Abstract
Bovine type I collagen consists of two α1 and one α2 chains, containing the internal triple helical regions and the N- and C-terminal telopeptides. In industries, it is frequently digested with porcine pepsin to produce a triple helical collagen without the telopeptides. However, the digestion mechanism is not precisely understood. Here, we performed a mass spectrometric analysis of the pepsin digest of the N-terminal telopeptide pQLSYGYDEKSTGISVP (1–16) in the α1 chain. When purified collagen was digested, pQLSYGY (1–6) and pQLSYGYDEKSTG (1–12) were identified, while DEKSTG (7–12) was not. When the N-terminal telopeptide mimetic synthetic peptide pQLSK(MOCAc)GYDEKSTGISK(Dnp)P-NH2 was digested, pQLSK(MOCAc)GYDEKSTG (1–12) and ISK(Dnp)P-NH2 (13−16) were readily identified, pQLSK(MOCAc)GY (1−6) and DEKSTGISK(Dnp)P-NH2 (7−16) were weakly detected, and DEKSTG (7–12) was hardly identified. These results suggest that pepsin preferentially cleaves Tyr6–Asp7 and less preferentially Gly12–Ile13. They also suggest that the former cleavage requires native collagen structure, while the latter cleavage does not.
Graphical abstract
Pepsin preferentially cleaves Tyr6–Asp7 and less preferentially Gly12–Ile13. The former cleavage requires native collagen structure, while the latter cleavage does not.
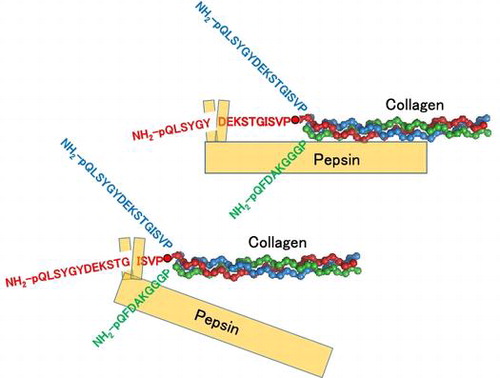
Key words:
Collagen is located in connective tissues including skin, bone, tendon, cartilage, and teeth, exerting various biological functions.Citation1) It contains the triple helix and the N- and C-terminal telopeptides. Of all types of collagen, type I is the most abundant. Bovine type I collagen consists of two α1 and one α2 chains. The number of amino acid residues of the N-terminal telopeptide, internal helical region, and C-terminal telopeptides are 16, 1014, and 26, respectively, for the α1 chain (NCBI Reference Sequence: NP_001029211.1) and 9, 1014, and 15, respectively, for the α2 chain (NP_776945.1).Citation2,3) In industries, acetic acid is used to extract collagen, and pepsin is frequently used with acetic acid to extract collagen without the telopeptides, so-called atelocollagen, from bovine skins. Collagen and its digestion products (collagen peptides) are used as functional foods and diagnostic tools.Citation1,4–6) Several bacterial and mammal enzymes are related to collagen degradation.Citation7) Collagen digestion takes place under various physiological and pathological conditions.Citation1,7) For example, Nakayama et al. showed that Flavobacterium psychrophilum collagenase is the putative toxin responsible for bacterial cold water disease that affects ayu (Plecoglossus altivelis).Citation8)
Under weak acidic condition, pepsin cleaves the N- and the C-terminal telopeptides. Edman degradation analysis has shown that collagen is cleaved at Tyr6–Asp7 and Gly12–Ile13 in the N-terminal telopeptide of the α1 chain (Fig. (A)) and at Phe2-Asp3 in the N-terminal telopeptide of the α2 chain.Citation9) On a SDS-PAGE under reducing conditions, type I collagen partially loses its high-dimensional structure and is separately detected as six major bands that correspond to two monomeric chains (α1 and α2 chains), three dimeric chains (β11, β12, and variant β11 chains), and one trimeric chain (γ chain). The β11 chain consists of two α1 chains covalently linked at the N-terminal telopeptide; the β12 chain consists of one α1 and one α2 chains covalently linked at the N-terminal telopeptide; and the variant β11 chain consists of two α1 chains that linked both at the N- and the C-terminal telopeptides.Citation10,11) The γ chain consists of two α1 and one α2 chains linked at the N- and/or C-terminal telopeptides.Citation6) In the previous study, we focused on the variant β11 chain among the monomeric and polymeric chains of bovine type I collagen and developed a method to evaluate the kinetics of the digestion of bovine type I collagen with porcine pepsin, in which the amount of variant β11 chain was quantified in SDS-PAGE and utilized as an index.Citation12)
Fig. 1. Digestion of bovine type I collagen with pepsin.
