Abstract
In this study, decomposition products of glycidyl palmitate (GP) of fatty acids heated at high temperature such as deep frying were investigated. When GP and tripalmitin (TP) were heated at 180 and 200 °C, they were decreased with heating time. The weight of GP was less than that of TP, although both GP and TP were converted to polar compounds after heating. The decomposition rate of GP was higher than TP. Both GP and TP produced considerable amounts of hydrocarbons and aldehydes during heating. Aldehydes produced from GP and TP included saturated aldehydes with carbon chain length of 3–10, while hydrocarbons consisted of carbon chain length of 8–15. It was observed that major hydrocarbons produced from GP during heating were pentadecane. Moreover, the level of carbon dioxide (CO2) released from GP was higher than that of TP. It was suggested that fatty acids in GE might be susceptible to decarboxylation. From these results, GP might be quickly decomposed to hydrocarbons, aldehydes and CO2 besides polar compounds by heating, in comparison with TP.
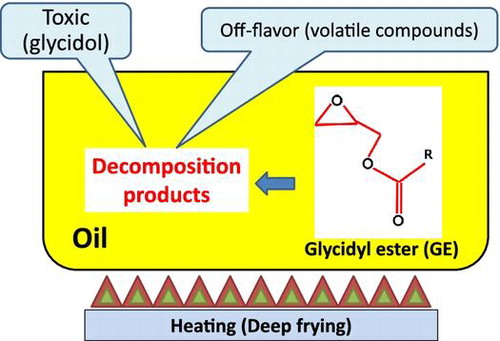
Decomposition products of glycidyl ester of fatty acids may affect flavor and nutritional value of fried foods?
The presence of glycidyl esters (GE) of fatty acids in vegetable oils was reported as the result of research to investigate the origin of 3-monochloro-1,2-propanediol (3-MCPD) and 3-MCPD esters of fatty acids in these oils.Citation1–4) In their work, GE was detected as the precursors to these compounds. Weißhaar and PerzCitation5) reported the existence of relatively high levels of GE of fatty acids in some vegetable oils. When Masukawa et al.Citation6) performed a survey of commercial oils sold in Japan they reported that GE was detected in every sample tested. Contents of GE in a variety of vegetable oils were correlated to the diacylglycerol content in the oil.Citation7) Especially, palm oil was relatively rich in GE, because it contained considerable amounts of diacylglycerol. Therefore, it has been thought that mono- and di-acylglycerols may be the precursors of GE formed by an intermolecular mechanism under the conditions of high-temperature refining.Citation8) Moreover, the composition of GEs was reflected by the fatty acid composition of vegetable oils. The ratio of glycidyl oleate was higher in rapeseed oil, whereas that of glycidyl oleate and glycidyl palmitate (GP) was higher in palm oil. These observations supported the mechanism that GE could be formed from mono- and di-acylglycerols in vegetable oils.
These studies led the Federal Institute for Risk Assessment (BfR), a scientific agency of the Federal Republic of Germany, to state its opinion regarding the necessity for a method to quantify GE in edible oils to provide reliable risk assessment.Citation9) Toxicological investigations of GE are not available yet. However, the International Agency for Research on CancerCitation10) classified glycidol as probably carcinogenic to humans, although it is unclear to what extent glycidol is split off from ester-bound glycidol in the human body.Citation11)
Many researchers have developed the method to determine the composition of GE in edible fats and oils and oil foods using LC–MS and GC–MS.Citation12–16) These methods could be useful for investigating the metabolism such as digestion and absorption of GE in experimental animal and human after intake of fats and oils contaminated with GE. On the other hand, the behavior of GE contaminated in vegetable oils during heat cooking is unclear. During deep frying, a considerable portion of the frying medium may be absorbed by the food. GE may be present in any oil foods that contain refined vegetable oils and fats. However, there is a lack of research on changes in the content of GE during frying, although there have been reports of GE in a variety of refined oils. Aniolowska and KitaCitation17) investigated the content of GE in some vegetable oils during frying of French fries. They reported that GE present in vegetable oils could be transferred to fried foods during deep frying because the presence of GE was recognized in French fries besides vegetable oils. They also reported that contents of GE were remarkably reduced in vegetable oils and French fries during heating. However, they did not give the information concerning with decomposition products of GE during deep frying. Decomposition products of GE contaminated in edible oils by deep frying may affect the flavor and nutritional value of fried foods.
