Abstract
Lichtheimia ramosa is one of the predominant filamentous fungi in Korean traditional nuruk. The nonvolatile and volatile metabolites of L. ramosa cultivated in three growth media: complete medium (CM), potato dextrose broth (PDB), and sabouraud dextrose broth (SDB), were investigated and compared. Among nonvolatile metabolites, serine, lysine, and ornithine increased in CM and PDB cultivated with L. ramosa during the exponential phase. In addition, glucose level increased in CM whereas decreased in PDB and SDB. The major volatile metabolites in the extract samples were acetic acid, ethanol, 3-methyl-2-buten-1-ol, 2-phenylethanol, ethylacetate, 2-furaldehyde, 5-(hydroxymethyl)-2-furaldehyde, 2,3-dihydro-3,5,-dihydroxy-6-methyl-4H-pyran-4-one, and α-humulene. In particular, the levels of volatile metabolites related to makgeolli (e.g., acetic acid, ethanol, and ethyl acetate) were highest in extracts cultivated in CM. On the other hand, the level of 2-phenylethanol was relatively higher in PDB and SDB, possibly due to there being more phenylalanine present in the biomass sample in media.
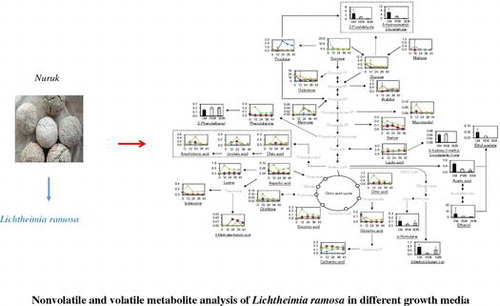
Lichtheimia ramosa, a member of the Mucorales (as are Rhizopus species), is pathogenic to humans, causing mucormycosis with a symptom of fungal growth at the skin, brain, and other sites in subjects with weak immune systems.Citation1–4) L. ramosa has also been investigated for the production of several enzymes such as xylanase and β-glucosidase used in the food (beverages), pharmaceutical, and biofuel industries.Citation5–7) Recently, it has been revealed to be one of the predominant fungal species isolated in nuruk.Citation2,8) In addition, L. ramosa isolated from traditional nuruk is relatively high in α-amylase and exhibits strong saccharogenic power, starch hydrolysis, and acid-producing activity.Citation9)
Makgeolli is a traditional rice wine in Korea that is generally manufactured with steamed rice as a major ingredient and nuruk or koji as a microbial starter for saccharifying starch to glucose.Citation10,11) Nuruk is a traditional mixed culture that is primarily based on rice, wheat, barley, or rye, and it includes diverse air-borne micro-organisms such as filamentous fungi, yeasts, and bacteria occurring naturally, whereas koji contains a single culture micro-organism, mostly Aspergillus oryzae.Citation12) These cultures are sources of amylolytic and proteolytic enzymes used in the fermentation of makgeolli.Citation13)
The fungi associated with nuruk produce amylolytic and proteolytic enzymes that play important roles in starch saccharification and protein or peptide digestion, respectively.Citation14,15) In the process of fermenting makgeolli, fungi play an important role in providing diverse enzymes including amylase, glucoamylase, glucose oxidase, lipase, and protease, which can convert macromolecules (starch, proteins, and lipids) into small molecules (sugars, amino acids, and fatty acids) that yeasts can utilize.Citation16,17) Aspergillus and Rhizopus species are the most common fungi found in nuruk, and their effects on the quality of nuruk and makgeolli have been investigated.Citation8,10,18,19) The micro-organisms in nuruk are responsible for various qualities of makgeolli, including its taste (due to organic acids and amino acids) and aroma (due to esters and higher alcohols).Citation20,21) In addition, the type of fungus can also significantly affect these cultures due to the different metabolites produced by different fungi and media.Citation20,22) However, even though several studies have investigated Aspergillus species in nuruk with the aim of improving its quality, few studies have investigated the association of L. ramosa with nuruk.
The metabolites in fermented foods such as soy sauce, makgeolli, doenjang, and cheonggukjang, Korean traditional fermented soybean pastes, and related starter cultures including koji and nuruk have been investigated previously.Citation8, 23–29) Microbial metabolites have also been analyzed for studying the unique chemical fingerprints of metabolite profiles, with characterization of the micro-organisms.Citation30,31) In particular, the characteristics of metabolites of yeasts such as Saccharomyces cerevisiae and fungi such as Aspergillus, Penicillium, and Rhizopus species have been compared.Citation30,32–34) Various high-throughput analytical methods such as gas chromatography–mass spectrometry (GC–MS),Citation29,30,35,36) high-performance liquid chromatography,Citation37), and capillary electrophoresisCitation38,39) have been used to analyze microbial metabolites.Citation29) GC–MS is a comprehensive and nonbiased methodology with high sensitivity, great resolution, and quantification power that has been used for quantifying volatile metabolites in micro-organism extracts such as Aspergillus, Fusarium, Mucor, Penicillium, and Trichoderma species.Citation36,40,41) In addition, gas chromatography coupled to time-of-flight mass spectrometry (GC–TOF-MS) has been used to analyze amino acids, fatty acids, carbohydrates, and organic acids in intracellular metabolites due to its relatively high reproducibility, high resolution, throughput, and sensitivity, enabling deconvolution analysis.Citation28,30,42)
The diverse metabolites related to unique aromas, flavors, and textures are produced and influenced by the associated microbiological processes.Citation43) The metabolites in nuruk fermented with specific micro-organisms such as A. oryzae have been profiled previously,Citation25,26,44) but no previous study has analyzed the nonvolatile and volatile metabolites of L. ramosa isolated from nuruk. The objective of the current study was to investigate the nonvolatile and volatile metabolites of L. ramosa cultivated in different media using GC–TOF-MS and GC–MS, respectively. The results obtained can be applied to understanding and utilization of L. ramose, which can be possibly related to the qualities of some fermented products such as nuruk and makgeolli. To our knowledge, this is the first study to analyze nonvolatile and volatile metabolites in L. ramosa isolated from nuruk.