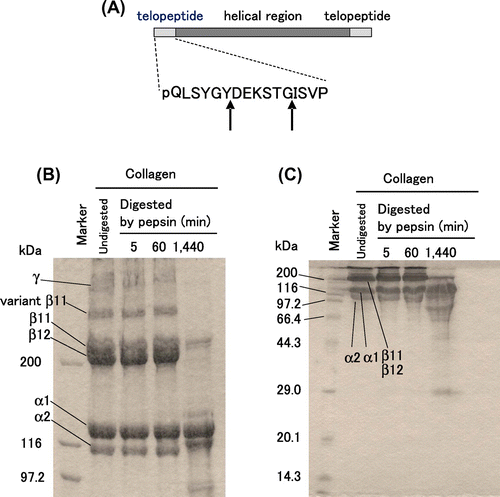
In this study, we aimed to characterize the digestion of the α1 chain of bovine type I collagen using mass spectrometry. In the digestion of the α1 chains of bovine type I collagen with porcine pepsin, as already mentioned, two cleavage sites (Tyr6–Asp7 and Gly12–Ile13) had been identified in the N-terminal telopeptide through Edman degradation;Citation9) however, the order of priority for these two sites remains to be elucidated. Besides, how these sites are cleaved in the cross-linked chains such as the variant β11 was unknown. To address these issues, we performed a mass spectrometric analysis of the pepsin digest of bovine type I collagen extracted from bovine skins with acetic acid, focusing on the monomeric and dimeric chains of α1. Pepsin digest of the synthetic fluorescent peptide that mimics the N-terminal telopeptide of the collagen α1 chain was also examined.
Materials and methods
Materials
Acid-soluble collagen (ASC), which was extracted and purified from bovine skin without losing native triple helical structure (3 mg/mL in 5 mM acetic acid), was obtained from Nippi Inc. (Tokyo, Japan). Pepsin extracted from porcine stomach mucosa was purchased from Nacalai Tesque (Kyoto, Japan). L-pyroGln-L-Leu-L-Ser-L-Lys-(7-methoxycoumarin-4-yl)acetyl-Gly-L-Tyr-L-Asp-L-Glu-L-Lys-L-Ser-L-Thr-Gly-L-Ile-L-Ser-L-Lys-[N3-(2,4-dinitrophenyl)]-L-Pro-NH2 [pQLSK(MOCAc)GYDEKSTGISK(Dnp)P-NH2] (2102.2 Da) and pQLSK(MOCAc)GY (893.9 Da) were synthesized in Peptide Institute (Osaka, Japan). Its concentration was determined by the denoted molecular weight.
Digestion of purified collagen with pepsin and gel filtration chromatography
ASC (3 mg/mL in 5 mM acetic acid) was diluted to 2.52 mg/mL with 50 mM sodium acetate buffer (pH 4.0). Pepsin was dissolved to 3 μM with 0.7 M sodium acetate buffer (pH 4.0). The hydrolysis reaction was initiated by mixing 900 μL of collagen solution and 100 μL of pepsin solution. The reaction temperature was 37 °C. At 5 min, the reaction was stopped by adding 200 μL of 3.0 M Tris-HCl buffer (pH 7.5). The solution was applied to the column (3.0 cm inner diameter × 60 cm) packed with a BioGel P4 (Biorad, Hercules, CA) equilibrated with 0.7 M sodium acetate buffer (pH 4.0). The flow rate was 1.2 mL/min, the fraction volume was 9.6 mL, and 100 fractions were collected. Each fraction was assessed for measurement of absorbance at 280 nm (A280) and SDS-PAGE.