In this study, we investigated decomposition products from GP by heating when GP was used as a typical GE, and discussed the chemical reaction of GE during heating at high temperature such as deep frying.
Materials and methods
Materials. Tripalmitin (TP), glycidyl palmitate (GP), and glycidyl myristate were purchased from Wako Pure Chemicals Industries (Osaka, Japan). Their purities were more than 98%. Standard aldehydes (propanal, butanal, pentanal, hexanal, heptanal, octanal, nonanal, and decanal) and hydrocarbons (octane, nonane, decane, undecane, dodecane, tridecane, tetradecane, pentadecane, and hexadecane) were purchased from Wako Pure Chemical Industries (Osaka, Japan).
Heating test. GP or TP (500 mg) was put in a 20 mL- or 40 mL-test tube sealed with a silicon rubber cap. They were heated at 180 or 200 °C with a block heater. Head space gas (1.0 mL) was collected with a gas tight syringe after heating and supplied to gas liquid chromatography (GLC).
Volatile compounds in head space were analyzed by GLC with a flame ionization detector (FID), equipped with a CP-SIL 88 (60 m × 0.25 mm, 0.25 μm, Varian), using nitrogen gas as a carrier at flow rate of 1.0 mL/min. The program of oven temperature was as follows; the temperature kept at 40 °C for 5 min, then raised to 140 °C at the rate of 5 °C/min, further raised to 225 °C at the rate of 10 °C/min, and held at 225 °C for 15 min. The temperature of the injector and the FID detector were set at 225 °C. The split ratio was 20:1. The peak assignment was performed by authentic standards and GC–MS using Shimadzu Parvum2 Gas Chromatograph Mass Spectrometer connected with a Inter Cap 5MS/Sil column (30 m × 0.25 mm, 0.25 μm).
Carbon dioxide (CO2) in head space was determined with a Gastec gas sampling pump set GV-110S using a detector tube 2LC (Gastec Corp., Japan).
Moreover, heated GP and TP were stile solid and weighed before the lipid composition was estimated by thin layer chromatography with FID (TLC-FID) using a Chromatrod-SIII and an Iatroscan MK-6s equipment (Mitsubishi Kagaku Iatron Inc., Japan). The solvent system consisted of n-hexane/diethyl ether/formic acid (80:30:1, by vol).
Data show the mean and standard deviation of triplicate.
Results
When GP and TP were heated at 180 and 200 °C, the changes in weights of GP and TP during heating are shown in Fig. . The weight of GP and TP was slightly reduced (below 5%) during heating at 180 °C, although the weight of the former was lower than that of the latter. The weight of solid GP was markedly reduced during heating at 200 °C, in comparing with that of solid TP. The weight of GP after heating for 8 h was reduced to 83% of unheated one, whereas that of TP was reduced to 95%. These results showed that GP could be quickly decomposed and volatized by heating at high temperature in comparison with TP, although residual GP was still solid.
Fig. 1. Changes in the weight of glycidyl palmitate (GP) and tripalmitin (TP) during heating at 180 and 200 °C. ● GP (200 °C), ▴ GP (180 °C), ■ TP (200 °C), and ♦ TP (180 °C). Data show the mean and standard deviation of triplicate.
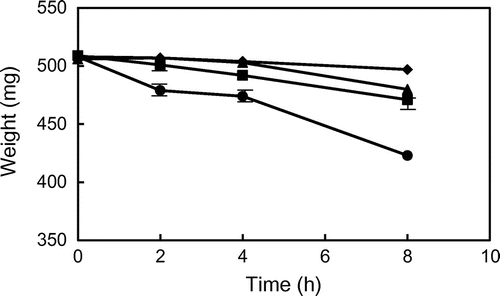
Fig. shows the chromatogram of TLC-FID analysis for GP and TP after heating at 180 °C. Both GP and TP were decomposed and converted to polar compounds (PC) by heating. Similar observations were obtained for heating at 200 °C (data not shown). Nature GP was not almost detected after heating for 8 h, while some of TP remained. However, the hydrolysis of the ester bond in GP was not recognized during heating because glycidol and free fatty acids were not almost detected in GP after heating. Thus, PC formed from GP seemed to be polymers and other compounds than hydrolyzed compounds.
