Abstract
The intestinal tract comes into direct contact with the external environment despite being inside the body. Intestinal epithelial cells, which line the inner face of the intestinal tract, have various important functions, including absorption of food substances, immune functions such as cytokine secretion, and barrier function against xenobiotics by means of detoxification enzymes. It is likely that the functions of intestinal epithelial cells are regulated or modulated by these components because they are frequently exposed to food components at high concentrations. This review summarizes our research on the interaction between intestinal epithelial cells and food components at cellular and molecular levels. The influence of xenobiotic contamination in foods on the cellular function of intestinal epithelial cells is also described in this review.
Graphical abstract
Relationship between various food components and intestinal epithelial cells.
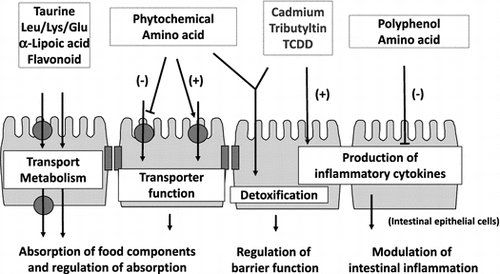
The intestinal tract is an organ with a large surface area that comes into direct contact with the external environment despite being inside the body. In humans, the small intestine, the site of absorption of food components is approximately 6 m-long and has 0.5–1.5-mm-long villi on its inner wall. Each villus is covered by a sheet of intestinal epithelial cells, and there are approximately 1-μm-long microvilli on the luminal surface of absorptive epithelial cells, which constitute the majority of intestinal epithelial cells. Therefore, the total area of the inner wall of the intestinal tract can be as large as 200 m2 (almost equivalent to the area of a tennis court), allowing for efficient absorption of food components, which comprise nutrients and non-nutrients. Intestinal epithelial cells positioned in the inner lining of the intestinal tract provide an important border that separates the external environment from the inside of the body and are known to possess the following three central physiological functions: (1) absorbing food components (absorptive function); (2) protecting the body from the invasion of foreign substances through contaminated food (barrier function); and (3) acting as sensors environmental factors, including food components, for signal transmission (sensor function) (Fig. ).Citation1,2) Intestinal epithelial cells are thought to be frequently exposed to high concentrations of food components or xenobiotic contaminants. Therefore, the functions of intestinal epithelial cells are highly possibly regulated or modulated by various food components.
Here, we review our work on the characteristics and mechanism of absorption and permeation of various food components into the intestinal epithelium and the physiological effects of food factors on intestinal epithelial cell functions primarily using in vitro models of human intestinal epithelial cells. We also report the influence of xenobiotics from contaminated foods on the function of intestinal epithelial cells.
I. Characteristics and mechanisms of food component absorption or permeation through intestinal epithelial cells
Permeation pathways of food components through the intestinal epithelial monolayer are broadly categorized as follows : (1) the transporter-mediated pathway; (2) energy-dependent intracellular transport pathway via transcytosis, etc.; (3) paracellular transport pathway through the intercellular space; and (4) intracellular passive diffusion pathway.Citation3) We analyzed the mechanisms by which various functional food factors are absorbed by the intestinal epithelium using human intestinal epithelial-like Caco-2 cells, frequently used as an in vitro intestinal epithelial cell model.Citation4)