Digestion of the N-terminal telopeptide mimetic synthetic fluorescent peptide with pepsin
For kinetic analysis, the peptide solution (1 mM in dimethyl sulfoxide (DMSO)) was diluted to 20 μM with either 0.7 M HCl–KCl (pH 2.1–2.6), 0.7 M glycine-HCl buffer (pH 2.6–3.5) or 0.7 M sodium acetate buffer (pH 3.5–5.5). The hydrolysis reaction was initiated by mixing 117 μL of 20 μM peptide solution and 13 μL of pepsin solution (3 μM in water). The initial peptide and pepsin concentrations were 18 μM and 0.3 μM, respectively. The reaction temperatures were set stepwise in the range of 30–65 °C. The reaction was monitored by following the increase in fluorescence intensity at 400 nm with excitation at 325 nm with an EnSight (PerkinElmer, Waltham, MA) for 30 min. The amount of the product was estimated by fluorescence intensity by comparison with that of the pQLSK(MOCAc)GY solution. On the assumption that the hydrolysis of the peptide was carried out under pseudo-first order conditions, the specificity constant (kcat/Km) was calculated by Equation (Equation1(1) ):
(1)
where vo, [E]o, and [S]o mean the initial reaction rate, the initial enzyme concentration, and the initial substrate concentration, respectively.
For LC–MS/MS analysis, the peptide solution (1 mM in DMSO) was diluted to 20 μM with 0.7 M sodium acetate buffer (pH 4.0). The hydrolysis reaction was initiated by mixing 117 μL of the 20 μM peptide solution and 13 μL of pepsin solution (3 μM in water). The initial peptide and pepsin concentrations were 18 μM and 0.3 μM, respectively. The reaction was carried out in 0.63 M sodium acetate buffer (pH 4.0), 1.8% v/v DMSO at 37 °C.
SDS-PAGE
SDS-PAGE was performed under reducing conditions. The reaction solutions or the control solutions were mixed with four volumes of the SDS-PAGE sample buffer (0.25 M Tris-HCl buffer (pH 6.8), 50% v/v glycerol, 10% w/v SDS, 5% v/v 2-mercaptoethanol, 0.05% w/v bromophenol blue) and were boiled for 10 min. The solution (10 μl) was applied to 5% polyacrylamide gel with a constant current of 40 mA for 35 min. After electrophoresis, proteins were stained with 0.25% Coomassie Brilliant Blue R-250, 45% methanol, and 10% acetic acid. Molecular weight marker (Protein Molecular Weight Marker (Broad)) was purchased from Takara Bio Inc. (Otsu, Japan).
LC–MS/MS
The enzymatic digests or the control solutions were purified and concentrated using C-18 spin column (Thermo Scientific, Waltham, MA) and resuspended in 0.1% formic acid. Ten μl of the concentrated purified collagen digest or the digest of the 18 pmol of synthetic peptide was separated using Nano-LC-Ultra 2D-plus cHiPLC Nanoflex (Eksigent, Dublin, CA) with trap column (200 μm × 0.5 mm ChromXP C18-CL 3 μm 120 Å (Eksigent)) and analytical column (75 μm × 15 cm ChromXP C18-CL 3 μm 120 Å (Eksigent)). The separation was carried out using a binary gradient with mobile phases A (98% water, 2% acetontrile, 0.1% formic acid) and B (20% water, 80% acetonitrile, 0.1% formic acid). The gradient program was as following: 2–40% B for 50 min, 90% B for 5 min, and 2% B for 19 min, at a flow rate of 300 nL/min. The eluates from the analytical column were directly infused to a mass spectrometer, TripleTOF 5600+ System (SCIEX, Framingham, MA). The data-sets were acquired using information dependent acquisition method.
Identification of peptide fragments
Peptide identification from the enzymatic digests of collagen was carried out by screening the existence of protonated molecular ions ([M + H]+, [M + 2H]2+, [M + 3H]3+, [M + 4H]4+, and [M + 5H]5+) for the expected fragments in the LC–MS/MS datasets. The screening was based on the extracted ion chromatograms (XICs) generated using m/z values corresponding to the putative adducts. Identifications of several fragments yielded distinct peaks with MSMS spectrum, including pQLSYGYDEKSTG (1–12) ([M + 2H]2+), were conducted using ProteinPilot software version 4.5β (SCIEX) and UniProtKB/Swiss-Prot database (Bos Taurus, June 2014). Peptides with confidence more than 95% were considered to be identified. Identification of pQLSYGY (1–6) ([M + H]+) was confirmed by the comparison of the acquired MSMS fragmentation pattern with the expected MSMS fragmentation pattern that was calculated by ProteinProspector (http://prospector.ucsf.edu). Since highly purified collagen or synthetic peptide were utilized in this study, identification of the cross-linked peptides, labeled peptides (except for the peptide for which we have standard), and peptides yielded small peaks without MSMS spectrum, were provisionally carried out based on the screening of XIC peaks with the expected m/z values. For further evaluation, the acquired spectrum was compared to the background/reference spectrum, which was obtained from the control samples from which substrate or enzyme was omitted, or different substrate or enzyme was used. Isotope and spectral distributions were used for the confirmation of the monoisotopic mass and charged states of the detected ions. Retention times were also taken into consideration to evaluate the different adducts from the common peptide. XICs were generated with tolerance +/−0.025 m/z using Peakview software (SCIEX). Peak areas were calculated using MultiQuant software (SCIEX).
