Abstract
We provide the first large-scale quantitative proteomics analysis in Hyriopsis schlegelii. To investigate the proteins expressed in the gonads, a quantitative proteomics approach has been utilized to analyze differentially expressed proteins between the spermary and ovary. In this study, we identified and quantified 2416 proteins in the gonads of Hyriopsis schlegelii. Of these, 559 proteins showed significantly different expression between the spermary and ovary. Some specific proteins expressed in either the spermary or ovary were identified in Hyriopsis schlegelii. In addition, a series of proteins related to gametogenesis were also identified. Compared with previous reports, many proteins in Hyriopsis schlegelii identified here have different expression patterns between the spermary and ovary. The special hermaphroditism in Hyriopsis schlegelii may contribute to these inconsistent results. The provided proteomics data could be considered as a starting point for subsequent studies focusing on the proteins involved in sexual gland development and maturity.
Graphical abstract
The distribution of 2416 proteins based on the differential expression between spermary and ovary.
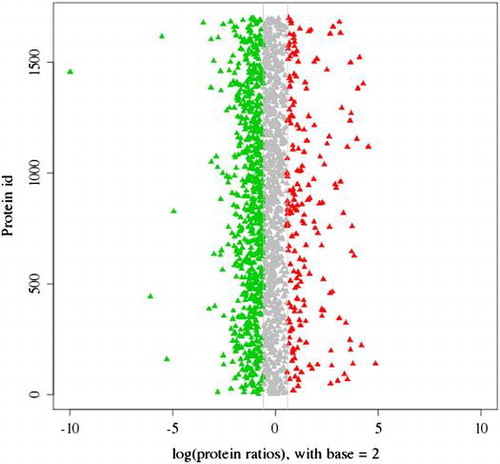
Proteomics technology has become a useful tool for screening tissue proteins. Male-related proteins from testes in humans,Citation1) mice,Citation2) ascidianCitation3), and abaloneCitation4) and female-related proteins from ovaries in mouse,Citation5) cattle,Citation6) zebrafish,Citation7) honeybeeCitation8), and shrimpCitation9) have been investigated. Some sexual gland development- and maturity-related proteins, including Ilf2 and Ilf3 (interleukin enhancer-binding factors 2 and 3), Raldh3 (retinaldehyde dehydrogenase type 3), Zgc:195027 (low-density lipoprotein-related receptor protein 3), and Sept5a (septin 5a), were identified by proteomics analysis. The expression patterns of the above-mentioned proteins suggested that they may play a specific role in sexual gland development and maturity. The molecular mechanisms governing sexual gland development and maturity could be revealed by further investigation of these proteins. However, comparative proteomics analysis of spermary and ovary in freshwater bivalves has been very limited.
Bivalves exhibit both dioeciousCitation10) and hermaphroditicCitation11,12) reproduction. In the development process, some bivalves have the ability to transform between hermaphroditism and gonochorism.Citation13) Because of the wide variations in sexuality, the bivalve mollusk has become an excellent animal model for studying sexual gland development and maturity.Citation14)
Hyriopsis schlegelii is an important mussel species for freshwater pearl production in China. The spermary and ovary in Hyriopsis schlegelii are wrapped in visceral mass. The spermary and ovary consist of the follicle, genital canal and gonoduct, and their shape is described as grape-like or dendritic branch-like. Sexual gland development can be divided into five stages: proliferating stage, growing stage, maturing stage, spawning stage, and resting stage. These stages are identified by cytological characteristics and the relative amounts of developing sexual cells in the follicle. As reproductive organs, the normal development and maturity of the spermary and ovary have significance in the subsequent successful fertilization of sperm and egg, seedling culture, and propagation. A special hermaphroditic phenomenon was observed in the spermary of some individuals.Citation15) To identify and quantify the proteins related to development and maturity of sexual glands, we used quantitative proteomics technology to compare protein expression between the spermary and ovary. We specifically chose spermary and ovary samples that had previously been investigated at the transcript level.Citation16) This is the first publication using proteomics technology for a freshwater bivalve. The data from our study may contribute to existing sequence resources and accelerate research on the molecular mechanisms of sexual gland development and maturity in freshwater bivalves.
Materials and methods
Experimental materials
Approximately 4-year-old adult Hyriopsis schlegelii specimens were obtained from the Fuzhou Hongmen Reservoir Exploitation Limited Company of Jiangxi Province in China. For the iTRAQ experiment, four individual samples of total protein from spermaries and ovaries were pooled into two subgroups to minimize individual variation and enhance signals (Fig. ).