First, we investigated the characteristics of taurine uptake into the intestinal epithelial cells. Taurine is a sulfur-containing β-amino acid with many physiological functions, including anti-oxidation, osmoregulation, conjugation with bile acids, and modulation of neurotransmitter.Citation5,6) Our findings revealed that taurine is incorporated by intestinal epithelial cells through a Na+- and Cl– -dependent taurine transporter (TAUT; SLC6A6) that is specific for β-amino acids in Caco-2 cells.Citation7) Moreover, TAUT activity was regulated in response to extracellular substrate concentration.Citation7) TAUT uptake and expression were also found to increase under hyperosmotic conditions through calmodulin-dependent protein kinase II.Citation8,9) We elucidated that TAUT is regulated by tumor necrosis factor-alpha (TNF-α), an inflammatory cytokine,Citation10) and that the regulation involves the activation of the transcription factor nuclear factor kappa B (NFκB).Citation11) We also characterized the uptake of neutral, basic, and acidic amino acids by Caco-2 cells through amino acid transporters. Specifically, treatment with cycloheximide, a protein synthesis inhibitor, enhanced the incorporation of leucine and lysine through the activation of the neutral/basic amino acid transport system (system b0,+).Citation12)
Second, we focused on α-lipoic acid, originally a therapeutic agent for diabetes.Citation13,14) Since its use as a food was permitted after a revision of the guidelines for distinction between drugs and foodstuffs in 2004, α-lipoic acid has come into the limelight as a component of functional foods and dietary supplements, similar to coenzyme Q10. However, the absorption mechanisms of α-lipoic acid in the intestinal tract had not been elucidated. Therefore, we examined the characteristics and mechanism by which α-lipoic acid permeates the intestinal epithelium by analyzing cultured Caco-2 cells on a permeable membrane and found that α-lipoic acid rapidly permeated through intestinal epithelial cells.Citation15) The permeation was proton-dependent and was remarkably suppressed by sodium azide, a metabolic inhibitor. In addition, α-lipoic acid permeation was competitively inhibited by octanoic acid, a medium-chain fatty acid, suggesting that the transport of α-lipoic acid is facilitated by an unidentified medium-chain fatty acid transporter. Moreover, we determined that α-lipoic acid was converted by glutathione reductase into dihydrolipoic acid, the reduced form with higher antioxidant activity, in intestinal epithelial cells.Citation15) In addition, the intestinal epithelial permeability of functional food components used in recent years, such as hyaluronic acid and chondroitin sulfate was examined. After their conversion into smaller molecules, these food substances were found to permeate the intestinal epithelium through the paracellular pathway of the Caco-2 monolayer.Citation16,17) Taken together, these results demonstrate that functional food components are absorbed by and permeate through intestinal epithelial cells via various transport pathways (Fig. ).
II. Regulation of intestinal transporters by food components
As described in the previous section, various transporters are expressed in intestinal epithelial cells and are involved in the absorption of food substances. However, food components are expected to not only be absorbed by transporters as substrates on the intestinal epithelium, but also to regulate or modulate other transporter activities. Therefore, we conducted analyses of food components that regulate and modulate the activities of intestinal epithelial transporters. The activity of sodium-dependent glucose transporter 1 (SGLT1), a major glucose transporter on the intestinal epithelium, was inhibited by green tea extract, which contains epicatechin gallate (ECg).Citation18) Moreover, ECg was confirmed to inhibit SGLT1 activity, whereas catechins lacking a gallate group, such as catechin and epicatechin, showed no inhibitory activity against SGLT1, implying that the inhibition of SGLT1 activity by catechins requires a gallate group in addition to the basic catechin structure (Fig. ). Furthermore, ECg binds to the plasma membrane, but ECg itself is not a substrate for SGLT1, which suggests an antagonistic effect of ECg on SGLT1.Citation18) In addition, a search for food components regulating TAUT activity determined that the activity in black sesame extract specifically inhibited TAUT activity.Citation19) One of the inhibitors was ascertained to be lysophosphatidylcholine.Citation20)
Fig. 3. Effect of catechins on SGLT1-mediated glucose uptake activity.