Fig. 2. TLC-FID chromatograms of GP and TP after heating at 180 °C. A TP (2 h), B TP (4 h), C TP (8 h), D GP (2 h), E GP (4 h), and F GP (8 h). GP, glycidyl palmitate; TP, tripalmitin; PC, polar compounds.
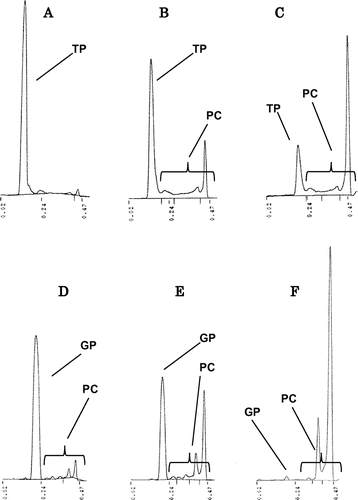
Fig. shows the changes in the relative ratio of GP, TP, and PC during heating at 180 and 200 °C. The relative ratio of GP, TP, and PC was described as the ratio of individual peak area to total peak areas on the chromatogram. GP quickly disappeared than TP during heating at 180 and 200 °C. GP was not almost detected after heating for 8 h, and most of GP were converted to PC. On the other hand, TP remained by 27 and 14% after 8 h-heating at 180 and 200 °C, respectively, although most of TP were converted to PC. These results suggested that GE could be polymerized by heating at high temperature. The gel permeation column chromatography showed the existence of peaks eluting prior to GP (data not shown). Probably, they must be higher molecular weight compounds than GP and may be corresponded to PC.
Fig. 3. Changes in the relative ratio of GP, TP, and polar compounds (PC) to total peak areas during heating at 180 and 200 °C. ● GP (200 °C), ▴ GP (180 °C), ■ TP (200 °C), and ♦ TP (180 °C). Data shows the mean and standard deviation of triplicate.
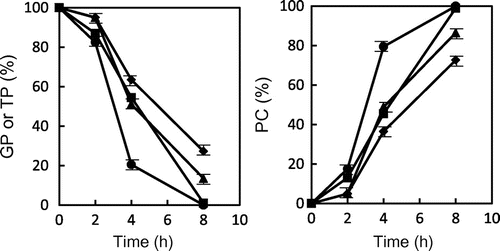
We investigated decomposition products, especially volatile compounds from PG during heating, to know the reason of the weight loss of GP during heating at high temperature. Fig. shows typical gas chromatograms of head space gas of TP and GP after heating at 200 °C for 2 h. Many kinds of peaks were observed on gas chromatograms of TP and GP after heating. A similar chromatogram was obtained for TP and GP heated at 180 °C (data not shown). Most of volatile compounds were identified as hydrocarbons and aldehydes by GC–MS and authentic standards. GC–MS analysis demonstrated the occurrence of hydrocarbons with carbon chain length of 8–15 and saturated aldehydes with carbon chain length of 3–10. There was no difference in varieties of volatile compounds in between heated GP and TP. Saturated aldehydes included propanal, butanal, pentanal, hexanal, heptanal, octanal, nonanal, and decanal. On the other hand, hydrocarbons included octane, nonane, decane, undecane, dodecane, tridecane, tetradecane, and pentadecane. It was observed that the peak corresponding to pentadecane was much bigger in GP after heating at 200 and 180 °C, although hydrocarbons and aldehydes were mainly formed via hydroperoxides produced by thermal oxidation of fatty acids. A similar observation was obtained for heated glycidyl myristate. The peak corresponding to tridecane was much bigger in heated glycidyl myristate (Fig. ). That is, the hydrocarbon that one carbon atom was removed from the fatty acid in GE could be formed by heating at high temperature. These observations suggested that the decarboxylation of fatty acids occurred in GE by heating at high temperature.
Fig. 4. Gas chromatograms of head space gas from TP, GP, and glycidyl myristate after heating at 200 °C for 2 h.
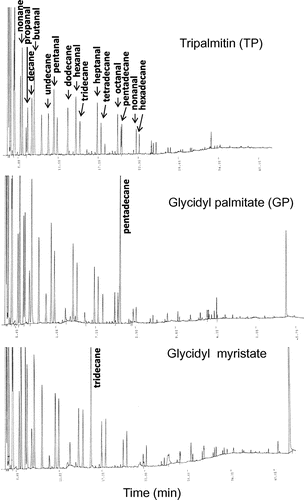
Fig. shows the changes in total contents of saturated aldehydes with carbon chain length of 3–10 from GP and TP during heating at 180 and 200 °C. Total contents of saturated aldehydes were increased with heating time. During heating of GP and TP at 200 °C, total contents of saturated aldehydes were significantly higher than those at 180 °C. In particular, total contents of saturated aldehydes from GP heated at 200 and 180 °C for 8 h were three times and twice as high as those from TP, respectively. The former was significantly higher than those from the latter. More amounts of aldehydes formed from GE such as GP may affect the flavor and nutritional value of fried foods as well as frying oil.
