Abstract
Strigolactones (SLs) and karrikins (KARs) regulate photomorphogenesis. GR24, a synthetic SL and KAR1, a KAR, inhibit the hypocotyl elongation of Arabidopsis thaliana in a weak light. GR24 and KAR1 up-regulate the expression of STH7, encoding a transcription factor belonging to the double B-box zinc finger subfamily. In this study, we used STH7-overexpressing (STH7ox) lines and functionally defective STH7 (STH7-SRDX) mutants to investigate roles of SLs and KARs in photomorphogenesis of Arabidopsis. Hypocotyl elongation of STH7-SRDX mutants was less sensitive to both GR24 and KAR1 treatment than that of wild-type Arabidopsis under weak light conditions. Furthermore, the chlorophyll and anthocyanin content was increased in STH7ox lines when de-etiolated with light and GR24-treated plants had enhanced anthocyanin production. GR24 and KAR1 treatment significantly increased the expression level of photosynthesis-related genes LHCB1 and rbcS. The results strongly suggest that SL and KAR induce photomorphogenesis of Arabidopsis in an STH7-dependent manner.
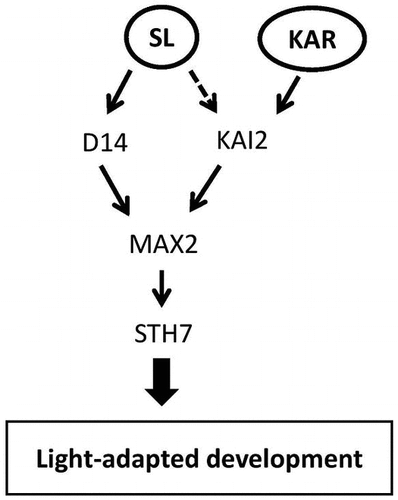
Strigolactone response is mediated by D14 and KAI2 while karrikin response is mediated by only KAI2.
Introduction
Light induces transitions from dark-grown (etiolated) to light-grown (de-etiolated) morphology.Citation1) In dicotyledonous plants, etiolated seedlings grown in the dark have elongated hypocotyls, closed apical hooks, unopened cotyledons, and undeveloped chloroplasts.Citation2,3) When plants are grown under light conditions, seedlings show light-adapted development characterized by a decrease of hypocotyl elongation, opened cotyledons, induction of true leaf expansion and biosynthesis of chlorophyll and anthocyanin.Citation1,2) This process is called de-etiolation or photomorphogenesis.Citation3–5) Light-adapted development during de-etiolation is regulated by several phytohormones such as gibberellins, auxins, ethylene, brassinosteroids, and strigolactones.Citation4,6–8) However, how plant hormone signal transduction pathways interact with light signals and activate the photomorphogenesis process is not fully understood.
Multiple genes regulate photomorphogenesis. The B-box zinc-finger protein or BBX protein family is one of the main downstream effectors of seedling photomorphogenesis. BBX proteins contain a box domain with one or two B-box motifs at the N-terminal, and some subfamilies have a CCT (CO-COL-TOC1) domain at the C-terminal.Citation9,10) The BBX protein family is composed of five main subfamilies divided by their protein sequences.Citation11) BBX subfamily IV has been reported as the major regulator of photomorphogenesis and light signaling. Subfamily IV B-box proteins consist of eight members including BBX18–BBX25. This subfamily carries only B-box B1 and B2 without a CCT domain.Citation10) STH7/BBX20 has been described as a positive regulator of photomorphogenesis and a negative regulator of brassinosteroid function.Citation4) Genome-wide analysis of gene expression revealed that the transcriptional expression of STH7 was up-regulated by strigolactone (SL)Citation12) and karrikin (KAR).Citation13)
SLs are terpenoidal lactone-type plant hormones that are classified as seed germination stimulants of root-parasitic weeds of the Orobanchaceae.Citation14) SLs are involved in the regulation of various phenomena such as suppression of shoot branching, regulation of root morphology, control of secondary growth, and so on.Citation14–18) KARs are a class of seed germination stimulant containing a methyl-butenolide moiety that is a common structure in SLs.Citation13,14) Both SLs and KARs can promote seed germination of Arabidopsis and inhibit hypocotyl elongation in the light which is one of the photomorphogenetic processes.Citation13,19–21) As SL and KAR can enhance STH7 expression level and STH7 is reported as a positive regulator of photomorphogenesis,Citation4) STH7 could be a possible candidate protein that functions in linking SL and KAR to induce light-adapted development in planta.
Here, we employed STH7-overexpressing (STH7ox) lines and functionally defective STH7 (STH7-SRDX) mutants to investigate roles of SLs and KARs in photomorphogenesis of Arabidopsis, and found that SL- and KAR-induced photomorphogenesis of Arabidopsis depends on STH7.
Material and methods
Plant materials and growth conditions
Arabidopsis thaliana ecotype Columbia (Col) or Landsberg erecta (Ler) were used as the wild-type plants. Arabidopsis SL-related mutants including max2-1 (a SL signaling mutant), max3-1 (a SL biosynthesis mutant), and d14-1 (a SL receptor mutant) as well as kai2 which is a KAR receptor mutant were used to investigate the effects of SL or KAR on hypocotyl elongation. Seeds were surface-sterilized by 70 and 99% ethanol for 30 min and 30 s, respectively. After that the sterilized seeds were germinated on 1/2 Murashige and Skoog (MS) medium containing 3% sucrose and 0.8% phyto agar (Duchefa, Haarlem, The Netherlands). Seeds were sown in medium supplemented with different concentrations of GR24 (a racemic mixture of two stereoisomers—GR245DS and GR24ent-5DS) or KAR1 in 0.1% (v/v) DMSO at 0, 1, 5, and 10 μM. Then, Arabidopsis were transferred to 4 °C for 2 days and moved to grow at 22 °C in the dark, in weak light (1.75 μmol m−2 s−1) or light (23 μmol m−2 s−1) for 4 days. The hypocotyl length was measured with ImageJ software.