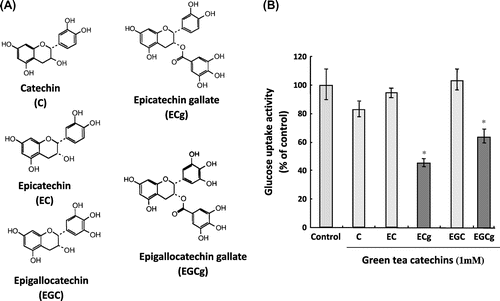
Two types of transporters are expressed in the intestinal epithelium: one includes transporters involved in the uptake from the luminal side, and the other includes efflux transporters that excrete the substances once incorporated by the cells into the luminal side. These include multidrug resistance 1 (MDR1, also called P-glycoprotein), which excretes hydrophobic xenobiotics as a substrate, and multidrug resistance-associated protein 2, which excretes glucuronic acid conjugates as a substrate.Citation21) Then, we searched for food components that regulate MDR1 activity in 40% ethanol extracts of approximately 60 species of vegetables and fruits. Bitter melon extract remarkably inhibited MDR1 activity, and 1-monopalmitin was subsequently identified as an inhibitory component.Citation22) 1-Monopalmitin inhibited the transport activity of MDR1 by inhibiting the ATPase activity of MDR1 but did not function as the substrate of MDR1. These results suggest that intestinal epithelial transporters are subjected to a variety of regulations and modulations by food factors through various mechanisms.
III. Preventive and ameliorative effect of food factors on intestinal inflammation
The recent growing popularity of Western food has resulted in a concomitant increase in the incidence of digestive tract diseases. In particular, patients diagnosed with intestinal inflammation, including inflammatory bowel disease (IBD), have rapidly increased by several folds over the past 20 years and are likely to increase further in the future. IBD, comprising ulcerative colitis and Crohn’s disease, is an intractable disease, the cause of which is unknown but is thought to be due to a failure of the intestinal immune system.Citation23) Abnormally activated macrophages secrete excessive inflammatory cytokines that cause injury to intestinal epithelial cells. Therefore, we attempted to develop a co-culture system (complex culture system) of intestinal epithelial model cells (Caco-2) and activated macrophage model cells (THP-1) to search and analyze food components that can prevent or improve IBD. Caco-2 cells were cultured on a permeable membrane for 2 weeks for differentiation into intestinal epithelial-like cells. THP-1 cells were activated with phorbol myristate acetate for differentiation into activated macrophage-like cells and co-cultured with Caco-2 cells. Caco-2 cells were found to be damaged, demonstrating typical apoptosis and necrosis primarily by TNF-α secreted by THP-1.Citation24) It was confirmed that the addition of a TNF-α-neutralizing antibody (infliximab) or 5-aminosalicylic acid, an IBD therapeutic agent, to this co-culture system remarkably suppressed Caco-2 cellular injury under both conditions. Therefore, we used the co-culture system as an in vitro IBD model and analyzed the food components that could suppress Caco-2 cell damage induced by THP-1 cells.Citation25) Caffeine and taurine were shown to have a suppressive effect. Caffeine reduced Caco-2 cell damage by suppressing the TNF-α production by THP-1. In contrast, taurine suppressed the Caco-2 cell damage by acting on the side of intestinal epithelial cells, not affecting TNF-α production (Fig. ). Furthermore, we examined the effect of taurine in vivo by using a dextran sulfate sodium (DSS)-induced mouse colitis model (DSS provided in water ad libitum). Therefore, it was observed that the prior administration of taurine reduced the symptom of DSS-induced colitis.Citation26) This result revealed a new physiological function of taurine: preventing and improving colitis. Attempts at revealing the regulatory mechanism of colitis prevention by taurine using DNA microarray analysis are currently underway.Citation27) Moreover, this co-culture system was established as a useful in vitro search and evaluation system of food factors that could prevent or improve enteritis (Fig. ).
Fig. 4. In vitro IBD model system using co-culture of intestinal epithelial-like Caco-2 monolayers and activated macrophage-like THP-1 cells.