Protein extraction and iTRAQ reagent labeling
Total protein was extracted with a tissue protein extraction kit (Cwbio, Tianjin, China). In brief, the spermary or ovary tissue was homogenized in the protein extraction buffer supplied by the kit, mixed with Triton X-100 (10%), incubated on ice for 20 min and then centrifuged (10,000 × g, 20 min, 4 °C). The supernatant was collected and the protein concentration was analyzed with the BCA Protein Assay Kit (Cwbio, Tianjin, China). iTRAQ analysis was carried out as previously reported at the Beijing Genomics Institute (BGI, Shenzhen, China).Citation17) Briefly, extracted protein samples (100 μg each) were reduced with 10 mM DTT (56 °C for 1 h), alkylated with 55 mM iodoacetamide (room temperature for 45 min), and digested using sequencing-grade trypsin (37 °C for 12 h). The protein concentrations were measured using a 2-D Quant Kit (Amersham Biosciences). The completion of tryptic digestion was examined by SDS-PAGE, and the tryptic peptides were lyophilized and dissolved in 50% TEAB buffer. According to the manufacturer’s instructions, the tryptic peptides were labeled using iTRAQ 8-plex kits (AB Sciex Inc., MA, USA) at room temperature for 2 h. The labeled peptide mixtures were then pooled and dried by vacuum centrifugation.
Strong cation exchange fractionation
After labeling and quenching, the samples were combined and lyophilized. Subsequently, the samples were redissolved in 4 mL of buffer A (25% (v/v) CAN and 25 mM NaH2PO4, pH 3.0) for fractionation by strong cation exchange (SCX) chromatography on an Ultremex SCX column (4.6 mm × 250 mm) using a Shimadzu LC-20AB HPLC. The peptides were collected at a rate of 1 mL/min with buffer A for 10 min, 0–20% buffer B (10 mM KH2PO4, 25% ACN, 500 mM KCL, pH 3.0) for 15 min, and 20–40% buffer B for 10 min. The system was then maintained in 80% buffer B for 3 min before equilibrating with buffer A for 10 min prior to the next injection. The chromatograms were recorded by measuring the absorbance at 214 nm, and fractions were collected every 1 min. The collected peptides were desalted with a Strata X C18 column (Phenomenex), concentrated to dryness using vacuum centrifuge and reconstituted in 0.1% formic acid for LC–MS/MS analysis.
Peptides identified by TripleTOF 5600
A TripleTOF 5600 System (AB SCIEX, Concord, ON) coupled with an online micro flow HPLC system (Shimadzu, JAPAN) was used for peptide identification. The separation was performed at a flow rate of 20 μL/min with a splitter to obtain an effective flow rate of 0.2 μL/ min. The mass spectrometry data were acquired in the positive ion mode, with a selected mass range of 300–2000 m/z. For the information-dependent acquisition (IDA), survey scans performed in 250 ms and peptides with +2 to +4 charge states were selected for MS/MS. Total cycle time was fixed to 3.3 s. The Q2 transmission window was 100 Da for 100%.
Data analysis
All the raw data files were converted into mgf file format using Proteome Discoverer 1.2 (Thermo Scientific), and all the wiff data files were converted into mgf file format by Protein Pilot software (version 4.2 AB SCIEX, USA). Protein identification and quantification were performed using Mascot software (version 2.3.02, Matrix Science Inc, Boston, MA).Citation18) The search parameters were as follows: MS/MS ion search; trypsin enzyme; MS/MS fragment mass tolerance ±0.1 Da; monoisotopic mass values; variable modifications of Gln to pyro-Glu (N-termQ), oxidation (M) and iTRAQ 8-plex (Y); and tryptic peptides with ≤1 missed cleavage site. Carbamidomethylation of cysteine and iTRAQ 8-plex modification of the N-terminus and K were set as fixed modifications. Peptide tolerance was set to 20 ppm, and MS/MS tolerance was set to 0.05 Da. To reduce false positive results, all data were reported based on a 95% confidence level and a false discovery rate less than 1%. For quantitative analysis, a 1.5-fold cut-off value was used to identify up-regulated and down-regulated proteins with a p-value of less than 0.05 (default parameters in Mascot Software package). The quantitation was performed at the peptide level by following the procedures described in http://www.matrixscience.com/help/quant_statistics_help.html. Student’s t-test was performed using Mascot 2.3.02 software. Protein quantitation information is provided in Supplementary Tables 1 and 2.