Preformation of Arabidopsis STH7 overexpression and STH7-SRDX. Arabidopsis STH7ox was obtained by overexpression of the B-box zinc finger protein STH7 which was driven by a CaMV35S promoter in the wild-type Arabidopsis ecotype Col background. Functionally defective mutants of STH7 were created by using chimeric repressor silencing technology.Citation22) The SRDX motif was fused to the C-terminal end of STH7 and expressed under a CaMV35S promoter in wild-type Arabidopsis ecotype Col. Three homologous STH7ox lines (STH7ox-2, STH7ox-3, and STH7ox-5) and three homologous STH7-SRDX lines (STH7-SR-2, STH7-SR-4, and STH7-SR-5) were used as the representative lines. Various STH7 mutant lines were planted at 22 °C under a 16/8 light/dark cycle for phenotype observation. The hypocotyl lengths were measured at 7 days old whereas petiole lengths were measured at 14 and 21 days old.
Quantitative real-time PCR
Quantitative real-time PCR (qRT-PCR) was performed as follows. Plant samples were frozen and homogenized in liquid nitrogen, and total RNA was extracted from the samples with a Total RNA Extraction Kit Mini for plants (RBC Bioscience, New Taipei City, Taiwan). Complementary DNA (cDNA) was synthesized using ReverTra Ace® qPCR RT Master Mix with gDNA remover (Toyobo, Osaka, Japan), and was used in qRT-PCR. qRT-PCR was performed according to the instructions provided with the PCR Thermal Cycler Dice (Takara Bio, Shiga, Japan), using the SYBR Premix Ex Taq system (Takara Bio). Transcript levels were normalized to those of ACT7. To investigate the expression levels of STH7 in wild-type Arabidopsis ecotype Col (hereafter wild-type), wild-type plants were grown under weak light conditions for 4 days. The plants were then incubated in 0.1% (v/v) DMSO as a control, 10 μM GR24 or 10 μM KAR1 solution prepared in 1/2 MS medium without sucrose and phyto agar for 3 h. The STH7 transcript levels were checked by using STH7 primers: STH7-forward 5′-CCAATAAACTAGCCGGGAAA-3′ and STH7-reverse 5′-GCTCTGTCTTCTTGGCAAAAT-3′. To investigate the STH7 expression levels in wild-type Arabidopsis, three STH7ox lines (STH7ox-2, STH7ox-3, and STH7ox-5) and three STH7-SRDX lines (STH7-SR-2, STH7-SR-4, and STH7-SR-5), wild-type Arabidopsis, STH7ox and STH7-SRDX plants were grown under weak light conditions for 4 days. Plants were collected to extract RNA and perform qRT-PCR in the same manner as described above. To examine the effects of SL and KAR on the expression levels of photosynthesis-related genes, wild-type Arabidopsis, STH7ox-3, STH7ox-5, and STH7-SR-5 plants were grown under dark conditions for 4 days. Then, plants were incubated in 0.1% (v/v) DMSO as a control, 10 μM GR24 or 10 μM KAR1 solution prepared in 1/2 MS medium without sucrose and phyto agar for 24 h. Samples were collected to extract RNA and perform qRT-PCR in the same manner as described above. The following photosynthesis-related primers were used: LHCB1-forward 5′-CCATTTGGGCCACTCAAGTTATC-3′ and LHCB1-reverse 5′-AGCCTCTGGGTCGGTAGCAAG-3′; rbcS-forward 5′-GTTAGCTGCATGAAGGTGTGG-3′ and rbcS-reverse 5′-ACGGTACACAAATCCGTGCTCCA-3′; CHS-forward 5′-GGCTATTGGCACTGCTAACCCTGAG-3′ and CHS-reverse 5′-GTGACGTTTCCGAATTGTCGACTTG-3′. ACT7 was used as the constitutively expressed control gene throughout the experiment. The ACT7 primers used were ACT7-forward 5′-GATATTCAGCCACTTGTCTGTGAC-3′ and ACT7-reverse 5′-CATGTTCGATTGGATACTTCAGAG-3′.
Anthocyanin and chlorophyll measurement
Wild-type Arabidopsis and various STH7ox and STH7-SRDX mutant lines were planted in 1/2 MS medium containing 3% sucrose and 0.8% phyto agar under dark or weak light conditions for 7 days or light conditions for 7 and 14 days, respectively. Arabidopsis grown under darkness were transferred to the light for 6 or 12 h to de-etiolate. After that, 10–20 mg of cotyledons and leaves were collected for chlorophyll and anthocyanin extraction. The chlorophyll content was measured according to Porra et al.Citation23) Plant samples were added to 1 mL dimethylformamide (DMF) and kept at 4 °C overnight. After extraction, chlorophyll content was measured at 647 and 664 nm. Total chlorophyll (total Chl), chlorophyll a (Chl a), and chlorophyll b (Chl b) were calculated by the following equations: total Chl = (17.67 × A647) + (7.17 × A664), Chl a = (12 × A664) − (3.11 × A647), and Chl b = (20.78 × A647) − (4.88 × A664). Anthocyanin content was measured according to Ito et al.Citation24) Methanol in 1% HCl was used as an extraction solution. Plant samples were added to 300 μL of extraction solution and kept at 4 °C overnight. Then, 200 μL of distilled water and 200 μL of chloroform were added and the mixture was centrifuged at 10,000 ×g for 1 min. The anthocyanin content was measured at 530 nm. The amount of anthocyanin was calculated by A530/fresh weight (FW). Moreover, wild-type Arabidopsis, STH7ox-3, STH7ox-5, and STH7-SR-5 plants were grown in 1/2 MS medium containing 0.1% (v/v) DMSO as a control, 10 μM GR24 or 10 μM KAR1 under light conditions for 7 days. After that the cotyledons from each treatment were collected and anthocyanin was extracted in the same manner.