Fig. 5. Changes in the contents of saturated aldehydes from GP and TP during heating at 180 and 200 °C. ● GP (200 °C), ▴ GP (180 °C), ■ TP (200 °C), and ♦ TP (180 °C). Data show the mean and standard deviation of triplicate.
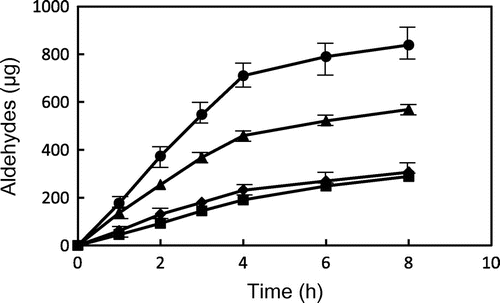
Fig. shows the changes in total contents of hydrocarbons with carbon chain length of 9–15 from GP and TP during heating at 180 and 200 °C. Among hydrocarbons, octane could not be determined because the peak of octane overlapped with other compounds. Total contents of hydrocarbons were also increased with heating time. During heating at 180 and 200 °C, total contents of hydrocarbons from GP were much higher than those from TP. In particular, GP produced twice amounts of hydrocarbons than TP during heating at 200 °C for 8 h. These results suggested the remarkable weight loss of GP during heating might come from the formation of hydrocarbons as well as aldehydes. Moreover, these decomposition products of GE may affect the quality of frying oil and fried foods, although GE is trace in edible oils.
Fig. 6. Changes in the contents of hydrocarbons from GP and TP during heating at 180 and 200 °C. ● GP (200 °C), ▴ GP (180 °C), ■ TP (200 °C), and ♦ TP (180 °C). Data show the mean and standard deviation of triplicate.
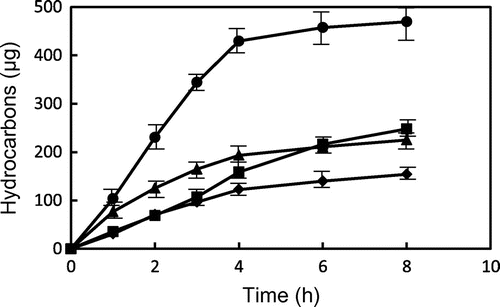
Fig. shows the changes in the relative ratio of pentadecane to peak area of total hydrocarbons produced from GP and TP during heating at 180 and 200 °C. The relative ratio of pentadecane to peak area of total hydrocarbons from TP was the range of 10–15%, and it did not depend on heating temperature and time. On the other hand, the ratio of pentadecane in hydrocarbons from GP was more than 20% after heating at 180 °C, and it reached to more than 50% after 4 h-heated at 180 °C. These observations showed that pentadecane was preferentially formed from palmitic acid esterified with glycidol by decarboxylation. Hydrocarbons could be formed by not only the oxidation but also the decarboxylation of fatty acids in GE during heating at high temperature because the high ratio of pentadecane and tridecane to total hydrocarbons was not observed for TP and trimyristin during heating, respectively. The relative ratio of pentadecane in GP heated at 200 °C was also higher than that in TP, although it was 40% at maximum. That is the reason why the production of hydrocarbons other than pentadecane could be also increased at 200 °C.
Fig. 7. Changes in the relative ratio of pentadecane to peak areas of total hydrocarbons from GP and TP during heating at 180 and 200 °C. ● GP (200 °C), ▴ GP (180 °C), ■ TP (200 °C), and ♦ TP (180 °C). Data show the mean and standard deviation of triplicate.