Materials and methods
Chemicals and reagents
Yeast extract, potato dextrose broth (PDB), sabouraud dextrose broth (SDB), and peptone were obtained from Becton Dickinson (Sparks, MD, USA). Threitol and magnesium sulfate heptahydrate were purchased from Tokyo Chemical and SHOWA Chemical (Tokyo, Japan), respectively. Pyridine, norleucine, tropic acid, heptadecanoic acid, 4-ethylphenol, N,O-bis(trimethylsilyl) trifluoroacetamide (BSTFA) with 1% trimethylchlorosilane (TMCS), methoxyamine hydrochloride, sucrose, sodium nitrate, potassium chloride, potassium phosphate, citric acid, zinc sulfate monohydrate (ZnSo4∙H2O), ammonium iron sulfate hexahydrate [Fe(NH4)2(SO4)2∙6H2O] copper sulfate penthydrate (CuSO4∙5H2O), manganese sulfate (MnSO4), boric acid, sodium molybdate dehydrate (Na2MoO4∙2H2O), riboflavin, pyridoxine, nicotinamide, ascorbic acid, p-aminobenzoic acid, choline, folic acid, biotin, and inositol were purchased from Sigma–Aldrich (St. Louis, MO, USA).
Cultivation of L. ramosa
In order to evaluate nonvolatile and volatile metabolites produced in L. ramosa isolated from nuruk according to the culture medium and cultivation times, L. ramosa was cultured in complete medium (CM), PDB, and SDB at 37 °C for 48 h. The composition of each medium was as follows: CM consists of 1 g of yeast extract, 30 g of sucrose, 2.5 g of peptone, 2 g of sodium nitrate, 1 g of potassium phosphate, 0.5 g of magnesium sulfate heptahydrate, 0.5 g of potassium chloride, 0.2 mL of trace element solution (5 g of citric acid, 1 g of ZnSo4∙H2O, 5 g of Fe(NH4)2(SO4)2∙6H2O, 250 mg of CuSO4∙5H2O, 50 mg of MnSO4, 50 mg of boric acid, 50 mg of Na2MoO4∙2H2O in distilled water), and 10 mL of vitamin stock solution (100 mg of thiamine, 30 mg of riboflavin, 75 mg of pyridoxine, 200 mg of pantothenic acid, 75 mg of nicotinamide, 5 mg of ascorbic acid, 5 mg of p-aminobenzoic acid, 200 mg of choline, 5 mg of folic acid, 5 mg of biotin, 4 g of inositol in 50% ethanol). PDB is composed of 4 g of potato infusion and 20 g of dextrose in distilled water. The composition of SDB was 5 g of enzymatic digest of casein, 5 g of enzymatic digest of animal tissue, and 20 g of dextrose in distilled water. Erlenmeyer flasks containing growth media were sterilized at 121 °C for 15 min.
One milliliter of spore suspension (1 × 10Citation7 CFU/mL) of L. ramosa was used for the inoculation. Erlenmeyer flasks containing 40 mL of each media with screw cap were placed in a shaking incubator (Vision Scientific Co., Ltd., Bucheon-si, Gyeonggi-do, Korea) at 220 rpm and 37 °C for 0, 12, 24, 36, and 48 h. In order to obtain the dry cell weight of L. ramosa for growth curve, cultivation media were filtered on the filter paper (150-mm diameter; WhatmanTM, Piscataway, NJ, USA). The filter paper was then dried in a vacuum drying system (HB-501VL, HANBAEK, Bucheon-si, Gyeonggi-do, Korea) at 65 °C for 24 h. The cultivation media samples were collected every 12 h during 48-h cultivation. Each cultivation and sampling was conducted in triplicate.
Analysis of nonvolatile metabolites by gas chromatography–time of flight-mass spectrometry
Modified fast filtration methodCitation45) was used to extract nonvolatile metabolites. Five milliliters of sample were filtered through a nylon membrane filter (0.45-μm pore size, 30-mm diameter, Whatman, Piscataway, NJ, USA) under vacuum before adding 5 mL of water to eliminate remaining media. Filtered cell biomass was mixed with 20 mL of acetonitrile/water mixture (1:1) at −22 °C for 1 min, immersed in liquid nitrogen, and thawed on ice. The thawed mixture was centrifuged at 3857.1 × g and 4 °C for 20 min (Union 32R Plus, Hanil Science Industrial Co., Ltd., Incheon, Korea). The supernatant (1.5 mL) was transferred into a safe-lock tube and then vacuum dried using a Centri-Vap (Labconco Corp., Kansas City, Mo, USA). The extract was resuspended in 500 μL of acetonitrile:water (1:1) mixture at 0 °C and then dried. The dried extract was derivatized using 60 μL of 20-mg/mL methoxyamine hydrochloride in pyridine (Sigma–Aldrich, ST. Louis, MO, USA) at 30 °C for 60 min and then 80 μL of N,O-bis(trimethylsilyl)trifluoroacetamide with 1% trimethylchlorosilame (BSTFA + 1% TMCS, Supelco, Bellefonte, PA, USA) at 70 °C for 60 min prior to GC–TOF-MS analysis. The derivatized nonvolatile metabolite samples were analyzed using a 6890 N GC (Agilent Technologies, Palo Alto, CA, USA) connected to a Pegasus III TOF MS (Leco, St. Joseph, MI, USA) equipped with a DB-5MS column (30 m length x 0.25 mm i.d. × 0.25 μm film thickness; J&W Scientific, Folsom, CA, USA). The flow rate of helium was constantly 1.0 mL/min. The derivatized sample (1 μL) was injected in splitless mode with the injector temperature at 270 °C. The oven temperature program was as follows: initial temperature of 80 °C for 5 min raised to 180 °C at 10 °C/min, which was kept for 5 min, increased to 240 °C at 8 °C/min, which was held for 5 min, elevated to 290 °C at 10 °C/min, and then maintained for 10 min. The detector transfer line and ion source temperatures were 280 and 200 °C, respectively. The data acquisition rate was 20 scans/s in the mass range of m/z 35 to 500. The electron ionization (EI) was carried out at 70 eV.