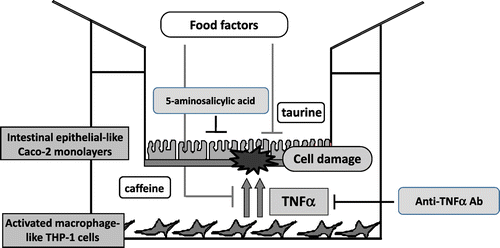
Intestinal epithelial cells secrete humoral factors, including interleukin-8 (IL-8), in response to inflammatory stimulation such as oxidative stress and inflammatory cytokines. The secreted IL-8 chemoattracts and activates immune cells, including neutrophils, underneath the intestinal epithelial cell layer, further aggravating enteritis (called an inflammatory loop). Co-stimulation of Caco-2 cells by H2O2 and TNF-α resulted in a remarkable increase in IL-8 production.Citation28) Therefore, food factors that suppress IL-8 production were screened by the co-stimulation system, and chlorogenic acid (a polyphenol), caffeic acid (a metabolite of chlorogenic acid), soybean isoflavone, and histidine were found to significantly suppress IL-8 production.Citation29–31) Furthermore, the in vitro anti-inflammatory effect of chlorogenic acid was confirmed in vivo by its significant prevention of DSS-induced colitis in mice.Citation32)
IV. Effect of food components on the expression of detoxification enzymes in the intestinal epithelium
Various detoxification or drug-metabolizing enzymes are expressed in intestinal epithelial cells, similar to the liver. These enzymes are involved in the metabolic detoxification of xenobiotic contaminants in foods. Therefore, we searched for food components that enhance the expression and activation of detoxification enzymes that enhance the barrier function against the invasion of xenobiotics. The detoxification system consists of three phases. Xenobiotics are oxidized by phase I enzymes such as cytochrome P (CYPs), conjugated by phase II enzymes, and then excreted from cells by efflux transporters (phase III). The expression of many phase I, II, and III detoxification enzymes is known to be regulated by nuclear receptors.Citation33) One of the nuclear receptors, pregnane X receptor (PXR), is highly expressed in the liver and the intestinal epithelium, and it functions as the master regulator of the expression of detoxification enzymes.Citation34) Therefore, we focused on PXR and screened food components that could activate PXR by constructing a reporter assay system for the search and evaluation of activating components. Briefly, a human PXR expression vector and a reporter vector in which the PXR response sequence was integrated upstream of the luciferase gene were introduced into LS180 cells, a model of human intestinal epithelial cells. The cells were then incubated with phytochemicals from several types of foods to investigate the influence of each phytochemical on PXR-dependent transcription activity. We found that tangeretin (a flavonoid) and ginkgolide A and B (terpenoids from ginkgo leaf extract) enhanced the PXR-dependent transcription activity and the expression and activation of target genes of PXR such as MDR1 (Fig. ).Citation35) Furthermore, the transcriptional activity and expression level of UDP-glucuronosyltransferase 1A1, one of the phase II detoxification enzymes, are increased by baicalein (a flavonoid) via PXR activation.Citation36) We also focused on quinones that are known to be present in foods and exhaust gas and to generate reactive oxygen species in vivo, and we searched for food factors that enhance the expression of NAD(P)H: quinone oxidoreductase 1 (NQO1), a phase II detoxification enzyme that can metabolize quinones to facilitate their detoxification. We found that cysteine remarkably enhanced the expression and activation of NQO1.Citation37) We also revealed that Nf-E2 related factor 2 (Nrf-2), a transcription factor, is involved in the regulation of NQO1 expression. We further confirmed the enhancement of NQO1 expression in vivo. These results indicate that certain types of food factors can enhance the barrier function against the invasion of xenobiotics through the activation of detoxification enzymes by transcription factors such as PXR expressed in the intestinal epithelium.