Results
SL and KAR reduce Arabidopsis hypocotyl elongation under weak light conditions
GR24 or KAR1 at 0, 1, 5, and 10 μM was applied to wild-type Arabidopsis as a synthetic SL and a KAR, respectively. The results showed that 5 and 10 μM of GR24 significantly decreased Arabidopsis hypocotyl length under weak light conditions when compared with their control (Fig. (A) and (C)). Only the highest concentration of KAR1 could significantly reduce hypocotyl elongation (Fig. (B) and (D)). When the effect of light conditions was compared between dark, weak light and light conditions, GR24 and KAR1 clearly showed the inhibition of hypocotyl elongation under weak light conditions (Supplemental Fig. 1). Therefore, weak light conditions were selected for the observation of hypocotyl elongation in further experiments. Moreover, it is noteworthy that GR24 and KAR1 at the highest concentrations in this test slightly decreased the hypocotyl length of wild type Arabidopsis under light and dark conditions (Supplemental Fig. 1).
Fig. 1. Strigolactone and karrikin inhibit the hypocotyl length of 4-day-old wild-type Arabidopsis under weak light conditions.
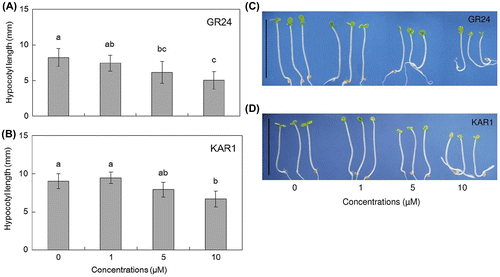
Characterization of STH7ox and STH7-SRDX
Wild-type Arabidopsis were grown under weak light conditions and incubated with 10 μM GR24 or 10 μM KAR1 for 3 h. Treatments of both GR24 and KAR1 up-regulated the expression of STH7 (At4g39070); however, GR24 expressed STH7 at a level significantly higher than KAR1 (Fig. (A)). As it was reported that SL and KAR reduced Arabidopsis hypocotyl elongation,Citation13,21) and this inhibition of hypocotyl elongation was one of the light-adapted responses,Citation1,2) we assumed that there should have been a relation between SL, KAR and light-adapted development.
Fig. 2. Quantitative RT-PCR analysis of STH7 expression level.
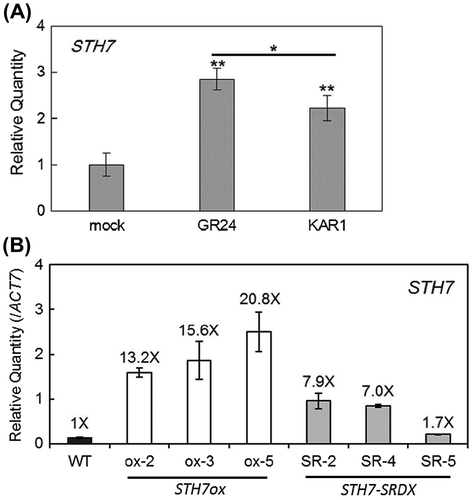
To understand how STH7 functions in photomorphogenesis, STH7ox and STH7-SRDX lines were used in this study. The qRT-PCR results showed that in three mutant STH7ox lines, STH7ox-2, STH7ox-3, and STH7ox-5, STH7 transcript was strongly expressed at 13.2-, 15.6-, and 20.8-fold the wild-type level, respectively (Fig. (B)). In three mutant STH7-SRDX lines, STH7-SR-2, STH7-SR-4, and STH7-SR-5, STH7 was also expressed at a higher level than in the wild type, which may result in the repression of endogenous STH7 function (Fig. (B)).
When Arabidopsis was grown on 1/2 MS media under light conditions for 7 days, three mutant STH7-SRDX lines exhibited longer hypocotyls than the wild type. On the other hand, the hypocotyl length of one mutant STH7ox line, STH7ox-5, was shorter than that of the wild type and the hypocotyl lengths of the other lines, STH7ox-2 and STH7ox-3, were at the same level as that of the wild type (Fig. (A) and (C)). When Arabidopsis was grown under 16/8 light/dark cycle, the petiole lengths of 14-day-grown STH7-SR-2 and STH7-SR-4 were significantly longer than those of the wild type and the same result was observed in 21-day-grown STH7-SRDX mutants. Meanwhile, the petiole lengths of STH7ox-2 and STH7ox-3 were similar to those of the wild type except for the petiole length of STH7ox-5 which was shorter than that of the wild type (Fig. (B) and (D)).
Fig. 3. Phenotype of STH7-ox and STH7-SRDX mutants.
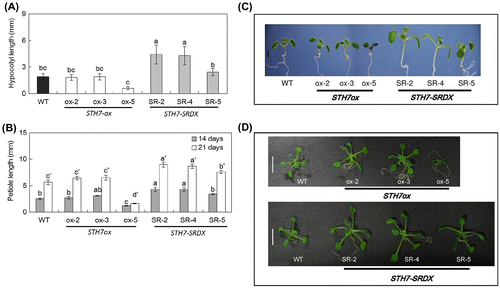
SL and KAR reduces hypocotyl elongation of STH7ox mutants
Not only GR24 and KAR1 could reduce hypocotyl elongation in wild-type Arabidopsis but they also decreased the hypocotyl length of STH7ox mutants (Fig. ). The hypocotyl lengths of all STH7ox mutants (STH7ox-2, STH7ox-3, and STH7ox-5) were significantly reduced by GR24 treatment in a concentration-dependent manner. Among the three STH7ox lines, the hypocotyl length of STH7ox-5 was the shortest even without application of GR24 (Fig. (A)). The extent of the reduction of hypocotyl length by GR24 treatment was lower in all STH7-SRDX lines than in the wild type. In particular, the STH7-SR-5 line was clearly insensitive to GR24 (Fig. (A)). KAR1 treatment of STH7 mutants at both 5 and 10 μM reduced the hypocotyl length of all STH7ox and STH7-SRDX lines; however, STH7-SRDX lines were less sensitive to KAR1 than STH7ox lines and the wild type (Fig. (B)).