Nonvolatile metabolites were identified by comparing retention times and mass spectra with those of authentic reference compounds. Fiehn library, replibrary, mainlibrary, Wiley9, and in-house library were used to identify the metabolites whenever the authentic compounds were not available. The relative peak areas of nonvolatile metabolites were obtained by comparing their peak areas to those of internal standard compounds, (2S)-2-aminohexanoic acid (norleucine) [100 μg/mL in water (w/v)] for amino acids, (2R,3R)-butane-1,2,3,4-tetrol (threitol) [100 μg/mL in water (w/v)] for sugars, 3-hydroxy-2-phenylpropanoic acid (tropic acid) [100 μg/mL in water (w/v)] for organic acids, and heptadecanoic acid [100 μg/mL in hexane (w/v)] for fatty acids, respectively.
Extraction and analysis of volatile metabolites by gas chromatography–mass spectrometry
Stir bar sorptive extraction (SBSE) was employed for the extraction of volatile metabolites in L. ramosa samples cultivated in growth media. Volatile metabolites were adsorbed onto Gerstel Twister® EG-silicone coated with ethylene glycol (32 μL phase volume, 10-mm length; GERSTEL GmbH & Co. KG, Mülheim an der Ruhr, Germany). A stir bar was placed into a 10-mL vial containing 8 mL of the samples and kept at 210 × g for 60 min. The desorption temperature program of a stir bar was as follows: initial temperature at 40 °C for 0.5 min, increased to 220 °C at 120 °C/min, and held at 220 °C for 5 min. During desorption, CIS-4 PTV temperature was maintained at −80 °C. The analysis of volatile metabolites was performed using an Agilent 7890B gas chromatograph-5977A mass detector (Agilent Technologies, Palo Alto, CA, USA) equipped with DB-wax column (30 m length x 0.25-mm i.d. × 0.25 μm film thickness, J&W Scientific, Folsom, CA, USA). Helium was used as a carrier gas at a constant flow rate of 0.8 mL/min. The oven temperature was maintained at 40 °C for 5 min, elevated to 230 °C at 4 °C/min, and then maintained for 10 min. The transfer line and ion source temperatures were 280 and 250 °C, respectively. Mass spectra were obtained in the EI mode (70 eV) with a mass scan rage of m/z 35–350 at a rate of 4.5 scans/s.
The identification of volatile metabolites was achieved using retention times and mass spectral database (Wiley9n.1 and NIST08). The relative peak area of volatile metabolites was determined by comparing with that of internal standard, 4-ethylphenol [100 ppm (w/v) in methanol].
Statistical analysis
Multivariate analysis of variance (MANOVA) was performed using SPSS (Version 12.0, Chicago, IL, USA) to verify the differences in metabolites between samples.
Results and discussion
L. ramosa growth
This study cultivated L. ramosa at 37 °C for 48 h; since, in commercial applications involving media such as SDB and PDB or containing agar, it is generally cultivated for 48 h at 28–37 °C based on its growth curve.Citation7,46,47) The growth curves of L. ramosa cultivated in CM, PDB, and SDB for 48 h were obtained (Supplemental Figure S; see Biosci. Biotechnol. Biochem. Website). L. ramosa cultivated in CM showed a monophasic exponential growth from inoculation until 24 h, while cultivation in PDB or SDB produced exponential growth from inoculation until 12 h. The stationary phases of L. ramosa cultivated in CM, PDB, and SDB were 24–36, 12–36, and 12–24 h, respectively. The death phase begun after 36 h of cultivation in CM and PDB, but after 24 h of cultivation in SDB.
Growth medium-dependent nonvolatile metabolites in biomass of L. ramosa
Nonvolatile metabolites in biomass of L. ramosa cultivated in CM, PDB, and SDB for 48 h were analyzed using GC–TOF-MS after derivatization. Nonvolatile metabolites in biomass samples cultivated in three different media were quantified with their relative peak areas on the DB5 column (Supplemental Tables S1–S3; see Biosci. Biotechnol. Biochem. Website). The following 58 nonvolatile metabolites were identified in the biomass sample cultivated in CM: 14 amino acids, 9 carbohydrates, 17 fatty acids, and 18 organic acids. Cultivation in PDB produced 56 nonvolatile metabolites (13 amino acids, 8 carbohydrates, 17 fatty acids, and 18 organic acids), while cultivation in SDB produced 14 amino acids, 9 carbohydrates, 15 fatty acids, and 18 organic acids.
Fig. shows the changes in the levels of nonvolatile and volatile metabolites detected from the biomass and the extracts of L. ramosa cultivated in different growth media during 48 h, which are implicated by their possible metabolic pathways.
Fig. 1 Summary of possible metabolic pathways for Lichthemia ramosa. Black lines: metabolites were detected, and dashed lines involve several reaction steps. Line charts refer to nonvolatile metabolites of biomass of L. ramosa during 48 h, and bar charts indicate volatile metabolites of the extracts of L. ramosa at 48 h cultivated in different growth media: CM (•), PDB (■), and SDB (▲). The x and the y-axes indicate fermentation time and relative peak area, respectively. Linoleic acid, (9Z,12Z)-9,12-octadecadienoic acid; oleic acid, (9Z)-octadec-9-enoic acid; succinic acid, butanedienoic acid.
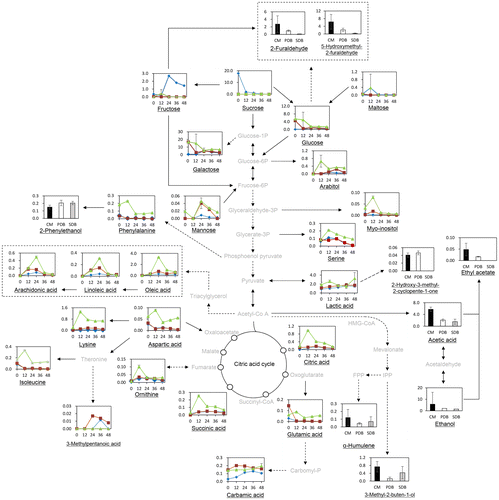
Amino acids in biomass of L. ramosa cultivated in CM were similar to those in PDB but not in SDB during 48 h of cultivation. Most of the amino acids identified decreased rapidly during the exponential phase (to 12 h), and then increased at 24 h (except for serine, ornithine, and lysine).
