sports, Science and Technology, Japan [Ref. 21580103], [Ref. 24580128] to K. Ueda; and a grant from the Institute of Fermentation, Osaka, Japan, and the Foundation NAGASE Science Technology Development, Osaka, Japan to K. Ueda.
Supplemental material
The supplemental material for this paper is available at http://dx.doi.org/10.1080/09168451.2016.1238297.
TBBB_1238297_Supplementary_Table_2.docx
Download MS Word (28.4 KB)TBBB_1238297_Supplementary_Table_1.docx
Download MS Word (18.3 KB)TBBB_1238297_Supplementary_Figure.pptx
Download MS Power Point (260 KB)References
- Katz L, Baltz RH. Natural product discovery: past, present, and future. J. Ind. Microbiol. Biotechnol. 2016;43:155–176.10.1007/s10295-015-1723-5
- Barka EA, Vatsa P, Sanchez L, et al. Taxonomy, physiology, and natural products of Actinobacteria. Microbiol. Mol. Biol. Rev. 2016;80:1–43.10.1128/MMBR.00019-15
- Flärdh K, Buttner MJ. Streptomyces morphogenetics: dissecting differentiation in a filamentous bacterium. Nat. Rev. Microbiol. 2009;7:36–49.10.1038/nrmicro1968
- Ikeda H, Shin-ya K, Omura S. Genome mining of the Streptomyces avermitilis genome and development of genome-minimized hosts for heterologous expression of biosynthetic gene clusters. J. Ind. Microbiol. Biotechnol. 2014;41:233–250.10.1007/s10295-013-1327-x
- Ikeda H, Ishikawa J, Hanamoto A, et al. Complete genome sequence and comparative analysis of the industrial microorganism Streptomyces avermitilis. Nat. Biotechnol. 2003;21:526–531.10.1038/nbt820
- Arnison PG, Bibb MJ, Bierbaum G, et al. Ribosomally synthesized and post-translationally modified peptide natural products: overview and recommendations for a universal nomenclature. Nat. Prod. Rep. 2013;30:108–160.10.1039/C2NP20085F
- Lubelski J, Rink R, Khusainov R, et al. Biosynthesis, immunity, regulation, mode of action and engineering of the model lantibiotic nisin. Cell. Mol. Life Sci. 2008;65:455–476.10.1007/s00018-007-7171-2
- Willey JM, van der Donk WA. Lantibiotics: peptides of diverse structure and function. Annu. Rev. Microbiol. 2007;61:477–501.10.1146/annurev.micro.61.080706.093501
- Willey JM, Gaskell AA. Morphogenetic signaling molecules of the streptomycetes. Chem. Rev. 2011;111:174–187.10.1021/cr1000404
- Iorio M, Sasso O, Maffioli SI, et al. A glycosylated, labionin-containing lanthipeptide with marked antinociceptive activity. ACS Chem. Biol. 2014;9:398–404.10.1021/cb400692w
- Férir G, Petrova MI, Andrei G, et al. The lantibiotic peptide labyrinthopeptin A1 demonstrates broad anti-HIV and anti-HSV activity with potential for microbicidal applications. PLoS ONE. 2013;8:e64010.10.1371/journal.pone.0064010
- Kodani S, Lodato MA, Durrant MC, et al. SapT, a lanthionine-containing peptide involved in aerial hyphae formation in the streptomycetes. Mol. Microbiol. 2005;58:1368–1380.10.1111/j.1365-2958.2005.04921.x
- Ueda K, Takano H, Nishimoto M, et al. Dual transcriptional control of amfTSBA, which regulates the onset of cellular differentiation in Streptomyces griseus. J. Bacteriol. 2005;187:135–142.10.1128/JB.187.1.135-142.2005
- Ueda K, Oinuma K, Ikeda G, et al. AmfS, an extracellular peptidic morphogen in Streptomyces griseus. J. Bacteriol. 2002;184:1488–1492.10.1128/JB.184.5.1488-1492.2002
- Keijser BJ, van Wezel GP, Canters GW, et al. Developmental regulation of the Streptomyces lividans ram genes: involvement of RamR in regulation of the ramCSAB operon. J. Bacteriol. 2002;184:4420–4429.10.1128/JB.184.16.4420-4429.2002
- Nguyen KT, Willey JM, Nguyen LD, et al. A central regulator of morphological differentiation in the multicellular bacterium Streptomyces coelicolor. Mol. Microbiol. 2002;46:1223–1238.10.1046/j.1365-2958.2002.03255.x
- Bentley SD, Chater KF, Cerdeño-Tárraga AM, et al. Complete genome sequence of the model actinomycete Streptomyces coelicolor A3(2). Nature. 2002;417:141–147.10.1038/417141a
- Yamazaki H, Takano Y, Ohnishi Y, et al. amfR, an essential gene for aerial mycelium formation, is a member of the AdpA regulon in the A-factor regulatory cascade in Streptomyces griseus. Mol. Microbiol. 2003;50:1173–1187.10.1046/j.1365-2958.2003.03760.x
- Ochi K, Hosaka T. New strategies for drug discovery: activation of silent or weakly expressed microbial gene clusters. Appl. Microbiol. Biotechnol. 2013;97:87–98.10.1007/s00253-012-4551-9
- Amano SI, Sakurai T, Endo K, et al. A cryptic antibiotic triggered by monensin. J. Antibiot. (Tokyo). 2011;64:703p.10.1038/ja.2011.69