Fig. 4. Strigolactone and karrikin decrease hypocotyl elongation of STH7-ox depending on their concentrations.
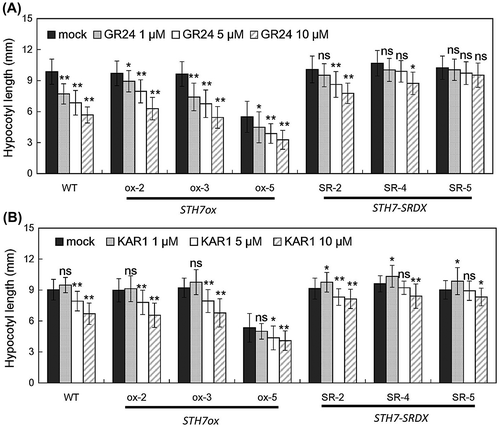
SL response of Arabidopsis in hypocotyl elongation is mediated by D14 and KAI2 while KAR response is mediated only by KAI2
Arabidopsis SL and KAR mutants were used to investigate the effect of SL and KAR on light-adapted development. Hypocotyl elongation of the Arabidopsis signaling mutant max2-1 was not affected either by treatment with GR24 or with KAR1. On the other hand, GR24 significantly reduced the hypocotyl length of the Arabidopsis SL biosynthesis mutant max3-1 (Fig. (A)). These data imply that MAX2 is important for both SL and KAR signal transduction. Next, SL receptor mutant d14-1 and KAR receptor mutant kai2 were used to examine the response of SL and KAR. KAR1 treatment significantly induced the reduction of d14-1 hypocotyl length under weak light conditions, while GR24 did not significantly decrease d14-1 hypocotyl length. In contrast, GR24 application slightly reduced kai2 hypocotyl length but KAR1 did not (Fig. (B)). These results suggest that both D14 and KAI2 mediate the response of SL, whereas only KAI2 mediates the KAR response in hypocotyl elongation of weak light-grown Arabidopsis.
Fig. 5. Response of strigolactone and karrikin on hypocotyl elongation.
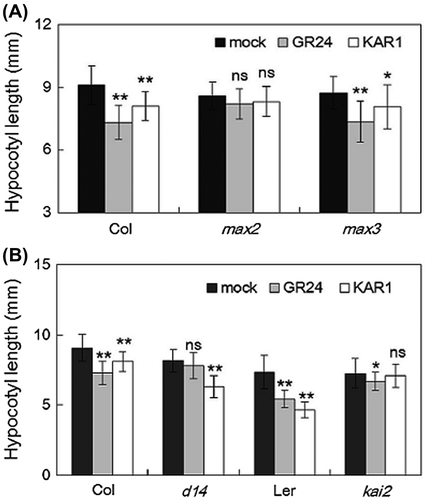
STH7 functions in the increase of anthocyanin and chlorophyll content during (or in the process of) photomorphogenesis
Increases of anthocyanin and chlorophyll content are well-known parameters for detection of plant responses to light.Citation2,5) When wild type, STH7ox, and STH7-SRDX mutants were grown in the dark, the anthocyanin content of all of them was undetectable, while in seedlings de-etiolated by light irradiation of the etiolated seedlings above for 6 and 12 h, anthocyanin levels in STH7ox-3 and STH7ox-5 mutants were significantly higher than those in the wild type and all STH7-SRDX mutant lines (Fig. (A)). The accumulation of a larger amount of anthocyanin in STH7ox-3 and STH7ox-5 mutants was also observed under both weak light and light conditions (Supplemental Fig. 2(A)). Furthermore, the chlorophyll content in de-etiolated Arabidopsis was investigated. The results showed that the total Chl, Chl a, and Chl b content in the de-etiolated seedlings after 6 h of light irradiation was non-significantly different among wild-type Arabidopsis, STH7ox and STH7-SRDX mutants (Fig. (B)). When Arabidopsis seedlings were placed in the light for 12 h, the STH7ox-5 mutant had a clearly increased total Chl content. Furthermore, total Chl content in STH7ox-2 and STH7ox-3 mutants was slightly higher than that in the wild type and STH7-SRDX (Fig. (B)). The results showed that Chl a and Chl b content of STH7ox mutants was slightly higher and significantly higher than the wild type, respectively, and the increase of total Chl in STH7ox was associated with the changes of Chl a and Chl b. On the other hand, in all STH7-SRDX lines, Chl a and Chl b content was almost at the same level as the wild type (Fig. (C) and (D)). Significant accumulation of total Chl in STH7ox mutants compared to the wild type and STH7-SRDX was also found in 7-day-grown Arabidopsis under weak light and normal light conditions (Supplemental Fig. 2(B)). The higher production of total Chl in all STH7ox mutants under weak light conditions was related to the higher content of Chl b. Under weak light conditions, Chl b content in STH7-SR-5 was lower than in the wild type whereas Chl a content in this mutant was similar to that in wild-type Arabidopsis (Supplemental Fig. 2(C) and (D)). When Arabidopsis was grown in the light for 14 days, STH7-SRDX mutants had increased total Chl, Chl a, and Chl b content similar to or higher than wild-type Arabidopsis (Supplemental Fig. 2(C) and (D)).
Fig. 6. Anthocyanin (A), total chlorophyll (B), chlorophyll a (C), and chlorophyll b (D) content in various STH7-ox and STH7-SRDX mutant lines.