Bioinformatic analysis of proteins
The protein sequences were mapped to Gene Ontology terms by local blast against a reference database downloaded from the website resource (GO-Annotation@EBI). Clusters of Orthologous Groups of proteins system (COGs) can be employed for functional annotation. The Kyoto Encyclopedia of Genes and Genomes (KEGG) is an updating system that computerizes the current knowledge of biochemical pathways and molecular interactions. KEGG can be used as a reference for the systematic interpretation of sequence data.Citation19)
Results
Identification of proteins in Hyriopsis schlegelii
In this study, a total of 246,910 spectra were generated from the iTRAQ experiment using spermary and ovary as materials. The data were further analyzed by Mascot software. Mascot identified a total of 28,002 spectra matched to known spectra, 21,415 spectra matched to unique peptides, 9180 peptides, 8714 unique peptides and 2416 proteins (Fig. ).
Fig. 2. Spectra, peptides and proteins identified from iTRAQ proteomics by searching against bioinformatic database. The X-axis indicates the identification category. And the Y-axis indicates the number. Total Spectra: the total of second order spectrogram. Spectra: the number of spectrogram after the quality control. Unique Spectra: the number of spectrogram matched with special peptides. Peptide: the number of identification peptides. Unique Peptide: the number of identification special peptides. Protein: the number of identification proteins.
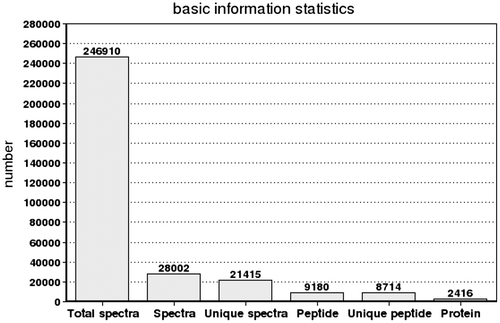
COG classification of the Hyriopsis schlegelii proteomes
All proteins were searched against the database of Clusters of Orthologous Groups of proteins (COGs) on NCBI (http://www.ncbi.nlm.nih.gov/COG/). In total, 1,808 sequences have a COG classification. Among the 23 COG categories, the cluster for “General function prediction” represents the largest group (341), followed by “Posttranslational modification, protein turnover, chaperones” (203) and “Translation, ribosomal structure and biogenesis” (164). The following categories represent the smallest groups we identified: RNA processing and modification (90), Chromatin structure and dynamics (13), Nuclear structure (2) and Cell motility (2) (Fig. ).
Gene Ontology (GO) characterization of the Hyriopsis schlegelii proteomes
GO assignments were used to classify and predict the functions of proteins. All protein sequences could be categorized into 41 functional groups based on sequence homology. The three main categories were marked as biological process, cellular component, and molecular function. “Cellular process” (1330 members), “Cell” (1520 members), and “binding” (1273 members) terms are dominant. Proteins from the categories of “reproduction” (127 members) and “reproductive process” (126 members) were also identified (Fig. ). Although the number of reproduction and reproductive process-related proteins is limited, they may play important roles in spermiogenesis, oocyte development and maturation, and fertilization. For example, testis-specific chromodomain protein Y 1-like, testis-specific serine kinase 1-like, testis-expressed 14, sperm flagellar protein 1, sperm flagellar protein 2-like, spermatogenesis-associated serine-rich 2-like, sperm-associated antigen 6-like, sperm adenylate kinase, sperm autoantigenic protein 17-like, (human nuclear autoantigenic sperm protein) homologue family member (nasp-2)-like, sperm phosphodiesterase 5-like, super sex combs-like, epididymal secretory protein E1-like, prostaglandin D2 synthase, 14-3-3 proteins, many maternal proteins, yolk ferritin, vitellogenin, receptor for egg jelly 4-like, carboxypeptidase, vitellogenic-like, and zonadhesin-like were identified in the reproduction and reproductive process categories of GO in Hyriopsis schlegelii. These proteins, which have been reported to be involved in spermiogenesis, oocyte development and maturation, and fertilization in other animals, may have similar functions in Hyriopsis schlegelii.
Fig. 4. GO classification of proteins after blastx search. Histogram presentation of the GO annotation was generated automatically by the web histogram tool WEGO (http://wego.genomics.org.cn/cgibin/wego/index.pl). The proteins were classified at the second level under three root GO domains: cellular component, molecular function and biological process. The right and left y-axes indicate the number and the corresponding percentage of a certain ontology within each root domain, respectively. One protein could be annotated into more than one GO term.