In biomass of L. ramosa cultivated in CM, serine was increased at 12 h and then subsequently decreased, whereas ornithine increased until 24 h and then decreased. However, in PDB, ornithine was increased at 12 h and decreased until 48 h, while lysine was increased at 24 h and decreased until 48 h. Most of the amino acids in biomass of L. ramosa cultivated in SDB increased until 12 h and then subsequently decreased, while isoleucine, aspartic acid, glutamic acid, and phenylalanine increased during the death phase (36–48 h).
The decrease in amino acids during the exponential phase could be due to their utilization as nitrogen or carbon sources for supporting fungal growth.Citation48,49) Gottleib and van Etten demonstrated that the total nitrogen against dry cell weight of fungi increased rapidly during lag phase when spores were germinated and formed hyphae. During the exponential phase, the percentage of total nitrogen decreased. This decrease in total nitrogen could be explained by the synthesis of other constituents of the mycelium. After that, total nitrogen increased with dry weight of mycelium.Citation48) Amino acids could be degraded due to the nitrogen balance which utilizes amino acids as nitrogen sources during early growth stage.Citation49) In addition, amino acids could be converted into aldehydes, alcohols, sulfur-containing compounds, and lactones via the microbial metabolic pathway.Citation50) In this study, the tendency for amino acids to decrease in biomass of L. ramosa cultivated in CM and PDB during the exponential phase could be explained by amino acid degradation via the metabolic pathway of micro-organisms. Specifically, fusel alcohols are produced from amino acids such as valine, leucine, isoleucine, methionine, and phenylalanine via with Ehrlich pathway.Citation50,51) Ehrlich pathway is composed of transaminase, decarboxylase, and alcohol dehydrogenase processes. Transamination of amino acids following decarboxylation and alcohol dehydrogenase reactions can generate α-keto acid, aldehyde, and alcohols, respectively.Citation51) In particular, some enzymes, such as mitochondrial branched-chain amino acid transferase (Twt1p) and cytosolic isozyme (Twt2p) associated with transamination reaction, are highly expressed during exponential phase and repressed during stationary phase.Citation52) Thus, fusel alcohols are usually generated during exponential growth phase. On the other hand, the increase in amino acids after 24 h of cultivation in SDB could be explained by the fungal synthesis of amino acids utilizing nitrogen compounds included in the media that contained sources of glucose and inorganic nitrogen.Citation53,54)
Most carbohydrates in biomass of L. ramosa cultivated in CM, PDB, and SDB increased at the end of the exponential phase (24 h for CM and 12 h for PDB and SDB) and decreased slightly as the fungi entered the death phase. This is consistent with Gottlieb and van Etten’s finding that the level of carbohydrates was maximal at the end of the exponential phase.Citation48) The synthesis of amino acids, RNA, protein, and chitin was gradually decreased, while carbohydrates’ synthesis was continued. Therefore, the contents of carbohydrates reach maximum at the end of the exponential phaseCitation53). Carbohydrates can be utilized as a carbon source during fungal growth.Citation55) Specifically, the levels of arabitol, which is known to be the most common storage carbohydrates, increased during the exponential phase. On the other hand, myo-inositol and sucrose in biomass cultivated in CM, fructose, glucose, and maltose in PDB, and mannose, galactose, and glucose in SDB decreased during the exponential phase. Understanding the metabolites present in biomass during fungal growth is very complicated due to various pathways being involved in their synthesis, uptake from medium, and breakdown.
Most fatty acids detected in L. ramosa cultivated in CM, PDB, and SDB were retained during growth, while 3-methylpentanoic acid, arachidonic acid, (9Z,12Z)-9,12-octadecadienoic acid (linoleic acid, C18:2), and (9Z)-octadec-9-enoic acid (oleic acid, C18:1) increased and then were retained during growth. The levels of most organic acids (except for carbamic acid, butanedienoic acid, and citric acid) identified in L. ramosa cultivated in CM, PDB, and SDB increased slightly or remained constant during growth. These organic acids increased rapidly during the exponential phase and then were retained until the death phase of L. ramosa in CM, PDB, and SDB. Citric acid, which is one of the most common organic acids produced by fungi, was the major acid detected in nuruk manufactured with L. ramosa.Citation31,56)
Most major nonvolatile metabolites detected were quantitatively high in the biomass cultivated in SDB compared to those in CM and PDB.
Growth medium-dependent volatile metabolites in the extracts of L. ramosa
Various fungal volatile metabolites such as 3-octanone and 1-octen-3-ol have been detected during microbial growth,Citation36) despite microbial volatiles usually being associated with the stationary phase of this growth.Citation57,58) Therefore, in the present study, volatile metabolites isolated in the extracts including culture media and biomass of L. ramosa at 48 h were selected to compare the differences between growth media; Supplemental Table S4; see Biosci. Biotechnol. Biochem. Web site.
Volatile metabolites in the extracts of L. ramosa cultivated in CM, PDB, and SDB during 48 h were analyzed using GC–MS equipped with SBSE. The SBSE method can be used to extract fungal volatile compounds from aqueous media.Citation42,59,60) Volatile metabolites identified in the extract samples of L. ramosa cultivated in CM, PDB, and SDB at 48 h are listed in supplemental Table S4.
The following 36 volatile metabolites were identified in extracts from samples cultivated in CM: 2 acids, 10 alcohols, 2 benzenes and benzene derivatives, 1 ester, 10 furans and furan derivatives, 6 ketones, 1 sulfur-containing compound, 3 terpenes, and 1 miscellaneous. There were 27 volatile metabolites detected in extracts cultivated in PDB: 2 acids, 7 alcohols, 1 benzene or benzene derivative, 1 ester, 7 furans and furan derivatives, 6 ketones, 2 terpenes, and 1 other compound. Finally, 36 volatile metabolites were detected in the extracts from SDB: 2 acids, 9 alcohols, 2 aldehydes, 2 benzenes and benzene derivatives, 8 furans and furan derivatives, 5 ketones, 2 sulfur-containing compounds, and 2 terpenes.