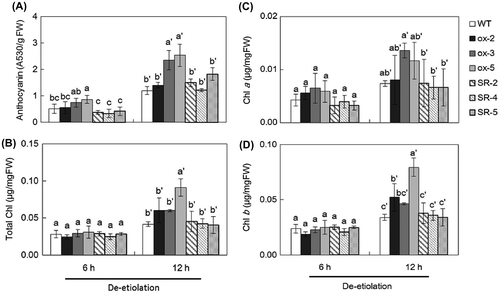
SL enhances anthocyanin content in Arabidopsis more than KAR
Because the anthocyanin content was strongly enhanced in STH7ox-3 and STH7ox-5 lines (Fig. (A), Supplemental Fig. 2(A)), SL and KAR functions might be associated with an increase of anthocyanin production. To check the involvement of SL and KAR in this process, wild-type Arabidopsis, STH7ox and STH7-SRDX mutants were grown in medium containing GR24 or KAR1 under light conditions. STH7ox-3 and STH7ox-5 were selected because these lines showed enhanced accumulation of the anthocyanin content in response to light. Because there was no significant difference between three STH7-SRDX mutants in the extent of the accumulation of the anthocyanin contents, we selected one STH7-SRDX line, STH7-SR-5 as a representative. The results showed that both GR24 and KAR1 significantly enhanced the anthocyanin content in the wild type. However, the increase of anthocyanin content by GR24 was more than by KAR1 (Fig. ). In STH7ox and STH7-SRDX mutants, only the addition of GR24 significantly increased the anthocyanin content. Although SL enhanced the anthocyanin content in the STH7-SRDX mutant, its production was still lower than that in STH7ox-3 and STH7ox-5 (Fig. ).
Fig. 7. Effect of strigolactone and karrikin on anthocyanin content.
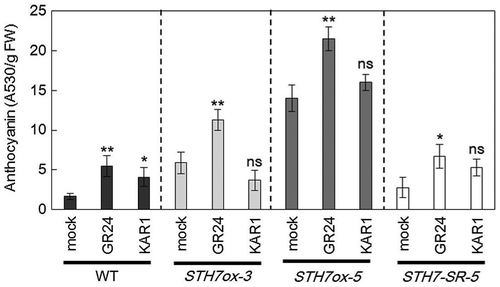
STH7 regulates the expression of photosynthesis-related genes that are induced by SL and KAR
Similar to the anthocyanin content, Chl a and Chl b content was higher in all STH7ox lines than in the wild type, and was almost at the same level in all STH7-SRDX lines as in the wild type (Fig. ). Therefore, STH7ox-3, STH7ox-5 and STH7-SR-5 were selected to examine the expression level of photosynthesis-related genes. Arabidopsis were grown in the dark for 4 days and then incubated with 1/2 MS media with 0.1% DMSO (control), 10 μM GR24 or 10 μM KAR1 for 24 h. LHCB1, encoding the light-harvesting chlorophyll a/b binding protein, and rbcS, encoding the small subunit of RuBisCO, were selected as representative of photosynthesis-related genes, and CHS encoding chalcone synthase was selected as an anthocyanin synthesis gene. qRT-PCR showed that GR24 treatment significantly increased LHCB1, rbcS, and CHS expression levels in STH7ox-3, STH7ox-5, and wild-type Arabidopsis compared with each of their controls. On the other hand, in the GR24-treated STH7-SR-5 mutant, transcription levels of photosynthesis-related genes were similar to the control groups (Fig. ). Moreover, in the GR24-treated STH7ox-3 mutants (Fig. (A) and (B)) the expression levels of LHCB1 and rbcS were significantly higher than those in wild-type Arabidopsis (Fig. (A) and (B)). When wild type, STH7ox-3 and STH7ox-5 were treated with KAR1, the expression levels of LHCB1 and rbcS were significantly higher than in each of their controls and plants treated with GR24 (Fig. (A) and (B)). In STH7ox-5 the expression level of CHS was the highest among the mutants, while in STH7-SR-5 the expression level of CHS was similar to wild-type Arabidopsis in all treatments (Fig. (C)). When GR24 or KAR1 was added to Arabidopsis, GR24 could up-regulate the expression level of CHS in the wild type, STH7ox-3 and STH7ox-5, but KAR1 could increase the expression level of CHS only in STH7ox-5. Moreover, GR24 was more effective than KAR1 in increasing CHS transcription (Fig. (C)).
Fig. 8. Quantitative RT-PCR analysis of photosynthesis-related gene expression.
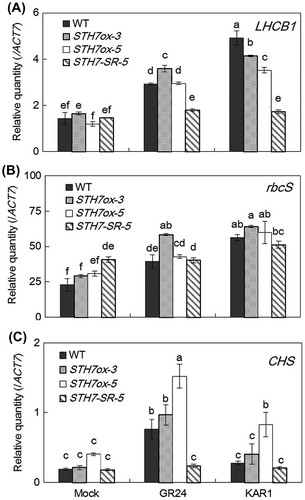
Discussion
When seeds germinate and shoots appear above the soil surface, seedlings undergo de-etiolation or photomorphogenesis. Light sensing is the main signal that impacts on the photomorphogenesis process. Several genes are regulated by light to induce morphological changes in plants.Citation25) COP1 is a negative regulator and HY5 is a positive regulator of photomorphogenesis.Citation26,27) By interacting with COP1, HY5 is degraded by proteasomes and light-adapted development is interrupted.Citation27) Photomorphogenesis in seedling development is also controlled by multiple plant hormones.Citation7,8) In this study, we investigated the involvement of SL and KAR in regulating photomorphogenetic development in Arabidopsis.
Consistent with a report that SL inhibits COP1 function similarly to light signaling,Citation28) GR24 treatment inhibited hypocotyl elongation in wild-type Arabidopsis (Fig. (A)). In addition, KAR1 treatment also inhibited hypocotyl elongation in wild-type Arabidopsis (Fig. (B)). Both light and SL suppress COP1 accumulation in the nucleus together with the accumulation of HY5, which then leads to a reduction of hypocotyl elongation. The decrease of nuclear COP1 by SL application strongly suggests that there should be crosstalk between SL and lightCitation28); however, as shown in Supplemental Fig. 1, 10 μM GR24 little affected the hypocotyl length of wild-type Arabidopsis in the dark. It might be possible to think that SL could affect hypocotyl length not only by crosstalk with light signals but also by substituting light signals and then regulating photomorphogenesis, though deeper investigation is required to unveil the relation between SL and light signaling. In the case of KAR, there is a possibility that hypocotyls could be inhibited by KAR by the same mechanism as SL.