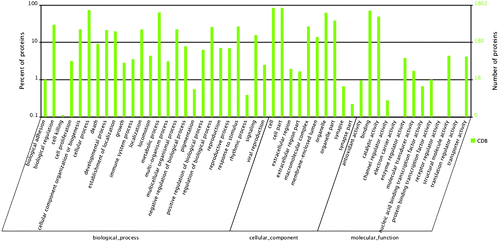
In addition, we mapped all the annotated protein sequences to the reference canonical pathways in the Kyoto Encyclopedia of Genes and Genomes (KEGG). The pathways with the most representation by unique protein sequences were metabolic pathways (428 members), biosynthesis of secondary metabolites (149 members), and microbial metabolism in diverse environments (113 members).
Comparison of protein profiles in the spermary and ovary
A total of 2416 proteins were identified by the proteomics analysis in Hyriopsis schlegelii (Fig. ). On the basis of the applied criteria (1.5-fold or greater change and p < 0.05), 559 proteins were identified as differentially expressed between the spermary and ovary, and this included 123 proteins that were up-regulated and 436 proteins that were down-regulated in the ovary (Fig. ). Proteins that show no obvious expression change (<1.5-fold) and 1.5-fold up-regulation between spermary and ovary are listed in Tables .
Fig. 5. The distribution of 2416 proteins based on the differential expression between spermary and ovary. Red dots and green dots indicated the up-regulated and the down-regulated proteins in ovary, respectively. Shaded dots designated the proteins that showed no obvious expression changes.
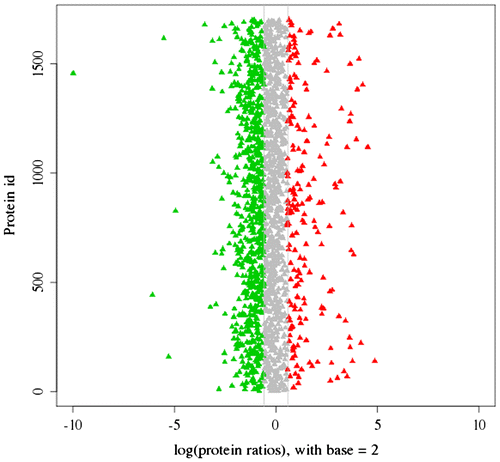
Fig. 6. The quantity statistics of differential expression proteins. The x-axis indicates the denotation of comparison groups. And the y-axis denotes the number of differential expression proteins. Red column indicates the number of up-regulated proteins, but the green column indicates the number of down-regulated proteins.
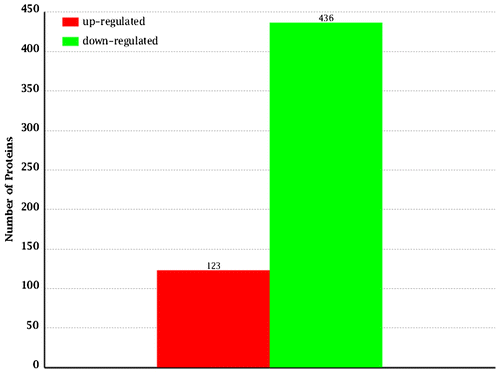
Table 1. Proteins showing upregulation in spermary, possibly relevant for gonad development and function
Table 2. Proteins showing upregulation in ovary, possibly relevant for gonad development and function.
Table 3. Proteins showing inconsistent expression between spermary and ovary, possibly relevant for gonad development and function.
Discussion
Proteins related to spermiogenesis
Some signaling proteins related to spermiogenesis were identified in this study. Guanine nucleotide-binding regulatory proteins (G proteins) play roles in spermatogenesis and mediating ZP3-induced acrosomal exocytosis.Citation20) Nucleoside diphosphate kinase B (NDPK B) is involved in spermiogenesis by increasing the expression of the antioxidant enzyme glutathione peroxidase 5.Citation21) Four proteins, including three G-protein subunits and NDPK B, were identified in Hyriopsis schlegelii. The presence of these signaling proteins suggests that signaling pathways related to spermatogenesis in other animals may exist in Hyriopsis schlegelii. In addition, three testis-specific proteins and eight sperm proteins were also identified in Hyriopsis schlegelii. These 11 spermatogenesis proteins were expressed in the spermary, indicating that these proteins may be related to spermatogenesis in Hyriopsis schlegelii.