V. Influence of xenobiotics from contaminated foods on the function of intestinal epithelial cells
Apart from vital nutrients, foods might contain xenobiotics as contaminants, such as endocrine disruptors (environmental hormones). Contamination of food items with such detrimental agents has therefore become a pressing public concern. Hence, we analyzed the toxic influence of xenobiotics on intestinal epithelial cells. Using a DNA microarray, we examined the effects of cadmium, a heavy metal that may contaminate foods like rice, on gene expression of intestinal epithelial cells and found a significant increase in IL-8 mRNA expression.Citation38) Thus, cadmium increases IL-8 expression at the transcriptional level by activating NFκB, after its incorporation into the cells by DMT-1, a divalent iron transporter (Fig. ). This phenomenon was also observed in vivo.Citation39) In addition, we found that the environmental hormone tributyltin permeates the intestinal epithelial cell layer by simple intracellular diffusion and that it inhibits the formation of the cell monolayer by suppressing the formation of tight junctions.Citation40) Moreover, we examined dioxins, which have also become a cause for concern to public health. Dioxins develop their toxicity through the activation of the transcription factor aryl hydrocarbon receptor (AhR). We developed the stable dioxin-responsive HepG2 cell line by introducing a plasmid incorporating the human CYP1A1 promoter containing AhR-responsive elements upstream of the luciferase gene.Citation41) Using this cell line, we searched for phytochemicals that suppress AhR-mediated CYP1A1 promoter activity. We found that some flavonoids, including tangeretin, suppressed CYP1A1 promoter activity, as well as CYP1A1 mRNA and protein expression.Citation42) Furthermore, we observed a phenomenon in which dioxins disrupted the flavonoid metabolic system in the intestinal epithelium, which included facilitating the metabolism of galangin to kaempferol by enhancing the expression and activation of CYP1A1 in Caco-2 cells.Citation43) Therefore, we could partly clarify the mechanisms by which certain xenobiotics in contaminated foods are absorbed by the intestinal epithelium or develop toxic actions therein.
VI. Conclusion
This article describes our analyses of the molecular and cellular mechanism by which food components permeate intestinal epithelial cells. We also introduced the results of our molecular and cellular studies on the physiological roles of certain food components on cellular functions of the intestinal epithelium, which include absorption (via transporters), immunity (via the regulation of cytokine production), and barrier function (via the detoxification system). In addition, our studies could provide novel knowledge on the influence of xenobiotics on intestinal epithelial cells. Understanding the molecular and cellular interaction between the intestinal epithelium and food components is important for understanding the mechanisms of absorption and the physiological functions of food factors in the intestinal tract. In the future, more detailed molecular analyses of the interaction between food factors and molecules in the intestinal epithelial cell layer, which receives and recognizes the factors, will advance the scientific understanding of physiological functions of food factors in the intestinal tract.
Disclosure statement
The author has no conflict of interest to disclose.
Funding
This work was partially supported by a Grant-in-Aid for Scientific Research from Ministry of Education, Culture, Sports, Science, and Technology (MEXT) of Japan.
Acknowledgments
This work was performed at the Laboratory of Food Chemistry, Department of Applied Biological Chemistry, Graduate School of Agricultural and Life Sciences, The University of Tokyo. I would like to express my sincere gratitude to Prof. Makoto Shimizu for his continuous support and invaluable discussion. I am greatly thankful to Prof. Soichi Arai for his helpful guidance and encouragement. I am very grateful to Prof. Keiko Abe, Prof. Toshihide Nishimura, Prof. Yusei Miyamoto, Prof. Ryuichiro Sato, Prof. Hiroto Watanabe, and Prof. Mamoru Totsuka for fruitful discussions. I thank Prof. Kozo Ochi, Prof. Yuzuru Tozawa, Prof. Hugh Rosen, and Dr. Steven J. Brown for their helpful support and encouragement. I also thank all my research collaborators and the members of the Laboratory of Food Chemistry, Department of Applied Biological Chemistry, Graduate School of Agricultural and Life Sciences at The University of Tokyo for their help and technical support.
Notes
This review was written in response to the author’s receipt of the JSBBA Award for Young Scientists in 2012.