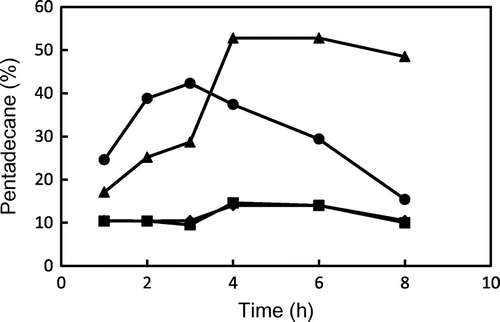
We also determined the level of carbon dioxide (CO2) released from GP and TP during heating, because pentadecane could be formed by decarboxylation of palmitic acid. Fig. shows the changes in the level of CO2 from GP and TP during heating at 200 °C. Although the CO2 level in the head space of GP and TP were increased with heating time, the CO2 level in the head space of GP was higher than that of TP during heating. In particular, approximately 9000 ppm of CO2 was observed in GP after 8 h-heating. These observations were consistent with the high level of pentadecane in head space of PG during heating. Generally, hydrocarbons may come from fatty acids via hydroperoxides by oxidation. However, these observations demonstrated that fatty acids esterified with glycidol could be susceptible to decarboxylation and result in the formation of hydrocarbon.
Discussion
Based on these results, we discuss the behavior of GE during heating at high temperature. Fig. shows conjectured mechanism for the formation of aldehydes, hydrocarbons, and polar compounds (polymers) from GE during heating at high temperature. Fatty acids in GE are exposed to thermal oxidation and transformed to hydroperoxides as well as those in triacylglycerols (TAG). Hydroperoxides are unstable at high temperature and easily decomposed to aldehydes and hydrocarbons. On the other hand, hydroperoxides are also polymerized. These reactions of GE are basically same as those of TAG.Citation18) Thermal oxidation like this is particularly remarkable for unsaturated fatty acids. However, there is difference in the chemical reaction of fatty acids in between GE and TAG during heating at high temperature. GE may be susceptible to the elimination or cleavage of epoxy group by heating. After that, the decarboxylation may immediately occur for fatty acid, and then alkyl radical (R・) may be formed. Alkyl radical is so reactive that it may denote hydrogen atom from another fatty acid (LH) to form hydrocarbon (RH). Alkyl radical also may react with another lipid radical (L・) and form polymers (LR). The chain reaction like this may occur for GE. That is the reason why GE could be unstable in comparison with TAG during heating at high temperature.
Fig. 9. Conjectured mechanism for the formation of hydrocarbons, aldehydes, and polar compounds (polymers) from glycidyl esters (GEs) during heating at high temperature.
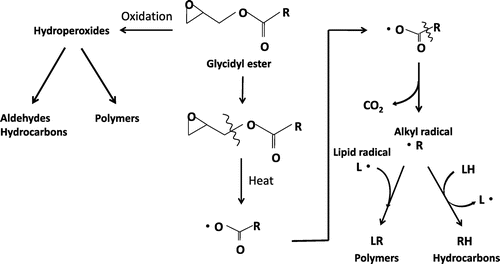
Aniolowska and KitaCitation17) found that GE contaminated in vegetable oils was transferred to French fries during deep frying, and that their GE was markedly decreased with frying time. Our results were very similar to their ones. We assume that GE present in vegetable oils and French fries could be thermally unstable and easily decomposed to aldehydes, hydrocarbons, and polar compounds including polymers. Moreover, they found that glycidyl oleate in vegetable oils and French fries showed the most reduce among GE during deep frying. Probably glycidyl oleate could be more susceptible to thermal oxidation besides decarboxylation than GP. Our hypothesis may explain the thermal instability of GE contaminated in edible oils and fried foods.
We think that aldehydes and hydrocarbons produced from GE during heating at high temperature, as shown in Fig. may affect the sensory quality of frying oils and fried foods. However, their nutritional effects (toxicity) are negligible because GE is trace in edible vegetable oilsCitation5–7) and PC produced from GE during heating could not be absorbed in human.
Conclusion
We investigated decomposition products from GP and TP heated at high temperature. Although GP and TP were decomposed to aldehydes, hydrocarbons, and polar compounds by heating at 200 and 180 °C, the decomposition rate of GP was much higher than TP. Palmitic acid in glycidyl ester was susceptible to decarboxylation and it easily formed pentadecane in comparison with TP. From these results, it was shown that GE was more unstable than TAG at high temperature. Aldehydes, hydrocarbons, and polymers produced from GE during heating may affect the sensory quality of frying oil and fried foods but not nutritional value, because GE is trace in edible vegetable oils.