SLs are carotenoid-derived phytohormones containing a butenolide ring (D ring) in their structure.Citation29,30) KARs, byproducts of smoke, also have the butenolide moiety and affect plant growth like SLs.Citation31,32) Treatment with both GR24 and KAR1 significantly decreased hypocotyl elongation under weak light conditions (Fig. ). These results suggest that there should be a relation between SL and light-adapted growth as well as between KAR and light-adapted growth. Moreover, GR24 and KAR1 significantly up-regulated the STH7 expression level in wild-type Arabidopsis grown under weak light conditions (Fig. (A)). STH7 encodes a transcription factor belonging to the double B-box zinc finger family, which is known as a positive regulator of photomorphogenesis.Citation4,9–11) Therefore, SL and KAR might evoke light-adapted development or photomorphogenesis through the function of STH7.
To clarify this hypothesis, we observed phenotypes of STH7ox and STH7-SRDX lines. STH7-SRDX mutants showed longer hypocotyls and longer petioles than wild-type Arabidopsis (Fig. ). Moreover, the hypocotyl elongation of STH7-SRDXs was less retarded by GR24 or KAR1 treatment in contrast with that of wild-type and STH7ox Arabidopsis (Figs. and (A)). These data suggest that SL and KAR modulate the inhibition of hypocotyl elongation dependent on STH7 function. STH7ox-5 Arabidopsis showed the highest expression level of the STH7 gene among three STH7ox lines exhibited shorter hypocotyls and petioles than wild-type Arabidopsis (Fig. (A) and (C)). This result suggests that the overexpression of STH7 should shorten the hypocotyls and petioles. However, because length of hypocotyls and petioles of other STH7ox lines were not different from that of wild-type plants, we cannot exclude the possibility that the phenotypes of STH7ox-5 is not related to the overexpression of STH7.
SL and KAR probably affect photomorphogenesis through different signal pathways although both SL and KAR signaling pathways require MAX2Citation20,33,34) because SLs and KARs are perceived by the paralogous α/β-hydrolases D14 and KAI2, respectively.Citation13,20,35,36) Waters and SmithCitation21) reported that the overexpression of KAI2 is enough to modulate the signaling response of both SLs and KARs in the hypocotyl developing state, and Scaffidi et al.Citation37) reported that only natural forms of SL such as 5-deoxystrigol (5DS) were active through D14, whereas non-natural enantiomers such as ent-5-deoxystrigol (ent-5DS) were active through KAI2. Here, we confirmed that neither GR24 nor KAR1 could inhibit hypocotyl elongation of the max2 mutant (Fig. (A)). Moreover, both GR24 and KAR1 treatment reduced the hypocotyl length of d14 under weak light conditions. However, only GR24 addition decreased kai2 hypocotyl elongation (Fig. (B)). These results suggest that the inhibition of hypocotyl elongation under weak light conditions by GR24 is mediated by both D14 and KAI2. Since the GR24 used in this study was a racemic mixture of chemically synthesized SLs, two stereoisomers—GR245DS and GR24ent-5DS—were separately applied to check the effect on hypocotyl inhibition. Both of these isomers are assumed to inhibit hypocotyl elongation via STH7 because STH7-SRDX lines were less sensitive to a racemic mixture of each stereoisomer than the wild type (Data not shown). In contrast, only GR24ent-5DS showed a significant reduction of d14-1 hypocotyl elongation while only GR245DS significantly inhibited kai2 hypocotyl elongation under weak light conditions (Supplemental Fig. 3). These results support the hypothesis that each stereoisomer of the GR24 racemic mixture is received separately by D14 or KAI2 and activates photomorphogenesis via STH7. In contrast, KAR1 could not reduce the hypocotyl length of the kai2 mutant (Fig. (B)), indicating that KAR is thoroughly perceived by KAI2 and activates photomorphogenesis via STH7 (Fig. ).
Fig. 9. Working model of strigolactone (SL) and karrikin (KAR) effects on light-adapted development or photomorphogenesis.
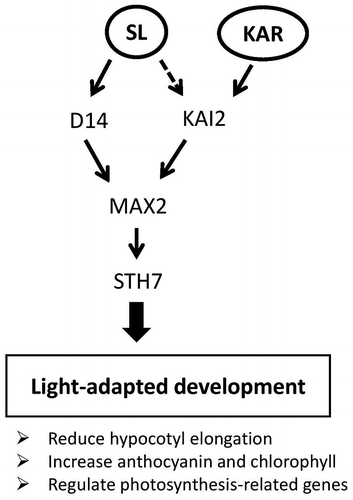
The biosynthesis of chlorophyll and anthocyanin is a process of de-etiolated development.Citation2,5) BBX proteins play a role in photomorphogenesis by inducing chlorophyll and anthocyanin accumulation.Citation9) Close homologues of STH7 such as STH2/BBX21 and STH3/BBX22/LZF1 have been reported as positive regulators of light-adapted development.Citation38–40) STH7 shares amino-acid identity of 71.82% with STH2 and 54.13% with STH3.Citation4) STH3 plays a role as a positive regulator of chloroplast development.Citation39–41) STH3ox exhibited increased anthocyanin and chlorophyll levels under red light while the sth3 mutant showed decreased anthocyanin and chlorophyll levels as well as delayed chloroplast development.Citation40) Moreover, the absence of STH2 in the cop1 mutant partially repressed anthocyanin production.Citation38) Our study demonstrated that de-etiolated STH7ox mutants accumulated more anthocyanin and chlorophyll than wild-type Arabidopsis and STH7-SRDX mutants (Fig. ). This suggests that STH7 mediates chlorophyll and anthocyanin accumulation during light-adapted processes.