Component proteins of spermatozoa
Actin and tubulin proteins are highly expressed in specific flagellum in spermatozoa. Actin is present in the flagellum, neck, and postacrosomal area of the head of spermatozoa in rams.Citation22) Tubulin isoforms were observed in the mouse sperm flagellum by immunogold analyses.Citation23) Seven actin and five tubulin proteins were identified in Hyriopsis schlegelii. These proteins may be among the main components of flagellum in spermatozoa. Moreover, other flagellum accessory proteins, including tektin A1, tektin-3 and ropporin 1-like protein, were also identified. These proteins have been reported to assist in sperm motility.
Maternal proteins
Eight maternal proteins, including Hsp70, Hsp90a, 78 kDa glucose-regulated protein (GRP78), glucose-regulated protein 94 (GRP94), oxygen-regulated protein 150, calreticulin, calnexin, and protein disulfide isomerase, have been identified in the mature mouse egg.Citation24) GRP78 was required for the cell proliferation of embryos and the protection of early mouse embryos from apoptosis.Citation25) As a maternal factor, proliferative cell nuclear antigen (PCNA) was up-regulated in high-quality oocytes.Citation26) PCNA expression facilitates the processivity of DNA replication and increases during oogenesis in the pacific oyster.Citation27,28) Many maternal proteins, including heat shock protein 70, heat shock protein 90, glucose-regulated protein 94, 78 kDa glucose-regulated protein, calreticulin, calnexin, four protein disulfide isomerases and PCNA, were identified in Hyriopsis schlegelii. The function of these proteins is not yet known. The accumulation of these maternal proteins in the oocyte may help prepare for oocyte maturation and early embryogenesis in Hyriopsis schlegelii.
Oocyte development and maturation proteins
During oocyte development, vitellogenin proteins are cleaved to generate yolk proteins and are regarded as the nutrient source for embryos.Citation29) As maternal protein factors, vitellogenins are related to oocyte quality in the pacific oyster Crassostrea gigas.Citation26) 14-3-3 proteins, which are expressed in oocytes and ovarian follicular cells, play roles in ovarian development, meiosis and oocyte maturation.Citation30) In this study, two vitellogenin proteins, one yolk protein, and three 14-3-3 protein isoforms were identified in Hyriopsis schlegelii. These proteins may be vital for oogenesis and early embryo development.
With respect to oocyte maturation, purines and phosphoinositides participate in the spontaneous and progesterone-induced maturation in Bufo arenarum full-grown oocytes.Citation31) Purine metabolic pathways are involved in inducing the resumption of mouse oocyte maturation.Citation32) Two kinds of enzymes involved in purine synthesis, including two phosphoribosylaminoimidazole proteins (PAIs) and nine ATP synthase subunits, were identified in Hyriopsis schlegelii. The presence of these proteins suggests that purine metabolism in the maintenance of oocyte maturation may also exist in Hyriopsis schlegelii.
Proteins related to fertilization
Axonemal dyneins play a role in regulating sperm motility.Citation33) Six dynein proteins and a high mobility group 1 protein related to sperm motility were identified in Hyriopsis schlegelii. These proteins may be related to sperm motility during the fertilization process. In sperm capacitation, proteasomes were reported to be involved in human sperm capacitation and the exocytosis of the acrosome reaction.Citation34,35) In the sea urchin Pseudocentrotus depressus, the sperm proteasome plays a key role in the acrosome reaction and sperm penetration through the vitelline envelope.Citation36) Eight proteasome subunits were identified in Hyriopsis schlegelii. All eight proteins have a relatively high expression level in the spermary. Although the function of proteasomes in the spermatozoa of Hyriopsis schlegelii has not been validated, these proteins may participate in sperm capacitation and acrosome reaction.
Isocitrate dehydrogenase (IDH) is involved in energy homeostasis and is crucial for fertility in mouse oocytes.Citation37) Active cofilins are essential for oocyte maturation and fertilization by increasing the Ca2+ signals.Citation38) After fertilization, cofilins migrate along the membrane and at the interface between the cortical and animal hemisphere cytoplasm.Citation39) IDH and cofilin/actin-depolymerizing factor homologue were also identified in Hyriopsis schlegelii. In addition, zonadhesin-like and egg jelly protein, which are related to fertilization in other animals, were also identified in Hyriopsis schlegelii. The expression of these proteins in the ovary may be related to oocyte maturation and the success of fertilization.