Abbreviations: AhR, aryl hydrocarbon receptor; DSS, dextran sulfate sodium; ECg, epicatechin gallate; IBD, inflammatory bowel disease; IL-8, interleukin-8; MDR1, multidrug resistance 1; NQO1, NAD(P)H:quinone oxidoreductase 1; Nrf-2, Nf-E2 related factor 2; NFκB, nuclear factor kappa B; PXR, pregnane X receptor; SGLT1, sodium-dependent glucose transporter; TAUT, taurine transporter; TNF-α, tumor necrosis factor-alpha
References
- McKernan DP, Egan LJ. The intestinal epithelial cell cycle: uncovering its ‘cryptic’ nature. Curr Opin Gastroenterol. 2015;31:124–129.10.1097/MOG.0000000000000154
- Peterson LW, Artis D. Intestinal epithelial cells: regulators of barrier function and immune homeostasis. Nat Rev Immunol. 2014;14:141–153.10.1038/nri3608
- Satsu H. “Functional food components and transporters”: nutrition and food function, and transporters. In: Taketani Y, Satsu H, Ito M, Takeda E, editors. (in Japanese). Tokyo: Kenpakusya; 2011. p. 247–264.
- Hidalgo IJ, Raub TJ, Borchardt RT. Characterization of the human colon carcinoma cell line (Caco-2) as a model system for intestinal epithelial permeability. Gastroenterology. 1989;96:736–749.10.1016/S0016-5085(89)80072-1
- Huxtable RJ. Physiological actions of taurine. Physiol Rev. 1992;72:101–163.
- Shimizu M, Satsu H. Physiological significance of taurine and the taurine transporter in intestinal epithelial cells. Amino Acids. 2000;19:605–614.10.1007/s007260070010
- Satsu H, Watanabe H, Arai S, et al. Characterization and regulation of taurine transport in Caco-2, human intestinal cells. J Biochem. 1997;121:1082–1087.10.1093/oxfordjournals.jbchem.a021698
- Satsu H, Miyamoto Y, Shimizu M. Hypertonicity stimulates taurine uptake and transporter gene expression in Caco-2 cells. Biochim Biophys Acta. 1999;1419:89–96.10.1016/S0005-2736(99)00058-9
- Satsu H, Manabe M, Shimizu M. Activation of Ca2+/calmodulin-dependent protein kinase II is involved in hyperosmotic induction of the human taurine transporter. FEBS Lett. 2004;569:123–128.10.1016/j.febslet.2004.05.062
- Mochizuki T, Satsu H, Shimizu M. Tumor necrosis factor alpha stimulates taurine uptake and transporter gene expression in human intestinal Caco-2 cells. FEBS Lett. 2002;517:92–96.10.1016/S0014-5793(02)02584-X
- Mochizuki T, Satsu H, Shimizu M. Signaling pathways involved in tumor necrosis factor α-induced upregulation of the taurine transporter in Caco-2 cells. FEBS Lett. 2005;579:3069–3074.10.1016/j.febslet.2005.04.063
- Satsu H, Hyun JS, Shin HS, et al. Cycloheximide treatment induces the uptake of neutral and dibasic amino acids via the activation of system b(0,+) in human intestinal Caco-2 cells. J Nutr Sci Vitaminol. 2009;55:44–51.10.3177/jnsv.55.44
- Bast A, Haenen GR. Lipoic acid: a multifunctional antioxidant. Biofactors. 2003;17:207–213.10.1002/biof.v17:1/4
- Moura FA, de Andrade KQ, dos Santos JC, et al. Lipoic acid: its antioxidant and anti-inflammatory role and clinical applications. Curr Top Med Chem. 2015;15:458–483.10.2174/1568026615666150114161358
- Takaishi N, Yoshida K, Satsu H, et al. Transepithelial transport of alpha-lipoic acid across human intestinal Caco-2 cell monolayers. J Agric Food Chem. 2007;55:5253–5259.10.1021/jf063624i