Results and discussion
Analysis of the pepsin digests of purified collagen
We previously reported that pepsin cleaves the N-terminal telopeptide of collagen at pH 4.0 at 37 °C.Citation12) As shown in Fig. (B) and (C), collagen treated with pepsin (0.3 μM) at pH 4.0 at 37 °C for 5 or 60 min (1 h) yielded clear high molecular weight bands that correspond to polymeric chains, including the variant β11 chain, suggesting that collagen retained triple helical structure. On the other hand, prolonged reaction for 1440 min (24 h) notably decreased these bands, suggesting that collagen denaturation progressed slowly under this condition, and the triple helical region was cleaved after the long time incubation. To avoid such undesired denaturation and cleavage, collagen (2.52 mg/mL) was digested with pepsin (0.3 μM) at pH 4.0 at 37 °C for 5 min and used in this study.
We attempted to remove undigested collagen and pepsin from the pepsin digests of collagen by gel filtration chromatography to prepare the samples for LC–MS/MS. For this purpose, BioGel P4 resin (typical fractionation range is 800–4000 Da) was used. When the pepsin digests of purified collagen was applied, fractions 12–16 yielded high molecular weight protein bands corresponding to the α1 chain upon SDS-PAGE (Fig. S1). Considering the fractionation range of the resin, it was thought that these fractions also contained β and γ chains and pepsin although they were not detected upon SDS-PAGE. Fractions 21–51 exhibited three large peaks of A280 values likely corresponding to the digested peptides (Fig. (A)). When the synthetic fluorescent peptide that mimics the N-terminal telopeptide of type I collagen α1 chain, pQLSK(MOCAc)GYDEKSTGISK(Dnp)P-NH2, was applied to the same gel filtration chromatography, fluorescence measurement indicated that the digested N-terminal telopeptide of the type I collagen α1 chain mainly elute in fractions 30–40 (data not shown).
Fig. 2. Analyses of purified collagen digested with pepsin.
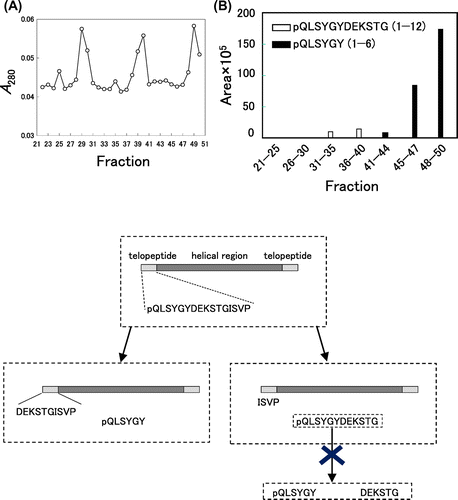
Fractions 21–25, 26–30, 31–35, 36–40, 41–44, 45–47, and 48–50 of gel filtration chromatography were combined separately, concentrated with a concentration ratio of 30, and applied to LC–MS/MS to discriminate the peptide fragments included in each sample. We focused on three fragments, pQLSYGYDEKSTG (1–12), pQLSYGY (1–6) and DEKSTG (7–12), to better understand the digestion at Tyr6–Asp7 and Gly12–Ile13 in the N-terminal α1 chain of bovine type I collagen. pQLSYGYDEKSTG (1–12) was strongly detected in fractions 31–35 and 36–40. pQLSYGY (1–6) was identified in fractions 41–44, 45–47 and 48–50. DEKSTG (7–12) was not identified from any fractions in our system. No fragments were identified in fractions 21–25 or 26–30. We speculate that the first of the three large peaks of A280 values in Fig. (A) corresponds to the mixture of various peptide fragments contained in the collagen and/or pepsin preparations. As shown in the representative TICs and XICs (Figs. and ), we could identify clear peaks of pQLSYGYDEKSTG (1–12) and pQLSYGY (1–6) in XICs, although they were difficult to be identified in TIC. Although the ionization efficiencies and matrix effects were different for each fragment and in each fraction, we estimated the abundance of each peptide based on the total XIC peak area. As shown in Fig. (B), the total peak area for pQLSYGY (1–6) was much larger than that of pQLSYGYDEKSTG (1–12). These results could be interpreted as following; (i) the N-terminal telopeptide of collagen is preferentially cleaved at Tyr6–Asp7 and less preferentially at Gly12–Ile13. (ii) pQLSYGYDEKSTG, released from the purified collagen through the digestion at Gly12–Ile13, is not effectively cleaved at Tyr6–Asp7 by pepsin (Fig. (C)).