Proteins showing inconsistent expression
Some proteins showing inconsistent expression between the spermary and ovary were identified in this study. For example, testis-expressed 14, sperm flagellar protein 1, sperm flagellar protein 2-like, spermatogenesis-associated, serine-rich 2-like, prostaglandin reductase 1, and 15-oxoprostaglandin 13-reductase, which were reported to be only or highly expressed in the spermary in other animals, showed no obvious differences in expression between the spermary and ovary in Hyriopsis schlegelii. In addition, some proteins related to oogenesis, including the receptor for egg jelly 4-like, 14-3-3zeta protein, and septin2, which were reported to be expressed only in the ovary in zebrafish,Citation40) also showed no obvious differences between the spermary and ovary in our study.
Carboxypeptidase, vitellogenic-like and septin 7Citation41) play roles in the development and maturation of oocytes. However, in our analysis, the expression of carboxypeptidase, vitellogenic-like and septin 7 is higher in the spermary than in the ovary. The epididymal secretory protein E1-like and prostaglandin D2 synthase are necessary to maintain the morphology and function of sperm. Here, the expression of epididymal secretory protein E1-like and prostaglandin D2 synthase is significantly higher in the ovary than in the spermary. In Hyriopsis schlegelii, from 26 to 32 months, a hermaphroditic phenomenon can be observed in males. At this stage, both female and male follicles exist in a gonad, and these follicles are arranged in the form of a mutual cross. The reasons for the unexpected protein expression that we observed between the spermary and ovary are still unclear. Excluding concerns with the sequencing technology, these extraordinary results may be due to the existence of hermaphrodites in Hyriopsis schlegelii.
Conclusion
To the best of our knowledge, this is the first publication using iTRAQ technology for a freshwater bivalve. These sequence data can be used as a tool for future spermatogenesis and oogenesis experiments and for immunity,Citation42) growth and apoptosis studies of freshwater bivalves as a whole. Our work did not discuss each of the candidate proteins in detail. The goal of this preliminary work focuses on delineating the primary comparative protein profiles of the spermary and ovary. Continued work will be necessary to assess the specific function of individual proteins in the reproductive process of Hyriopsis schlegelii.
Author contributions
Jianwu Shi and Yijiang Hong conceived the experiments. Jianwu Shi, Dexia Wang, Yan Zhou, Yiran Gu, and Di Wu designed and performed the experiments. Jianwu Shi analyzed the data. Junhua wang contributed reagents/materials/analysis tools. Jianwu Shi and Yijiang Hong wrote the paper.
Disclosure statement
No potential conflict of interest was reported by the authors.
Funding
The present work was supported by the National Natural Science Foundation of China [grant number 31660337]; the Natural Science Foundation of Jiangxi Province, China [20161BAB204169]; the Science and Technology Research Project of Jiangxi Province, China [150164]; the Key Scientific and Technological Program of Jiangxi Province, China [KJLD12001], [20132BBF60053]; the earmarked fund for Jiangxi Agriculture Research System [JXARS-10], [JXARS-02].
Supplemental materials
Supplemental material for this article can be accessed at http://dx.doi.org/10.1080/09168451.2016.1259553.
TBBB_1259553_Supplemental_Material.zip
Download Zip (234 KB)References
- Baker MA. Identification of gene products present in Triton X-100 soluble and insoluble fractions of human spermatozoa lysates using LC-MS/MS analysis. Proteomics Clin Appl. 2007;1:1–9.
- Baker MA, Hetherington L, Reeves GM, et al. The mouse sperm proteome characterized via IPG strip prefractionation and LC-MS/MS identification. Proteomics. 2008;8:1720–1730.10.1002/(ISSN)1615-9861
- Nakachi M, Nakajima A, Nomura M, et al. Proteomic profiling reveals compartment-specific, novel functions of ascidian sperm proteins. Mol Reprod Dev. 2011;78:529–549.10.1002/mrd.21341
- Palmer MR, McDowall MH, Stewart L, et al. Mass spectrometry and next-generation sequencing reveal an abundant and rapidly evolving abalone sperm protein. Mol Reprod Dev. 2013;80:460–465.