- Hisada N, Satsu H, Mori A, et al. Low-molecular-weight hyaluronan permeates through human intestinal Caco-2 cell monolayers via the paracellular pathway. Biosci Biotechnol Biochem. 2008;72:1111–1114.10.1271/bbb.70748
- Jin M, Satsu H, Yamada K, et al. Permeation of disaccharides derived from chondroitin sulfate through human intestinal Caco-2 Cell monolayers via the paracellular pathway. Biosci Biotechnol Biochem. 2010;74:1243–1249.10.1271/bbb.100075
- Kobayashi Y, Suzuki M, Satsu H, et al. Green tea polyphenols inhibit the sodium-dependent glucose transporter of intestinal epithelial cells by a competitive mechanism. J Agric Food Chem. 2000;48:5618–5623.10.1021/jf0006832
- Ishizuka K, Kanayama A, Satsu H, et al. Identification of a taurine transport inhibitory substance in sesame seeds. Biosci Biotechnol Biochem. 2000;64:1166–1172.10.1271/bbb.64.1166
- Ishizuka K, Miyamoto Y, Satsu H, et al. Characteristics of lysophosphatidylcholine in its inhibition of taurine uptake by human intestinal Caco-2 Cells. Biosci Biotech Biochem. 2002;66:730–736.10.1271/bbb.66.730
- Dietrich CG, Geier A, Oude Elferink RP. ABC of oral bioavailability: transporters as gatekeepers in the gut. Gut. 2003;52:1788–1795.10.1136/gut.52.12.1788
- Konishi T, Satsu H, Hatsugai Y, et al. Inhibitory effect of a bitter melon extract on the P-glycoprotein activity in intestinal Caco-2 cells. Br J Pharmacol. 2004;143:379–387.10.1038/sj.bjp.0705804
- Singh D, Srivastava S, Pradhan M, et al. Inflammatory bowel disease: pathogenesis, causative factors, issues, drug treatment strategies, and delivery approaches. Crit Rev Ther Drug Carrier Syst. 2015;32:181–214.10.1615/CritRevTherDrugCarrierSyst.v32.i3
- Satsu H, Ishimoto Y, Nakano T, et al. Induction by activated macrophage-like THP-1 cells of apoptotic and necrotic cell death in intestinal epithelial Caco-2 monolayers via tumor necrosis factor-alpha. Exp Cell Res. 2006;312:3909–3919.10.1016/j.yexcr.2006.08.018
- Watanabe F, Satsu H, Mochizuki T, et al. Development of the method for evaluating protective effect of food factors on THP-1-induced damage to human intestinal Caco-2 monolayers. Biofactors. 2004;21:145–147.10.1002/biof.v21:1/4
- Zhao Z, Satsu H, Fujisawa M, Hori et al. Attenuation by dietary taurine of dextran sulfate sodium-induced colitis in mice and of THP-1-induced damage to intestinal Caco-2 cell monolayers. Amino Acids. 2008;35:217–224.10.1007/s00726-007-0562-8
- Gondo Y, Satsu H, Ishimoto Y, et al. Effect of taurine on mRNA expression of thioredoxin interacting protein in Caco-2 cells. Biochem Biophys Res Commun. 2012;426:433–437.10.1016/j.bbrc.2012.08.116
- Shin HS, Zhao Z, Satsu H, et al. Synergistic effect of tumor necrosis factor-alpha and hydrogen peroxide on the induction of IL-8 production in human intestinal Caco-2 cells. Inflammation. 2011;34:440–447.10.1007/s10753-010-9251-y
- Zhao Z, Shin HS, Satsu H, et al. 5-caffeoylquinic acid and caffeic acid down-regulate the oxidative stress- and TNF-alpha-induced secretion of interleukin-8 from Caco-2 cells. J Agric Food Chem. 2008;56:3863–3868.10.1021/jf073168d