Fig. 3. TICs obtained from LC–MS/MS analysis of collagen digested with pepsin.
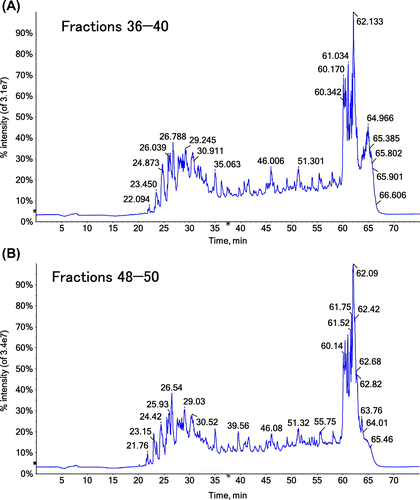
Fig. 4. XICs in LC–MS/MS analysis of purified collagen digested with pepsin.
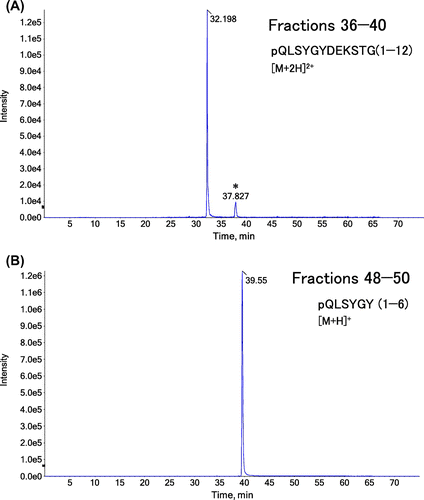
In type I collagen, two Lys9 residues in the α1 chain are cross-linked as allysines in a certain proportion.Citation9,10,13) In this study, cross-linked peptides consisting of two pQLSYGYDEKSTG fragments (1–12), one DEKSTG (6–12) and pQLSYGYDEKSTG fragments (1–12), or two DEKSTG fragments (6–12) were not detected. This suggests that pepsin does not cleave the cross-linked two α1 chains at Gly12–Ile13 on both strands. However, it is unknown whether pepsin cleaves them at Tyr6–Asp7 on single or both strands or Gly12–Ile13 on single strand.
Analysis of the pepsin digests of the N-terminal telopeptide mimetic synthetic fluorescent peptide
To characterize the digestion kinetics of the N-terminal telopeptide of collagen α1 chain by pepsin in more detail, we designed and synthesized a collagen N-terminal telopeptide mimetic fluorescent peptide using MOCAc-Dnp system (Fig. (A)). This system is widely accepted to investigate the enzymatic digestion of peptides. The fluorescence from MOCAc is quenched by Dnp through intramolecular fluorescence energy transfer when the two fluorophores are located in close proximity. In the synthetic peptide we have designed, enzymatic digestion between the labels (Lys4-Lys15) results in the emission of the MOCAc fluorescence. Using this system, we tried to characterize the overall kinetics of the digestion between the labels by pepsin, which is expected to exhibit the mixed outcome of the digestion at Tyr6–Asp7 and Gly12–Ile13.
Fig. 5. Kinetics of the digestion of the fluorescent synthetic peptide with pepsin.