The expression levels of photosynthesis- and anthocyanin synthesis-related genes, such as LHCB1, rbcS, and CHS, in wild-type Arabidopsis and STH7ox mutants were up-regulated when treated with GR24 or KAR1 (Fig. ). These three genes are normally increased by light irradiation.Citation28) These results imply that SL and KAR modulate light-adapted development through the up-regulation of photosynthesis-related genes and STH7 is involved in this process (Fig. ). LHCB1 and rbcS are genes related to chlorophyll production.Citation42,43) The up-regulation of these two genes by GR24 and KAR1 in STH7ox-5 treatment could be related to the increase of total chlorophyll content in this mutant (Fig. (B)). Moreover, the CHS gene is associated with anthocyanin biosynthesis.Citation44,45) The fact that the expression level of CHS by GR24 addition was more than by KAR1 addition (Fig. (C)) could be in accord with the result that the increase of anthocyanin content by GR24 addition was more than by KAR1 addition (Fig. ). Further analysis to investigate how expression of the STH7 gene is regulated by SL and KAR and whether STH7 regulates the expression of these genes directly will provide deeper mechanistic insights into SL signaling in photomorphogenesis.
Author contributions
H.N. and T.A. designed research. J.T., Y.N., M.N., and K.M. performed experiments. N.M. and M.O.T. produced the STH7-SRDX lines. T.N. advised on research. J.T., H.N., and T.A. wrote the paper. T.A. supervised research.
Supplemental matrials
The supplemental material for this paper is available at http://dx.doi.org/10.1080/09168451.2016.1254536.
TBBB_1254536_SUPPLEMENTARY_MATERIAL.zip
Download Zip (2.8 MB)Disclosure statement
No potential conflict of interest was reported by the authors.
Funding
This work was supported by CREST, JST, and JSPS KAKENHI [grant number 26440132]. This project was also funded by the Deanship of Scientific Research (DSR), at King Abdulaziz University, Jeddah, under [grant number 1-130-36-HiCi].
References
- Symons GM, Smith JJ, Nomura T, et al. The hormonal regulation of de-etiolation. Planta. 2008;227:1115–1125.10.1007/s00425-007-0685-x
- Chory J, Chatterjee M, Cook RK, et al. From seed germination to flowering, light controls plant development via the pigment phytochrome. Proc Natl Acad Sci U S A. 1996;93:12066–12071.10.1073/pnas.93.22.12066
- Li Q-F, He J-X. BZR1 interacts with HY5 to mediate brassinosteroid- and light-regulated cotyledon opening in Arabidopsis in darkness. Mol Plant. 2015;9:113–125.
- Fan X-Y, Sun Y, Cao D-M, et al. BZS1, a B-box protein, promotes photomorphogenesis downstream of both brassinosteroid and light signaling pathways. Mol Plant. 2012;5:591–600.10.1093/mp/sss041
- Neff MM, Chory J. Genetic interactions between phytochrome A, phytochrome B, and cryptochrome 1 during arabidopsis development. Plant Physiol. 1998;118:27–35.10.1104/pp.118.1.27
- Feng S, Martinez C, Gusmaroli G, et al. Coordinated regulation of Arabidopsis thaliana development by light and gibberellins. Nature. 2008;451:475–479.10.1038/nature06448
- Lau OS, Deng XW. Plant hormone signaling lightens up: integrators of light and hormones. Curr Opin Plant Biol. 2010;13:571–577.10.1016/j.pbi.2010.07.001
- Wang ZY, Bai MY, Oh E, et al. Brassinosteroid signaling network and regulation of photomorphogenesis. Annu Rev Genet. 2012;46:701–724.10.1146/annurev-genet-102209-163450
- Gangappa SN, Botto JF. The BBX family of plant transcription factors. Trends Plant Sci. 2014;19:460–470.10.1016/j.tplants.2014.01.010
- Sarmiento F. The BBX subfamily IV: additional cogs and sprockets to fine-tune light-dependent development. Plant Signal Behav. 2013;8:e23831-1–e23831-7.
- Khanna R, Kronmiller B, Maszle DR, et al. The arabidopsis B-box zinc finger family. Plant Cell. 2009;21:3416–3420.10.1105/tpc.109.069088
- Mashiguchi K, Sasaki E, Shimada Y, et al. Feedback-regulation of strigolactone biosynthetic genes and strigolactone-regulated genes in arabidopsis. Biosci Biotechnol Biochem. 2009;73:2460–2465.10.1271/bbb.90443
- Nelson DC, Flematti GR, Riseborough JA, et al. Karrikins enhance light responses during germination and seedling development in Arabidopsis thaliana. Proc Natl Acad Sci U S A. 2010;107:7095–7100.10.1073/pnas.0911635107
- Cook CE, Whichard LP, Turner B, et al. Germination of witchweed (Striga lutea Lour.): isolation and properties of a potent stimulant. Science. 1966;154:1189–1190.10.1126/science.154.3753.1189
- Akiyama K, Matsuzaki K, Hayashi H. Plant sesquiterpenes induce hyphal branching in arbuscular mycorrhizal fungi. Nature. 2005;435:824–827.10.1038/nature03608
- Gomez-Roldan V, Fermas S, Brewer PB, et al. Strigolactone inhibition of shoot branching. Nature. 2008;455:189–194.10.1038/nature07271
- Seto Y, Kameoka H, Yamaguchi S, et al. Recent advances in strigolactone research: chemical and biological aspects. Plant Cell Physiol. 2012;53:1843–1853.10.1093/pcp/pcs142
- Umehara M, Hanada A, Yoshida S, et al. Inhibition of shoot branching by new terpenoid plant hormones. Nature. 2008;455:195–200.10.1038/nature07272
- Nelson DC, Riseborough JA, Flematti GR, et al. Karrikins discovered in smoke trigger Arabidopsis seed germination by a mechanism requiring gibberellic acid synthesis and light. Plant Physiol. 2009;149:863–873.