The possible metabolic pathways of volatile metabolites of L. ramosa cultivated in different growth media at 48 h are shown in Fig. . The acid detected at the highest level in the extract of CM was acetic acid, while the level of ethanol was highest in the extract cultivated in CM. The level of 3-methyl-2-buten-1-ol was also dominantly determined in the extract cultivated in CM and SDB. This is an isopentenol, one of the simplest terpenoids, and is produced via the mevalonate pathway that is essential for filamentous fungi growth.Citation61–63) 3-Methyl-2-buten-1-ol provides fruity, green, and slightly lavender aroma notes.Citation63)
2-Phenylethanol was the dominant benzene or benzene derivative detected in the extracts cultivated in CM, PDB, and SDB, and was slightly more abundant in the extracts cultivated in PDB and SDB. 2-Phenylethanol is one of the fusel alcohols and is formed from phenylalanine via the Ehrlich pathway following transamination, decarboxylation, and dehydrogenation,Citation64) and is commonly detected as a major component of Korean rice wines such as makgeolli, and it provides a floral and rose aroma.Citation65–67) It is also known to be formed in diverse foods such as beer, wine, whiskey, olive oil, grapes, green and black teas, apple juice, and coffee, affecting their qualities due to its characteristic floral and rose-like odor notes.Citation68–72)
Acetic acid ethyl ester (ethyl acetate) was detected in the extracts cultivated in both CM and PDB, but not SDB, and it was more abundant in the extract of CM. It is possibly formed from acetyl-CoA and ethanol by alcohol acetyl transferase Citation73) and is the major volatile compound that contributes fruity and flower notes to the aroma of makgeolli and wine.Citation74,75)
Among furans and furan derivatives, the levels of 5-(hydroxymethyl)-2-furaldehyde and 2-furaldehyde were highest in CM. Previous studies have found that 5-(hydroxymethyl)-2-furaldehyde and 2-furaldehyde are formed from glucose and fructose via enzymatic methods and SnCl4.Citation76–80) Therefore, those compounds might be formed and excreted from L. ramosa into the medium, possibly due to the relatively high concentrations of fructose, glucose, and sucrose in CM. In particular, sucrose, which had considerably high initial content and degraded readily in the beginning of cultivation, could lead to both furan derivatives in CM.
2-Hydroxy-3-methyl-2-cyclopentene-1-one was detected in CM and PDB, but not in SBD. This compound is a cyclotene and is produced by lactic acid formed from D-fructose.Citation81) The ketone 2,3-dihydro-3,5-dihydroxy-6-methyl-4H-pyran-4-one was one of the major compounds detected in cultures in CM, PDB, and SDB. It has weak caramel and sugar-like odors and is produced by the degradation of hexose.Citation82) However, to our knowledge, there is little information on possible metabolic pathway by micro-organisms.
The terpene α-humulene, which is produced by bacteria and filamentous fungi,Citation83,84) was found in all of the media extracts and the highest in CM. α-Humulene is a sesquiterpene formed from simple five-carbon isoprene units and possibly formed via mevalonate pathway,Citation85) and it has a woody-balsamic odor note and is usually found in beer as a hop aroma.Citation86,87) Although the formations of humulene and its derivatives were elucidated in fungi,Citation83,84) its formation pathways are not fully understood. In plants, it is well known that a condensation of one molecule of dimehylallyl diphosphate with two molecules of isopentenyl diphosphate produces farnesyl diphosphate, the direct precursor of sesquiterpenes such as humulene, which is occurred by farnesyl diphosphate synthase.Citation88) As cultivation time increased, the level of terpenes increased in this study, in a similar pattern with other secondary metabolites.
The major volatile metabolites (except for 2-phenylethanol and 2-hydroxy-3-methyl-2-cyclopente-1-one) were detected at higher levels in CM compared to other growth media possibly due to relatively high level of sucrose present in CM at 0-h cultivation biomass. On the other hand, the biomass of both PDB and SDB at 0 h contained galactose dominantly.
Conclusion
This study demonstrates profiling of nonvolatile and volatile metabolites produced by L. ramosa. Also, it was shown that both nonvolatile and volatile metabolites were affected by cultivation media and times. In particular, some volatile compounds such as 5-(hydroxymethyl)2-furaldehyde and 2-furaldehyde, possibly derived from carbohydrates, were more predominantly formed in CM containing high amounts of sucrose and fructose. Also, higher levels of 2-phenylethanol and phenylmethanol were detected in L. ramosa cultivated in SDB which showed the highest content of phenylalanine. Therefore, these results indicate that some volatile metabolites produced by L. ramosa could be controlled by ingredients such as carbohydrates and amino acids present in growth media. This is the first report on a comparative analysis of nonvolatile and volatile metabolites of L. ramosa isolated from Korean traditional nuruk.
Author contributions
Hyun Chung: Data Analysis and interpretation, drafting the article.
NaKyeom Lee: Experimentation and data collection, drafting the article.
Jeong-Ah Seo: Conception and design of the work, critical revision of the article.
Young–Suk Kim: Conception and design of the work, critical revision of the article.
Final approval of the version to be published.
Disclosure statement
No potential conflict of interest was reported by the authors.
Supplemental material
The supplemental material for this paper is available at http://dx.doi.org/10.1080/09168451.2014.946390.
Funding
This work was supported by the Ministry of Agriculture, Food and Rural Affairs (Strategic Initiative for Microbiomes in Agriculture and Food) [grant number 914007-4]; the Technological innovation R&D program of SMBA [grant number S2340863].
TBBB_1256756_Supplemental_Data.zip
Download Zip (68.5 KB)References
- Alastruey-Izquierdo A, Hoffmann K, de Hoog GS, et al. Species Recognition and Clinical Relevance of the Zygomycetous Genus Lichtheimia (syn. Absidia Pro Parte, Mycocladus). Journal of Clinical Microbiology. 2010;48:2154–2170.10.1128/JCM.01744-09
- Hong SB, Kim DH, Lee MN, et al. Zygomycota associated with traditional meju, a fermented soybean starting material for soy sauce and soybean paste. Journal of Microbiology. 2012;50:386–393.10.1007/s12275-012-1437-6
- Sugar AM. Mucormycosis. Clinical Infectious Diseases. 1992;14:S126–S12910.1093/clinids/14.Supplement_1.S126
- Kutlu M, Ergin Ç, Bir F, et al. Pulmonary mucormycosis due to Lichtheimia ramosa in a patient with HIV infection. Mycopathologia. 2014;178:111–115.10.1007/s11046-014-9761-5
- Gonçalves FA, Leite RSR, Rodrigues A, et al. Isolation, identification and characterization of a novel high level β-glucosidase-producing Lichtheimia ramosa strain. Biocatalysis and Agricultural Biotechnology. 2013;2:377–384.