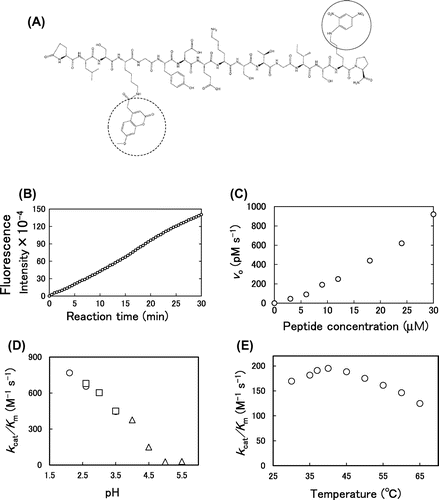
The reaction was carried out with 18 μM fluorescent peptide and 0.3 μM pepsin at 37 °C, at pH 4.0 for 30 min. As expected, the fluorescence intensity increased linearly as the reaction time increased (Fig. (B)). Assuming that the initial reaction rate obeys Michaelis–Menten equation, the effect of the initial peptide concentration on the reaction rate was examined. The peptide (0–30 μM) was incubated with pepsin (0.3 μM) at pH 4.0, at 37 °C, and the initial reaction rate (vo) was measured. The vo increased linearly against the initial peptide concentration, suggesting that the Km value is much higher than 30 μM (Fig. C). Next, the effect of pH on vo was examined. The peptide (18 μM) was incubated with pepsin (0.3 μM) at various pH (2.1–5.5) at 37 °C. The kcat/Km decreased with increasing pH. This profile was similar to that of the kcat/Km values in the digestion of collagen with pepsin.Citation12) Since pepsin is most active under acidic conditions and inactive at pH > 6.5, it seemed that the result simply reflected the pH dependence of pepsin activity (Fig. (D)). The effect of temperature on vo was finally examined. The peptide (18 μM) was incubated with pepsin (0.3 μM) at pH 4.0 at various temperatures (30–65 °C). The kcat/Km values exhibited a peak at 40 °C. This profile was different from that of the kcat/Km values in the digestion of purified collagen with pepsin, in which the kcat/Km values were relatively constant at 30–65 °C.Citation9) The difference can be explained by that the stability of pepsin and the melting of the triple helix are involved in the digestion of bovine type I collagen with pepsin,Citation13–16) while the stability of pepsin is only involved in the digestion of the peptide (Fig. (E)).
To obtain further insights into the digestion mechanism of the N-terminal telopeptide of collagen α1 chain, pepsin digest of the fluorescent synthetic peptide was applied to LC–MS/MS, and the results were compared with the results obtained from the purified collagen. Since our interest was on the digestion at Tyr6–Asp7 and Gly12–Ile13 in the N-terminal telopeptide of collagen α1 chain, we focused on the following peptides expected to be produced, pQLSK(MOCAc)GYDEKSTG (1–12), ISK(Dnp)P-NH2 (13–16), pQLSK(MOCAc)GY (1–6), DEKSTGISK(Dnp)P-NH2 (7–16), and DEKSTG (7–12), in addition to the full-length undigested peptide pQLSK(MOCAc)GYDEKSTGISK(Dnp)P-NH2 (1–16).
As shown in Fig. (A), three peaks were strongly detected in TIC of the pepsin digest of the synthetic peptide. These peaks were identified as the ions derived from pQLSK(MOCAc)GYDEKSTG (1–12) (a), ISK(Dnp)P-NH2 (13–16) (b) and pQLSK(MOCAc)GYDEKSTGISK(Dnp)P-NH2 (1–16) (c), as judged from the predominant ions in each peak, and comparison with the spectrum obtained from the control samples (data not shown). The representative XIC peaks for the m/z values corresponding to these three peptides are shown in Fig. . In comparison to these peptides, DEKSTGISK(Dnp)P-NH2 (7–16) and pQLSK(MOCAc)GY (1–6) were weakly detected (<10% of the strongly detected group of peptides), and DEKSTG (7–12) was faintly detected or hardly identified in our system (Fig. S2). The difference between the pepsin digests of collagen and those of the synthetic peptide could be interpreted as following: the synthetic peptide was predominantly cleaved at Gly12–Ile13, while faintly cleaved at Tyr6–Asp7, and the resulting pQLSK(MOCAc)GYDEKSTG (1–12) was rarely cleaved at Tyr6–Asp7 (Fig. (B)). However, the effect of MOCAc on the cleavage cannot be fully denied.