Author contribution
Wataru KIMURA, Analysis and interpretation of data.
Yasushi ENDO, Critical revision of the article, and conception and design of the study.
Disclosure statement
No potential conflict of interest was reported by the authors.
References
- Zelinkova′ Z, Svejkovska′ B, Velı′sˇek J, et al. Fatty acid esters of 3-chloropropane-1,2-diol in edible oils. Food Addit Contam. 2006;23:1290–1298.10.1080/02652030600887628
- Weißhaar R. Determination of total 3-chloropropane-1,2-diol (3-MCPD) in edible oils by cleavage of MCPD esters with sodium methoxide. Eur J Lipid Sci Technol. 2008;110:183–186.10.1002/(ISSN)1438-9312
- Weißhaar R. 3-MCPD-esters in edible fats and oils – a new and worldwide problem. Eur J Lipid Sci Technol. 2008;110:671–672.10.1002/ejlt.v110:8
- International Life Sciences Institute. 3-MCPD esters in food products. Summary report of a workshop held in February 2009 in Brussels. Belgium; 2009.
- Weißhaar R, Perz R. Fatty acid esters of glycidol in refined fats and oils. Eur J Lipid Sci Technol. 2010;112:158–165.10.1002/ejlt.v112:2
- Masukawa Y, Shiro H, Nakamura S, et al. A new analytical method for the quantification of glycidol fatty acid esters in edible oils. J Oleo Sci. 2010;59:81–88.10.5650/jos.59.81
- Haines T, Adlaf K, Pierceall R, et al. Direct determination of MCPD fatty acid esters and glycidyl fatty acid esters in vegetable oils by LC–TOFMS. J Am Oil Chem Soc. 2011;88:1–14.10.1007/s11746-010-1732-5
- CG.Hamlet, LAsuncion, JVelišeket al. The occurrence of fatty acid esters of chloropropanediols in foods: a review prepared for the UK Food Standards Agency; 2010. https://www.food.gov.uk/sites/default/files/C04072_Literature%20Review_Final_081010.pdf.
- Federal Institute for Risk Assessment (BfR). Initial evaluation of the assessment of levels of glycidol fatty acid esters detected in refined vegetable fats (in German). BfR Opinion No. 007/2009, 10 March; 2009. http://www.bfr.bund.de/cm/208/erste_einschaetzung_von_glycidol_fettsaeureestern.pdf.
- World Health Organization International Agency for Research. Glycidol. In: IARC monographs on the evaluation of carcinogenic risks to humans. Glycidol. Lyon: IARC; 2000. p. 469–485.
- Bakhiya N, Abraham K, Gurtler R, et al. Toxicological assessment of 3-chloropropane-1,2-diol and glycidol fatty acid esters in food. Mol Nutr Food Res. 2011;55:509–521.10.1002/mnfr.v55.4
- Masukawa Y, Shiro H, Kondo N, et al. Generalized method to quantify glycidol fatty acid esters in edible oils. J Am Oil Chem Soc. 2011;88:15–21.10.1007/s11746-010-1645-3
- Katsuragi Y, Kibune N, Kawai S. Development of direct determination method of glycidyl fatty acid esters – summary of joint AOCS/JOCS collaborative study – (in Japanese). Oleoscience. 2012;12:573–580.10.5650/oleoscience.12.573
- Miyazaki K, Koyama K, Sasako H, et al. Indirect method for simultaneous determinations of 3-chloro-1,2-propandiol fatty acid esters and glycidyl fatty acid esters. J Am Oil Chem Soc. 2012;89:1403–1407.
- Blumhorst MR, Collison MW, Cartil R, et al. Collaborative study for the analysis of glycidyl fatty acid esters in edible oils using LC–MS. J Am Oil Chem Soc. 2013;90:493–500.10.1007/s11746-012-2187-7
- MacMahon S, Begley TH, Diachenko GW. Occurrence of 3-MCPD and glycidyl esters in edible oils in the United States. Food Addit Contanm. 2013;30:2081–2092.10.1080/19440049.2013.840805
- Aniolowska M, Kita A. The effect of type of oil and degree of degradation on glycidyl esters content during the frying of french fries. J Am Oil Chem Soc. 2015;92:1621–1631.10.1007/s11746-015-2715-3
- Endo Y. Deterioration of edible oils. In: Totani and Hara editors. Oil science. Tokyo: Asakura; 2015. p. 145–173.