- Vitale AM, Calvert ME, Mallavarapu M, et al. Proteomic profiling of murine oocyte maturation. Mol Reprod Dev. 2007;74:608–616.10.1002/(ISSN)1098-2795
- Bhojwani M, Rudolph E, Kanitz W, et al. Molecular analysis of maturation processes by protein and phosphoprotein profiling during in vitro maturation of bovine oocytes: a proteomic approach. Cloning Stem Cells. 2006;8:259–274.10.1089/clo.2006.8.259
- Knoll-Gellida A, Andre M, Gattegno T, et al. Molecular phenotype of zebrafish ovarian follicle by serial analysis of gene expression and proteomic profiling, and comparison with the transcriptomes of other animals. BMC Genomics. 2006;7:46.10.1186/1471-2164-7-46
- Cardoen D, Ernst UR, Boerjan B, et al. Worker honeybee sterility: a proteomic analysis of suppressed ovary activation. J Proteome Res. 2012;11:2838–2850.10.1021/pr201222s
- Talakhun W, Roytrakul S, Phaonakrop N, et al. Identification of reproduction-related proteins and characterization of the protein disulfide isomerase A6 cDNA in ovaries of the giant tiger shrimp Penaeus monodon. Comp Biochem Physiol D. 2012;7:180–190.
- Chung EY. Ultrastructural studies of oogenesis and sexual maturation in female Chlamys (Azumapecten) farreri farreri (Jones & Preston, 1904)(Pteriomorphia: Pectinidae) on the western coast of Korea. Malacologia. 2008;50:279–292.10.4002/0076-2997-50.1.279
- Hwang JJ. Reproductive cycles of the pearl oysters, Pinctada fucata (Gould) and Pinctada margaritifera (Linnaeus) (Bivalvia: Pteriidae) in southwestern Taiwan waters. J Mar Sci Technol-Ta. 2007;15:67–75.
- Derbali A, Jarboui O, Ghorbel M, et al. Reproductive biology of the pearl oyster, Pinctada radiata (Mollusca: Pteriidae), in northern Kerkennah Island (Gulf of Gabes). Cah Biol Mar. 2009;50:215–222.
- Mackie GL. Bivalves. Mollusca. 1984;7:351–418.
- Lv J, Feng L, Bao Z, et al. Molecular characterization of RXR (Retinoid X Receptor) gene isoforms from the bivalve species Chlamys farreri. PLoS One. 2013;8:e74290.10.1371/journal.pone.0074290
- Yu Y, Hong YJ, Qiu QJ, et al. Embryonic development and breading-season gonad in Hyriopsis schlegeli. Chin J Zool. 2008;43:102–107.
- Shi JW, Hong YJ, Sheng JQ, et al. De novo transcriptome sequencing to identify the sex-determination genes in Hyriopsis schlegelii. Biosci Biotech Biochem. 2015;79:1257–1265.10.1080/09168451.2015.1025690
- Yang LT, Qi YP, Lu YB, et al. iTRAQ protein profile analysis of Citrus sinensis roots in response to long-term boron-deficiency. J Proteomics. 2013;93:179–206.10.1016/j.jprot.2013.04.025
- Perkins DN, Pappin DJ, Creasy DM, et al. Probability-based protein identification by searching databases using mass spectrometry data. Electrophoresis. 1999;20:3551–3567.10.1002/(ISSN)1522-2683
- Kanehisa M, Araki M, Goto S, et al. KEGG for linking genomes to life and the environment. Nucleic Acids Res. 2008;36:D480–D484.