Fig. 6. LC–MS/MS analysis of fluorescent synthetic peptide digested with pepsin.
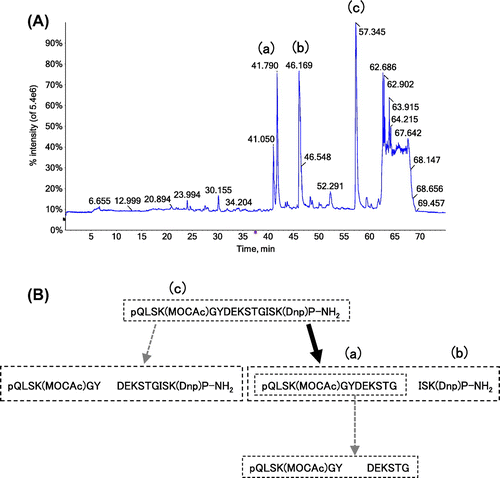
Fig. 7. Notes: XICs corresponding to the most abundant adducts for the strongly-detected group of peptides in LC–MS/MS analysis of fluorescent peptide digested with pepsin. XICs were generated from the data-set used in Fig. (A), with m/z values corresponding to the indicated adducts. The retention times for peak a1, b1, and c1 in (A), (B), and (C), are identical to those of peak a, b, and c in TIC shown in Fig. (A).
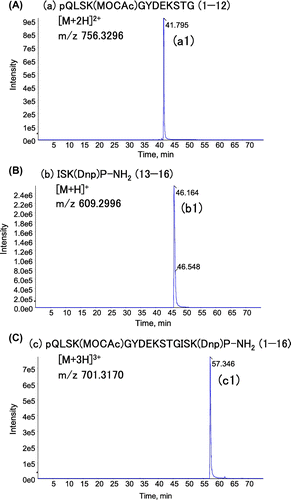
Combined with the results obtained from the digestion of the purified collagen, our results suggest that native structure of collagen protein and/or peptide is necessary for the effective cleavage at Tyr6–Asp7 but not for that at Gly12–Ile13. Pepsin is less specific than other proteases. Ahn et al.Citation17) pointed out that the substrate specificity of pepsin was affected by the conformation of substrate proteins rather than the amino acid sequences in the cleavage site. Our hypothesis is in agreement with this. However, it is not known how the native structure of collagen affects the cleavage at Tyr6–Asp7. The elucidation of the actual structural determinants for the different cleavage efficiency at Tyr6–Asp7 and Gly12–Ile13 in the substrates used in this study is the subject of future investigation.
Limitations
Although similar methods are used in the global quantification of peptides and/or proteins through LC–MS/MS, the relative quantification of peptides based on the detected peak areas cannot be directly converted to the absolute abundance due to the different ionization efficiency and matrix effects. Examinations of the ionization patterns and efficiencies with all possible standard peptides in each matrix may help more accurate quantification of each peptide in future. The elucidation of the involvement of chains other than monomeric and dimeric α1, and the minor digestion sites other than the ones we have focused, will be necessary to understand the whole picture of the collagen N-terminal peptide degradation by pepsin. It should be noted that our screening method left most of the peptides with post-translational modifications unidentified.
Conclusion
When bovine type I collagen was digested with porcine pepsin, the N-terminal telopeptide of the α1 chain was preferentially cleaved at Tyr6–Asp7 and less preferentially at Gly12–Ile13. The native structure of collagen protein seemed necessary for the cleavage at Tyr6–Asp7, but not for that at Gly12–Ile13.
Author contribution
K.Y. designed research; J.Q., S.I. J.S., H.G., and K.Y. performed research; J.Q., S.I., J.S., K.T., S.H., K.K., T.T., and K.Y. analyzed data; J.Q., S.I., and K.Y. wrote the manuscript.
Disclosure statement
No potential conflict of interest was reported by the authors.
Funding
The Medical Research Support Center, Graduate School of Medicine, Kyoto University, was supported by Platform for Drug Discovery, Informatics, and Structural Life Science from the Ministry of Education, Culture, Sports, Science and Technology, Japan.