- Satsu H, Hyun JS, Shin HS, et al. Suppressive effect of an isoflavone fraction on tumor necrosis factor-alpha-induced interleukin-8 production in human intestinal epithelial Caco-2 cells. J Nutr Sci Vitaminol. 2009;55:442–446.10.3177/jnsv.55.442
- Son DO, Satsu H, Shimizu M. Histidine inhibits oxidative stress- and TNF-alpha-induced interleukin-8 secretion in intestinal epithelial cells. FEBS Lett. 2005;579:4671–4677.10.1016/j.febslet.2005.07.038
- Shin HS, Satsu H, Bae MJ, et al. Anti-inflammatory effect of chlorogenic acid on the IL-8 production in Caco-2 cells and the dextran sulphate sodium-induced colitis symptoms in C57BL/6 mice. Food Chem. 2015;168:167–175.10.1016/j.foodchem.2014.06.100
- Kaminsky LS, Zhang QY. The small intestine as a xenobiotic-metabolizing organ. Drug Metab Dispos. 2003;31:1520–1525.10.1124/dmd.31.12.1520
- Kliewer SA, Goodwin B, Willson TM. The nuclear pregnane X receptor: a key regulator of xenobiotic metabolism. Endocr Rev. 2002;23:687–702.10.1210/er.2001-0038
- Satsu H, Hiura Y, Mochizuki K, et al. Activation of pregnane X receptor and induction of MDR1 by dietary phytochemicals. J Agric Food Chem. 2008;56:5366–5373.10.1021/jf073350e
- Hiura Y, Satsu H, Hamada M, et al. Analysis of flavonoids regulating the expression of UGT1A1 via xenobiotic receptors in intestinal epithelial cells. Biofactors. 2014;40:336–345.10.1002/biof.v40.3
- Satsu H, Chidachi E, Hiura Y, et al. Induction of NAD(P)H: quinone oxidoreductase 1 expression by cysteine via Nrf2 activation in human intestinal epithelial LS180 cells. Amino Acids. 2012;43:1547–1555.10.1007/s00726-012-1230-1
- Hyun JS, Satsu H, Shimizu M. Cadmium induces Interleukin-8 production via NF-κB activation in the human intestinal epithelial cell, Caco-2. Cytokine. 2007;37:26–34.10.1016/j.cyto.2007.02.011
- Zhao Z, Hyun JS, Satsu H, et al. Oral exposure to cadmium chloride triggers an acute inflammatory response in the intestines of mice, initiated by the over-expression of tissue macrophage inflammatory protein-2 mRNA. Toxicol Lett. 2006;164:144–154.10.1016/j.toxlet.2005.12.004
- Tsukazaki M, Satsu H, Mori A, et al. Effects of tributyltin on barrier functions in human intestinal Caco-2 cells. Biochem Biophys Res Commun. 2004;315:991–997.10.1016/j.bbrc.2004.01.147
- Natsume Y, Satsu H, Kitamura K, et al. In vitro system for assessing dioxin absorption by intestinal epithelial cells and for preventing this absorption by food substances. Cytotechnology. 2005;47:79–88.10.1007/s10616-005-3753-8
- Hamada M, Satsu H, Natsume Y, et al. TCDD-induced CYP1A1 expression, an index of dioxin toxicity, is suppressed by flavonoids permeating the human intestinal Caco-2 cell monolayers. J Agric Food Chem. 2006;54:8891–8898.10.1021/jf060944t
- Hamada M, Satsu H, Ashida H, et al. Metabolites of galangin by 2,3,7,8-tetrachlorodibenzo- p -dioxin-inducible cytochrome P450 1A1 in human intestinal epithelial Caco-2 Cells and their antagonistic activity toward aryl hydrocarbon receptor. J Agric Food Chem. 2010;58:8111–8118.10.1021/jf100778f