- Yoon V, Nodwell JR. Activating secondary metabolism with stress and chemicals. J. Ind. Microbiol. Biotechnol. 2014;41:415–424.10.1007/s10295-013-1387-y
- Onaka H, Tabata H, Igarashi Y, et al. Goadsporin, a chemical substance which promotes secondary metabolism and morphogenesis in streptomycetes. I. Purification and characterization. J. Antibiot. (Tokyo). 2001;54:1036–1044.10.7164/antibiotics.54.1036
- Komatsu M, Uchiyama T, Omura S, et al. Genome-minimized Streptomyces host for the heterologous expression of secondary metabolism. Proc. Natl. Acad. Sci. U S A. 2010;107:2646–2651.10.1073/pnas.0914833107
- Gomez-Escribano JP, Bibb MJ. Heterologous expression of natural product biosynthetic gene clusters in Streptomyces coelicolor: from genome mining to manipulation of biosynthetic pathways. J. Ind. Microbiol. Biotechnol. 2014;41:425–431.10.1007/s10295-013-1348-5
- Komatsu M, Komatsu K, Koiwai H, et al. Engineered streptomyces avermitilis host for heterologous expression of biosynthetic gene cluster for secondary metabolites. ACS Synth. Biol. 2013;2:384–396.10.1021/sb3001003
- Du D, Katsuyama Y, Onaka H, et al. Production of a novel amide-containing polyene by activating a cryptic biosynthetic gene cluster in Streptomyces sp. Chembiochem. 2016;17:1464–1471.
- Laureti L, Song L, Huang S, et al. Identification of a bioactive 51-membered macrolide complex by activation of a silent polyketide synthase in Streptomyces ambofaciens. Proc. Natl. Acad. Sci. U S A. 2011;108:6258–6263.10.1073/pnas.1019077108
- Onaka H, Mori Y, Igarashi Y, et al. Mycolic acid-containing bacteria induce natural-product biosynthesis in streptomyces species. Appl. Environ. Microbiol. 2011;77:400–406.10.1128/AEM.01337-10
- Völler GH, Krawczyk JM, Pesic A, et al. Characterization of new class III lantibiotics-erythreapeptin, avermipeptin and griseopeptin from Saccharopolyspora erythraea, Streptomyces avermitilis and Streptomyces griseus demonstrates stepwise N-terminal leader processing. Chembiochem. 2012;13:1174–1183.10.1002/cbic.v13.8
- Komatsu M, Tsuda M, Omura S, et al. Identification and functional analysis of genes controlling biosynthesis of 2-methylisoborneol. Proc. Natl. Acad. Sci. U S A. 2008;105:7422–7427.10.1073/pnas.0802312105
- Gusek TW, Kinsella JE. Review of the Streptomyces lividans/vector pIJ702 system for gene cloning. Crit. Rev. Microbiol. 1992;18:247–260.10.3109/10408419209113517
- Sun J, Kelemen GH, Fernández-Abalos JM, et al. Green fluorescent protein as a reporter for spatial and temporal gene expression in Streptomyces coelicolor A3(2). Microbiology. 1999;145(Pt 9):2221–2227.10.1099/00221287-145-9-2221
- Ueda K, Miyake K, Horinouchi S, et al. A gene cluster involved in aerial mycelium formation in Streptomyces griseus encodes proteins similar to the response regulators of two-component regulatory systems and membrane translocators. J. Bacteriol. 1993;175:2006–2016.
- Kieser T, Bibb MJ, Buttner MJ, et al. Practical Streptomyces genetics. Norwich: The John Innes Foundation; 2000.
- Maniatis T, Fritsch EF, Sambrook J. Molecular cloning: a laboratory manual. Cold Spring Harbor, NY: Cold Spring Harbor Laboratory Press; 1982.
- Schägger H, von Jagow G. Tricine-sodium dodecyl sulfate-polyacrylamide gel electrophoresis for the separation of proteins in the range from 1 to 100 kDa. Anal. Biochem. 1987;166:368–379.10.1016/0003-2697(87)90587-2
- Willey JM, Willems A, Kodani S, et al. Morphogenetic surfactants and their role in the formation of aerial hyphae in Streptomyces coelicolor. Mol. Microbiol. 2006;59:731–742.10.1111/mmi.2006.59.issue-3
- Kodani S, Hudson ME, Durrant MC, et al. The SapB morphogen is a lantibiotic-like peptide derived from the product of the developmental gene ramS in Streptomyces coelicolor. Proc. Natl. Acad. Sci. U S A. 2004;101:11448–11453.10.1073/pnas.0404220101
- Horinouchi S, Beppu T. Hormonal control by A-factor of morphological development and secondary metabolism in Streptomyces. Proc. Jpn. Acad. Ser. B Phys. Biol. Sci. 2007;83:277–295.10.2183/pjab.83.277
- Gubbens J, Janus M, Florea BI, et al. Identification of glucose kinase-dependent and -independent pathways for carbon control of primary metabolism, development and antibiotic production in Streptomyces coelicolor by quantitative proteomics. Mol. Microbiol. 2012;86:1490–1507.10.1111/mmi.2012.86.issue-6