Supplemental material
Supplemental material for this article can be accessed at http://dx.doi.org/10.1080/09168451.2016.1263146
Figure S1
Download MS Power Point (476.5 KB)Acknowledgments
The authors thank Dr. Kumiko Yoshizawa-Kumagaye of Peptide Institute, Inc. for the design of synthetic fluorescent peptide.
Notes
Abbreviations: ASC, acid soluble collagen; LC–MS/MS, liquid chromatography–tandem mass spectrometry; TIC, total ion chromatogram; XIC, extracted ion chromatogram.
References
- Kadler KE, Baldock C, Bella J, et al. Collagen at a glance. J Cell Sci. 2007;120:1955–1958.10.1242/jcs.03453
- Hofmann H, Fietzek PP, Kűhn K. The role of polar and hydrophobic interactions for the molecular packing of type I collagen: a three-dimensional evaluation of the amino acid sequence. J Mol Biol. 1978;125:137–165.10.1016/0022-2836(78)90342-X
- Jones EY, Miller A. Structural models for the N- and C-terminal telopeptide regions of interstitial collagens. Biopolymers. 1987;26:463–480.10.1002/(ISSN)1097-0282
- Nam KA, You SG, Kim SM. Molecular and physical characteristics of squid (Todarodes pacificus) skin collagens and biological properties of their enzymatic hydrolysates. J Food Sci. 2008;73:C249–C255.10.1111/j.1750-3841.2008.00722.x
- Taga Y, Kusubata M, Ogawa-Goto K, et al. Highly accurate quantification of hydroxyproline-containing peptides in blood using a protease digest of stable isotope-labeled collagen. J Agric Food Chem. 2014;62:12096–12102.10.1021/jf5039597
- Kusubata M, Koyama Y, Tometsuka C, et al. Detection of endogenous and food-derived collagen dipeptide prolylhydroxyproline (Pro-Hyp) in allergic contact dermatitis-affected mouse ear. Biosci Biotechnol Biochem. 2015;79:1356–1361.10.1080/09168451.2015.1027653
- Watanabe K. Collagenolyic proteases from bacteria. Appl Microbiol Biotechnol. 2004;63:520–526.10.1007/s00253-003-1442-0
- Nakayama H, Tanaka K, Teramura N, et al. Expression of collagenase in Flavobacterium psychrophilum isolated from cold-water disease-affected ayu (Plecoglossus altivelis) Biosci Biotechnol Biochem. 2016;80:135–144.
- Sato K, Ebihara T, Adachi E, et al. Possible involvement of aminotelopeptide in self-assembly and thermal Stability of collagen I as revealed by its removal with proteases. J Biol Chem. 2000;275:25870–25875.10.1074/jbc.M003700200
- Duconseille A, Astruc T, Quintana N, et al. Gelatin structure and composition linked to hard capsule dissolution: a review. Food Hydrocolloids. 2015;43:360–376.10.1016/j.foodhyd.2014.06.006
- Eyre DR, Weis M, Hudson DM, et al. A novel 3-hydroxyproline (3Hyp)-rich motif marks the triple-helical C terminus of tendon type I collagen. J Biol Chem. 2011;286:7732–7736.10.1074/jbc.C110.195768
- Qian J, Okada Y, Ogura T, et al. Kinetic analysis of the digestion of bovine type I collagen telopeptides with porcine pepsin. J Food Sci. 2016;81:C27–C34.10.1111/1750-3841.13179
- Reiser K, McCormick RJ, Rucker RB. Enzymatic and nonenzymatic cross-linking of collagen and elastin. FASEB J. 1992;6:2439–2349.
- Komsa-Penkova R, Koynova R, Kostov G, et al. Thermal stability of calf skin collagen type I in salt solutions. Biochim Biophys Acta. 1996;1297:171–181.10.1016/S0167-4838(96)00092-1
- Komsa-Penkova R, Koynova R, Kostov G, et al. Discrete reduction of type I collagen thermal stability upon oxidation. Biophys Chem. 1999;83:185–195.
- Duan R, Zhang J, Du X, et al. Properties of collagen from skin, scale and bone of carp (Cyprinus carpio). Food Chem. 2009;112:702–706.10.1016/j.foodchem.2008.06.020
- Ahn J, Cao M-J, Yu YQ, et al. Accessing the reproducibility and specificity of pepsin and other aspartic proteases. Biochim Biophys Acta. 2013;1834:1222–1229.10.1016/j.bbapap.2012.10.003