- Silva CAA, Lacerda MPF, Leite RSR. Production of enzymes from Lichtheimia ramosa using Brazillian savannah fruit wastes as substrate on solid state bioprocesses. Electronic Journal of Biotechnology. 2013;16:1–9.
- Garcia NFL, da Silva Santos FSS, Gonçalves FA, et al. Production of β-glucosidase on solid-state fermentation by Lichtheimia ramosa in agroindustrial residues: characterization and catalytic properties of the enzymatic extract. Electronic Journal of Biotechnology. 2015;18:314–319.10.1016/j.ejbt.2015.05.007
- Yang S, Lee J, Kwak J, et al. Fungi associated with the traditional starter cultures used for rice wine in Korea. Journal of the Korean Society for Applied Biological Chemistry. 2011;54:933–943.10.1007/BF03253183
- Kim HR, Kim JH, Bai DH, et al. Identification and characterization of useful fungi with α-amylase activity from the Korean traditional nuruk. Mycobiology. 2011;39:278–282.10.5941/MYCO.2011.39.4.278
- Song SH, Lee SH, Lee HJ, et al. Analysis of microflora profile in korean traditional nuruk. Journal of Microbiology and Biotechnology. 2013;23:40–46.10.4014/jmb
- Min JH, Kim YH, Kim JH, et al. Comparison of microbial diversity of Korean commercial Makgeolli showing high β-glucan content and high antihypertensive activity, respectively. Microbiology. 2012;40:138–141.
- Choi GI, Kim HJ, Kim HJ, et al. Changes of organic acids in Takju during storage conditions. Food Hygiene and Safety Science. 2012;27:127–132.
- Lee HH, Lee JH, Ko YJ, et al. Changes in allergenicity and quality of nuruk during fermentation. Journal of the Korean Society of Food Science and Nutrition. 2008;38:76–82.
- Kim HS, Hyun JS, Kim J, et al. Enzymological characteristics and identification of useful fungi isolated from traditional Korean nuruk. Journal of Microbiology and Biotechnology. 1998;26:456–464.
- Yu TS, Kim J, Kim HS, et al. Bibliographical study on microorganisms of traditional Korean nuruk (since 1945). Journal of the Korean Society of Food Science and Nutrition. 1998;27:789–799.
- Bae SM. The technology of Korean traditional liquor making. Seoul: Wookok Publishing Company; 2002.
- Lee TS, Choi JY. Volatile flavor components in mash of Takju prepared by using Aspergillus kawachii nuruks. Korean Journal of Food Science and Technology. 2005;30:638–643.
- Bal J, Yun SH, Song HY, et al. Mycoflora dynamics analysis of Korean traditional wheat-based nuruk. Journal of Microbiology. 2014;52:1025–1029.10.1007/s12275-014-4620-0
- Kim AJ, Choi JN, Kim J, et al. GC–MS based metabolite profiling of rice koji fermentation by various fungi. Bioscience, Biotechnology, and Biochemistry. 2010;74:100488-1–100488-6.
- Feron G, Bonnarme P, Durand A. Prospects for the microbial production of food flavours. Trends in Food Science & Technology. 1996;7:285–293.10.1016/0924-2244(96)10032-7
- So MH. Characteristics of a modified nuruk made by inoculation of traditional nuruk microorganisms. Korean Journal of Food and Nutrition. 1999;12:219–225.
- Sunesson AL, Vaes WHJ, Nilsson CA, et al. Identification of volatile metabolites from five fungal species cultivated on two media. Applied and Environmental Microbiology. 1995;62:2911–2918.
- Verdonk JC, Ric de Vos CR, Verhoeven HA, et al. Regulation of floral scent production in petunia revealed by targeted metabolomics. Phytochemistry. 2003;62:997–1008.10.1016/S0031-9422(02)00707-0
- Dunn WB, Ellis DI. Metabolomics: current analytical platforms and methodologies. Trends in Analytical Chemistry. 2005;24:285–294.
- PONNUSAMY K, LEE S, LEE CH. Time-dependent correlation of the microbial community and the metabolomics of traditional barley nuruk starter fermentation. Bioscience, Biotechnology, and Biochemistry. 2013;77:683–690.10.1271/bbb.120665
- Kim HR, Kim JH, Ahn BH, et al. Metabolite Profiling during Fermentation of Makgeolli by the Wild Yeast Strain Saccharomyces cerevisiae Y98-5. Mycobiology. 2014;42:353–360.10.5941/MYCO.2014.42.4.353
- Kum SJ, Yang SO, Lee SM, et al. Effects of Aspergillus Species Inoculation and Their Enzymatic Activities on the Formation of Volatile Components in Fermented Soybean Paste (doenjang). Journal of Agricultural and Food Chemistry. 2015;63:1401–1418.10.1021/jf5056002
- Kim J, Choi JN, John KM, et al. GC–TOF-MS- and CE–TOF-MS-Based Metabolic Profiling of Cheonggukjang (Fast-Fermented Bean Paste) during Fermentation and Its Correlation with Metabolic Pathways. Journal of Agricultural and Food Chemistry. 2012;60:9746–9753.10.1021/jf302833y
- Lenz EM, Wilson ID. Analytical Strategies in Metabonomics. Journal of Proteome Research. 2007;6:443–458.10.1021/pr0605217
- Ding MZ, Cheng JS, Xiao WH, et al. Comparative metabolomic analysis on industrial continuous and batch ethanol fermentation processes by GC–TOF-MS. Metabolomics. 2009;5:229–238.10.1007/s11306-008-0145-z
- Gadd GM. Fungal production of citric and oxalic acid: importance in metal speciation, physiology and biogeochemical processes. Advances in Microbial Physiology. 1999;41:47–92.10.1016/S0065-2911(08)60165-4
- Frisvad JC, Larsen TO, de Vries R, et al. Secondary metabolite profiling, growth profiles and other tools for species recognition and important Aspergillus mycotoxins. Studies in Mycology. 2007;59:31–37.10.3114/sim.2007.59.04
- Kaminski E, Stawicki S, Wasowicz E. Volatile flavor compounds produced by molds of Aspergillus, Penicillium, and Fungi imperfecti. Applied Microbiology. 1974;27:1001–1004.