- Glassner M, Jones J, Kligman I, et al. Immunocytochemical and biochemical characterization of guanine nucleotide-binding regulatory proteins in mammalian spermatozoa. Dev Biol. 1991;146:438–450.10.1016/0012-1606(91)90245-X
- Choi YJ, Cho SK, Hwang KC, et al. Nm23-M5 mediates round and elongated spermatid survival by regulating GPX-5 levels. FEBS Lett. 2009;583:1292–1298.10.1016/j.febslet.2009.03.023
- De las Heras MA, Valcarcel A, Perez LJ, et al. Actin localization in ram spermatozoa: effect of freezing/thawing, capacitation and calcium ionophore-induced acrosomal exocytosis. Tissue Cell. 1997;29:47–53.10.1016/S0040-8166(97)80071-7
- Fouquet JP, Prigent Y, Kann ML. Comparative immunogold analysis of tubulin isoforms in the mouse sperm flagellum: unique distribution of glutamylated tubulin. Mol Reprod Dev. 1996;43:358–365.10.1002/(ISSN)1098-2795
- Calvert ME, Digilio LC, Herr JC, et al. Oolemmal proteomicsidentification of highly abundant heat shock proteins and molecular chaperones in the mature mouse egg and their localization on the plasma membrane. Reprod Biol Endocrinol. 2003;1:27.10.1186/1477-7827-1-27
- Luo S, Mao C, Lee B, et al. GRP78/BiP is required for cell proliferation and protecting the inner cell mass from apoptosis during early mouse embryonic development. Mol Cell Biol. 2006;26:5688–5697.10.1128/MCB.00779-06
- Corporeau C, Vanderplancke G, Boulais M, et al. Proteomic identification of quality factors for oocytes in the Pacific oyster Crassostrea gigas. J Proteomics. 2012;75:5554–5563.10.1016/j.jprot.2012.07.040
- Franco A, Jouaux A, Mathieu M, et al. Proliferating cell nuclear antigen in gonad and associated storage tissue of the Pacific oyster Crassostrea gigas: seasonal immunodetection and expression in laser microdissected tissues. Cell Tissue Res. 2010;340:201–210.10.1007/s00441-009-0923-6
- Jouaux A, Heude-Berthelin C, Sourdaine P, et al. Gametogenic stages in triploid oysters Crassostrea gigas: irregular locking of gonial proliferation and subsequent reproductive effort. J Exp Mar Biol Ecol. 2010;395:162–170.10.1016/j.jembe.2010.08.030
- Zhang S, Wang S, Li H, et al. Vitellogenin, a multivalent sensor and an antimicrobial effector. Int J Biochem Cell Biol. 2011;43:303–305.10.1016/j.biocel.2010.11.003
- De S, Marcinkiewicz JL, Vijayaraghavan S, et al. Expression of 14-3-3 protein isoforms in mouse oocytes, eggs and ovarian follicular development. BMC Res Notes. 2012;5:57.10.1186/1756-0500-5-57
- Zelarayan L, Oterino J, Sanchez Toranzo G, et al. Involvement of purines and phosphoinositides in spontaneous and progesterone induced nuclear maturation of Bufo arenarum oocytes. J Exp Zool. 2000;287:151–157.10.1002/(ISSN)1097-010X
- Downs SM, Eppig JJ. Induction of mouse oocyte maturation in vivo by perturbants of purine metabolism. Biol Reprod. 1987;36:431–437.10.1095/biolreprod36.2.431
- Kingtong S, Kellner K, Bernay B, et al. Proteomic identification of protein associated to mature spermatozoa in the Pacific oyster Crassostrea gigas. J Proteomics. 2013;26:81–91.10.1016/j.jprot.2013.02.009
- Kong M, Diaz ES, Morales P. Participation of the human sperm proteasome in the capacitation process and its regulation by protein kinase A and tyrosine kinase. Biol Reprod. 2009;80:1026–1035.10.1095/biolreprod.108.073924
- Morales P. Participation of the sperm proteasome in human fertilization. Hum Reprod. 2003;18:1010–1017.10.1093/humrep/deg111
- Yokota N, Sawada H. Sperm proteasomes are responsible for the acrosome reaction and sperm penetration of the vitelline envelope during fertilization of the sea urchin Pseudocentrotus depressus. Dev Biol. 2007;308:222–231.10.1016/j.ydbio.2007.05.025
- Dumollard R, Ward Z, Carroll J, et al. Regulation of redox metabolism in the mouse oocyte and embryo. Development. 2007;134:455–65.
- Nusco GA, Chun JT, Ercolano E, et al. Modulation of calcium signalling by the actin-binding protein cofilin. Biochem Biophys Res Commun. 2006;348:109–114.10.1016/j.bbrc.2006.07.023
- Abe H, Obinata T, Minamide LS, Bamburg JR. Xenopus laevis actin-depolymerizing factor/cofilin: a phosphorylation-regulated protein essential for development. J Cell Biol. 1996;132:871–885.10.1083/jcb.132.5.871
- Groh KJ, Nesatyy VJ, Segner H, et al. Global proteomics analysis of testis and ovary in adult zebrafish (Danio rerio). Fish Physiol Biochem. 2011;37:619–647.10.1007/s10695-010-9464-x
- Li S, Ou XH, Wei L, et al. Septin 7 is required for orderly meiosis in mouse oocytes. Cell Cycle. 2012;11:3211–3218.10.4161/cc.21553
- Wang CY, Sheng JQ, Hong YJ, et al. Molecular characterization and expression of metallothionein from freshwater pearl mussel, Hyriopsis schlegelii. Biosci Biotech Bioch. 2016;80:1327–1335.