- Waters MT, Nelson DC, Scaffidi A, et al. Specialisation within the DWARF14 protein family confers distinct responses to karrikins and strigolactones in Arabidopsis. Development. 2012;139:1285–1295.10.1242/dev.074567
- Waters MT, Smith SM. KAI2- and MAX2-mediated responses to karrikins and strigolactones are largely independent of HY5 in Arabidopsis seedlings. Mol Plant. 2013;6:63–75.10.1093/mp/sss127
- Hiratsu K, Matsui K, Koyama T, et al. Dominant repression of target genes by chimeric repressors that include the EAR motif, a repression domain, in Arabidopsis. Plant J. 2003;34:733–739.10.1046/j.1365-313X.2003.01759.x
- Porra RJ, Thompson WA, Kriedemann PE. Determination of accurate extinction coefficients and simultaneous equations for assaying chlorophylls a and b extracted with four different solvents: verification of the concentration of chlorophyll standards by atomic absorption spectroscopy. Biochim Biophys Acta Bioenerget. 1989;975:384–394.10.1016/S0005-2728(89)80347-0
- Ito S, Nozoye T, Sasaki E, et al. Strigolactone regulates anthocyanin accumulation, acid phosphatases production and plant growth under low phosphate condition in Arabidopsis. PLoS One. 2015;10:1–17.
- Wu SH. Gene expression regulation in photomorphogenesis from the perspective of the central dogma. Annu Rev Plant Biol. 2014;65:311–333.10.1146/annurev-arplant-050213-040337
- Eckardt NA. From darkness into light: factors controlling photomorphogenesis. Plant Cell. 2001;13:219–221.10.1105/tpc.13.2.219
- Osterlund MT, Hardtke CS, Wei N, et al. Targeted destabilization of HY5 during light-regulated development of Arabidopsis. Nature. 2000;405:462–466.
- Tsuchiya Y, Vidaurre D, Toh S, et al. A small-molecule screen identifies new functions for the plant hormone strigolactone. Nat Chem Biol. 2010;6:741–749.10.1038/nchembio.435
- Al-Babili S, Bouwmeester HJ. Strigolactones, a novel carotenoid-derived plant hormone. Annu Rev Plant Biol. 2015;66:161–186.10.1146/annurev-arplant-043014-114759
- Seto Y, Yamaguchi S. Strigolactone biosynthesis and perception. Curr Opin Plant Biol. 2014;21:1–6.10.1016/j.pbi.2014.06.001
- Morffy N, Faure L, Nelson DC. Smoke and hormone mirrors: action and evolution of karrikin and strigolactone signaling. Trends Genet. 2016;32:176–188.10.1016/j.tig.2016.01.002
- Nelson DC, Flematti GR, Ghisalberti EL, et al. Regulation of seed germination and seedling growth by chemical signals from burning vegetation. Annu Rev Plant Biol. 2012;63:107–130.10.1146/annurev-arplant-042811-105545
- Nelson DC, Scaffidi A, Dun EA, et al. F-box protein MAX2 has dual roles in karrikin and strigolactone signaling in Arabidopsis thaliana. Proc Natl Acad Sci U S A. 2011;108:8897–8902.10.1073/pnas.1100987108
- Stanga JP, Morffy N, Nelson DC. Functional redundancy in the control of seedling growth by the karrikin signaling pathway. Planta. 2016;243:1397–1406.10.1007/s00425-015-2458-2
- Nakamura H, Xue Y-L, Miyakawa T, et al. Molecular mechanism of strigolactone perception by DWARF14. Nat Commun. 2013;4:1–10.
- Zhou F, Lin Q, Zhu L, et al. D14-SCFD3-dependent degradation of D53 regulates strigolactone signalling. Nature. 2013;504:406–410.10.1038/nature12878
- Scaffidi A, Waters MT, Sun YK, et al. Strigolactone hormones and their stereoisomers signal through two related receptor proteins to induce different physiological responses in Arabidopsis. Plant Physiol. 2014;165:1221–1232.10.1104/pp.114.240036
- Datta S, Hettiarachchi C, Johansson H, et al. SALT TOLERANCE HOMOLOG2, a B-box protein in Arabidopsis that activates transcription and positively regulates light-mediated development. Plant Cell. 2007;19:3242–3255.10.1105/tpc.107.054791
- Datta S, Johansson H, Hettiarachchi C, et al. LZF1/SALT TOLERANCE HOMOLOG3, an Arabidopsis B-box protein involved in light-dependent development and gene expression, undergoes COP1-mediated ubiquitination. Plant Cell. 2008;20:2324–2338.10.1105/tpc.108.061747
- Kumagai T, Ito S, Nakamichi N, et al. The common function of a novel subfamily of B-box zinc finger proteins with reference to circadian-associated events in Arabidopsis thaliana. Biosci Biotechnol Biochem. 2008;72:1539–1549.10.1271/bbb.80041
- Chang CS, Li YH, Chen LT, et al. LZF1, a HY5-regulated transcriptional factor, functions in Arabidopsis de-etiolation. Plant J. 2008;54:205–219.10.1111/j.1365-313X.2008.03401.x
- Dhingra A, Portis AR, Daniell H. Enhanced translation of a chloroplast-expressed RbcS gene restores small subunit levels and photosynthesis in nuclear RbcS antisense plants. Proc Natl Acad Sci U S A. 2004;101:6315–6320.10.1073/pnas.0400981101
- Meehan L, Harkins K, Chory J, et al. Lhcb transcription is coordinated with cell size and chlorophyll accumulation (studies on fluorescence-activated, cell-sorter-purified single cells from wild-type and immutans Arabidopsis thaliana). Plant Physiol. 1996;112:953–963.10.1104/pp.112.3.953
- Boss PK, Davies C, Robinson SP. Analysis of the expression of anthocyanin pathway genes in developing Vitis vinifera L. cv Shiraz grape berries and the implications for pathway regulation. Plant Physiol. 1996;111:1059–1066.10.1104/pp.111.4.1059
- Solfanelli C, Poggi A, Loreti E, et al. Sucrose-specific induction of the anthocyanin biosynthetic pathway in Arabidopsis. Plant Physiol. 2006;140:637–646.10.1104/pp.105.072579