- Bramorski A, Christen P, Ramirez M, et al. Production of volatile compounds by the edible fungus Rhizopus oryzae during solid state cultivation on tropical agro-industrial substrates. Biotechnology Letters. 1998;20:359–362.10.1023/A:1005323229125
- Jonsson P, Johansson AI, Gullberg J, et al. High-throughput data analysis for detecting and identifying differences between samples in GC/MS-based metabolomic analyses. Analytical Chemistry. 2005;77:5635–5642.10.1021/ac050601e
- Stoppacher N, Kluger B, Zeilinger S, et al. Identification and profiling of volatile metabolites of the biocontrol fungus Trichoderma atroviride by HS-SPME-GC–MS. Journal of Microbiological Methods. 2010;81:187–193.10.1016/j.mimet.2010.03.011
- Buchholz A, Takors R, Wandrey C, et al. Quantification of Intracellular Metabolites in Escherichia coli K12 Using Liquid Chromatographic-Electrospray Ionization Tandem Mass Spectrometric Techniques. Analytical Biochemistry. 2001;295:129–137.10.1006/abio.2001.5183
- Soga T, Ohashi Y, Ueno Y, et al. Quantitative Metabolome Analysis Using Capillary Electrophoresis Mass Spectrometry. Journal of Proteome Research. 2003;2:488–494.10.1021/pr034020m
- Hollywood K, Brison DR, Goodacre R. Metabolomics: Current technologies and future trends. PROTEOMICS. 2006;6:4716–4723.10.1002/(ISSN)1615-9861
- Pinches SE, Apps P. Production in food of 1,3-pentadiene and styrene by Trichoderma species. International Journal of Food Microbiology. 2007;116:182–185.10.1016/j.ijfoodmicro.2006.12.001
- Hung R, Lee S, Bennett JW. Fungal volatile organic compounds and their role in ecosystems. Applied Microbiology and Biotechnology. 2015;99(8):3395–3405.10.1007/s00253-015-6494-4
- Prieto A, Basauri O, Rodil R, et al. Stir-bar sorptive extraction: a view on method optimisation, novel applications, limitations and potential solutions. Journal of Chromatography A. 2010;1217:2642–2666.10.1016/j.chroma.2009.12.051
- Keatkrai Jeesuda, Jirapakkul W. Volatile profile of khanom jeen, Thai fermented rice noodles, and the changes during the fermentation process. Spinal Cord Injury Asia. 2010;36:46–51.10.2306/scienceasia1513-1874.2010.36.046
- Kang BS, Lee JE, Park HJ. Qualitative and quantitative prediction of volatile compounds from initial amino acid profiles in Korean rice wine (makgeolli) model. Journal of Food Science. 2014;79:C1106–C1116.10.1111/jfds.2014.79.issue-6
- Kim S, Lee DY, Wohlgemuth G, et al. Evaluation and Optimization of Metabolome Sample Preparation Methods for Saccharomyces cerevisiae. Analytical Chemistry. 2013;85:2169–2176.10.1021/ac302881e
- Woo PCY, Leung SY, Ngan AHY, et al. A significant number of reported Absidia corymbifera (Lichtheimia corymbifera) infections are caused by Lichtheimia ramosa (syn. Lichtheimia hongkongensis): an emerging cause of mucormycosis. Emerging Microbes and Infections. 2012;1:1–8.
- de Andrade Silva CAA, Lacerda MPF, Leite RSR, et al. Physiology of Lichtheimia ramosa obtained by solid-state bioprocess using fruit wastes as substrate. Bioprocess and Biosystems Engineering. 2014;37:727–734.10.1007/s00449-013-1043-y
- Gottlieb D, Van Etten JL. Biochemical changes during the growth of fungi. I. Nitrogen compounds and carbohydrate changes in Penicillium atrovenetum. Journal of Bacteriology. 1964;88:114–121.
- Eagle H. Amino Acid Metabolism in Mammalian Cell Cultures. Science. 1959;130:432–437.10.1126/science.130.3373.432
- Korpi A, Järnberg J, Pasanen AL. Microbial Volatile Organic Compounds. Critical Reviews in Toxicology. 2009;39(2):139–193.10.1080/10408440802291497
- Ravasio D. Major contribution of the Ehrlich pathway for 2-phenylethanol/rose flavor production in Ashbya gossypii. FEMS Yeast Research . 2014;14(6):833–844.10.1111/fyr.2014.14.issue-6
- Hazelwood LA, Daran JM, van Maris AJ, et al. The Ehrlich Pathway for Fusel Alcohol Production: a Century of Research on Saccharomyces cerevisiae Metabolism. Applied and Environmental Microbiology. 2008;74:2259–2266.10.1128/AEM.02625-07
- DAGLEY S, DAWES E, MORRISON G. Production of Amino-acids in Synthetic Media by Escherichia coli and Aerobacter aerogenes. Nature. 1950;165:437–438.10.1038/165437a0
- Morton AG, Broadbent D. The Formation of Extracellular Nitrogen Compounds by Fungi. Journal of General Microbiology. 1955;12:248–258.10.1099/00221287-12-2-248
- Bidochika MJ, Low NH, Khachatourians GG. Carbohydrate storage in the entomopathogenic fungus Beauveria bassiana. Applied and Environmental Microbiology. 1990;56(10):3186–3190.
- Im SY, Baek CH, Baek SY, et al. Quality characteristics of Takju according to different rice varieties and mixing ratio of Nuruk. Korean Journal of Food Preservation. 2014;21(6):892–902.10.11002/kjfp.2014.21.6.892
- Robinson T, Singh D, Nigam P. Solid-state fermentation: a promising microbial technology for secondary metabolite production. Applied Microbiology and Biotechnology. 2001;55:284–289.10.1007/s002530000565
- Weinberg ED. Biosynthesis of secondary metabolites: roles of trace metals. In: Rose AH, Wilkinson JF, editors. Advances in Microbial Physiology. London: Academic Press; 1970. p. 6.
- Müller A, Faubert P, Hagen M, et al. Volatile profiles of fungi-chemotyping of species and ecological functions. Fungal Genetics and Biology. 2013;54:25–33.10.1016/j.fgb.2013.02.005
- Bazemore RA, Feng J, Cseke L, et al. Biomedically important pathogenic fungi detection with volatile biomarkers. Journal of Breath Research. 2012;6:1–11.
- Withers ST, Gottlieb SS, Lieu B, et al. Identification of Isopentenol Biosynthetic Genes from Bacillus subtilis by a Screening Method Based on Isoprenoid Precursor Toxicity. Applied and Environmental Microbiology. 2007;73:6277–6283.10.1128/AEM.00861-07
- Buhaescu I, Izzedine H. Mevalonate pathway: A review of clinical and therapeutical implications. Clinical Biochemistry. 2007;40:575–584.10.1016/j.clinbiochem.2007.03.016
- McGinty D, Jones L, Letizia CS, et al. Fragrance material review on 3-methyl-2-buten-1-ol. Food and Chemical Toxicology. 2010;48:S64–S69.10.1016/j.fct.2009.11.013
- Etschmann M, Bluemke W, Sell D, et al. Biotechnological production of 2-phenylethanol. Journal of Applied Microbiology and Biotechnology. 2002;59:1–8.
- Han EH, Lee TS, Noh BS, et al. Volatile flavor components in mash of takju prepared by using different nuruks. Korean Journal of Food Science and Technology. 1997;29:563–570.
- Park HJ, Lee SM, Song SH, et al. Characterization of volatile components in makgeolli, a traditional Korean rice wine, with or without pasteurization, during storage. Molecules. 2013;18:5317–5325.10.3390/molecules18055317
- Jung H, Lee SJ, Lim JH, et al. Chemical and sensory profiles of makgeolli, Korean commercial rice wine, from descriptive, chemical, and volatile compound analyses. Food Chemistry. 2014;152:624–632.10.1016/j.foodchem.2013.11.127
- Szlavko CM. Tryptophol, tyrosol and phenylethanol-the aromatic higher alcohols in beer. Journal of the Institute of Brewing. 1973;79:283–288.10.1002/jib.1973.79.issue-4
- Yonezawa T, Fushiki T. Testing for taste and flavour of beer. In: Jackson JF, Linskens HF, editors. Anal Taste Aroma. Berlin: Springer; 2002. p. 29–45.10.1007/978-3-662-04857-3
- Kim HR, Jo SJ, Lee SJ, et al. Physicochemical and sensory characterization of a Korean traditional rice wine prepared from different ingredients. Korean Journal of Food Science and Technology. 2008;40:551–557.
- Kim HR, Kim JH, Bae DH, et al. Characterization of yakju brewed from glutinous rice and wild-type yeast strains isolated from nuruks. Journal of Microbiology and Biotechnology. 2010;20:1702–1720.
- Sake Kiyoshi Y. Production and flavor. Food Reviews International. 1999;15:83–107.
- Bartowsky EJ. Bacterial spoilage of wine and approaches to minimize it. Letters in Applied Microbiology. 2009;48:149–156.10.1111/lam.2009.48.issue-2
- So MH, Lee YS, Han SH, et al. Analysis of major flavor compounds in Takju mash brewed with a modified Nuruk. Journal of the Korean Society of Food Science and Nutrition. 1999;12:421–426.
- Lambrechts MG, Pretorius IS. Yeast and its importance to wine aroma: a review. South African Journal of Enology and Viticulture. 2000;21:97–129.
- Huag R, Qi W, Su R, et al. Integrating enzymatic and acid catalysis to convert glucose into 5-hydroxymethylfurfural. Chemical Communications. 2010;46:1115–1117.
- Hu S, Zhang Z, Song J, et al. Efficient conversion of glucose into 5-hydroxymethylfurfural catalyzed by a common Lewis acid SnCl4 in an ionic liquid. Green Chemistry. 2009;11:1746–1749.10.1039/b914601f
- Yong G, Zhang Y, Ying JY. Efficient catalytic system for the selective production of 5-hydroxymethylfurfural from glucose and fructose. Angewandte Chemie. 2008;120:9485–9488.10.1002/ange.v120:48
- Chheda JN, Román-Leshkov Y, Dumesic JA. Production of 5-hydroxymethylfurfural and furfural by dehydration of biomass-derived mono-and poly-saccharides. Green Chemistry. 2007;9:342–350.10.1039/B611568C
- Choudhary V, Mushrif SH, Ho C, et al. Insights into the interplay of Lewis and Brønsted acid catalysts in glucose and fructose conversion to 5-(Hydroxymethyl)furfural and levulinic acid in aqueous media. Journal of the American Chemical Society. 2013;135:3997–4006.10.1021/ja3122763
- Baltes W, Mevissen L. Model reactions on roast aroma formation 6. Volatile reaction-products from the reaction of phenylalanine with glucose during cooking and roasting. European Food Research and Technology. 1988;187:209–214.
- Shaw PE, Tatum JH, Berry RE, et al. 2,3-Dihydro-3, 5-dihydroxy-6-methyl-4H-pyran-4-one, a degradation product of a hexose. Carbohydrate Research. 1971;16:207–211.10.1016/S0008-6215(00)86115-7
- Zeringue HJ, Bhatnagar D, Cleeland TE. Volatile compounds unique to aflatoxigenic strains of Aspergillus flavus. Applied and Environmental Microbiology. 1993;59:2264–2270.
- Wilkins K, Larsen K, Simkus M. Volatile metabolites from mold growth on building materials and synthetic media. Chemosphere. 2000;41:437–446.10.1016/S0045-6535(99)00273-8
- Crespo R, Pedrini N, Juárez MP, et al. Volatile organic compounds released by the entomopathogenic fungus Beauveria bassiana. Microbiological Research. 2008;163:148–151.10.1016/j.micres.2006.03.013
- Zhang H, Qiu M, Chen Y, et al. Plant terpenes: phytochemistry and pharmacognosy. Encyclopedia of life support systems (Eolss). Oxford: Eolss; 2011. p. 1–9.
- Peackock VE, Deinzer ML. Chemistry of hop aroma in beer. Journal of the American Society of Brewing Chemists. 1981;39:0316.
- Markus B. L, Amirhossein A. Metabolic engineering of plant monoterpenes, sesquiterpenes and diterpenes-current status and future opportunities. Plant Biotechnology Journal. 2013;11:169–196.