Abstract
A Gram-negative rubber-degrading bacterium, Rhizobacter gummiphilus NS21 grew and produced aldehyde metabolites on a deproteinized natural rubber (DPNR)-overlay agar medium forming a clearing zone. A transposon-insertion mutant, which had lost the ability to degrade DPNR, was isolated to identify the rubber degradation genes. Sequencing analysis indicated that the transposon was inserted into a putative oxygenase gene, latA. The deduced amino acid sequence of latA has 36% identity with that of roxA, which encodes a rubber oxygenase of Xanthomonas sp. strain 35Y. Phylogenetic analysis revealed that LatA constitutes a distinct group from RoxA. Heterologous expression in a Methylibium host and deletion analysis of latA indicated that the latA product is responsible for the depolymerization of DPNR. The quantitative reverse transcription-PCR analysis indicated that the transcription of latA is induced during the growth on DPNR. These results strongly suggest that latA is directly involved in the degradation of rubber in NS21.
Graphical abstract
LatA oxygenase is responsible for the cleavage of poly(cis-1,4-isoprene) in Rhizobacter gummiphilus NS21.
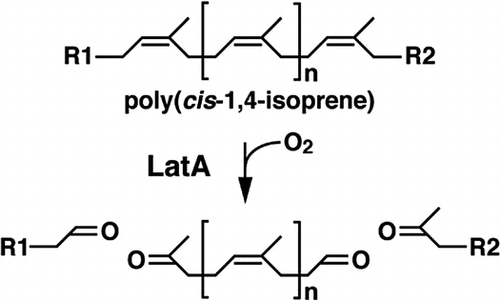
Natural rubber is a biopolymer containing poly(cis-1,4-isoprene) as the main component, and is produced by over 2000 plant species from approximately 300 genera including Hevea brasiliensis (H. brasiliensis).Citation1) Natural rubber from H. brasiliensis is used industrially for tires, seismic isolation rubbers, and medical gloves. The waste of these products has been treated by combustion or landfill processes, which are hazardous to the environment. It is required to find alternative treatment process for rubber waste.
Microbial degradation for natural rubber is thought to be involved in the fate of rubber in the environment and has been reported in Gram-positive bacteria such as Streptomyces, Gordonia, and Nocardia species and Gram-negative bacteria including Xanthomonas, Rhizobacter, and Pseudomonas species.Citation2–6) These rubber degrading bacteria are expected to be used as practical tools for the treatment process of rubber waste. Among the Gram-positive rubber degraders, it has been reported that the latex clearing protein (Lcp) is responsible for initial oxidative cleavage of poly(cis-1,4-isoprene) in Streptomyces sp. strain K30.Citation7) Lcp of K30 is a b-type cytochromeCitation8) and cleaves poly(cis-1,4-isoprene) into low-molecular weight products containing tetra-isoprenoid with aldehyde and keto end groups.Citation9) The resulting compounds are estimated to be further degraded into the corresponding acids by an oxidoreductase, OxiAB, which is encoded by the oxiAB genes, before entering β-oxidation pathway.Citation7,10) Recently, Yikmis and co-workers revealed that the transcription of lcp is increased in the presence of latex or synthetic poly(cis-1,4-isoprene), and Lcp is secreted via the twin-arginine translocation (Tat) system.Citation11) The lcp orthologs were found in Nocardia and Gordonia species and reported to be involved in the poly(cis-1,4-isoprene) degradation in these actinomycetes.Citation2,3,12,13) Hiessl and co-workers indicated that Lcp1 of Gordonia polyisoprenivorans VH2 is included in the copper-containing white laccase family unlike Lcp of K30.Citation14)
In the Gram-negative rubber degrader, an alternative rubber oxygenase (RoxA) encoded by the roxA gene was identified in Xanthomonas sp. strain 35Y.Citation15) RoxA is an extracellular c-type cytochrome with two heme groups, and the heme on the N-terminal side is estimated to constitute an active site surrounded by a cavity with hydrophobic residues.Citation16) It cleaves the carbon–carbon double bonds of poly(cis-1,4-isoprene) by incorporation of an oxygen molecule to produce a tri-isoprenoid with terminal aldehyde and keto end groups.Citation6,15,17,18) Five roxA orthologs were identified in the myxobacteria genomes, including Haliangium, Myxococcus, Corallococcus, and Chondromyces species and three of the orthologs restored rubber degradation activity in the 35Y mutant strain.Citation19) The low molecular weight products with aldehyde and keto end groups, which are generated by RoxA in Gram-negative bacteria, are assumed to be degraded through the same pathway as Gram-positive bacteria.Citation19)
Previously, a Gram-negative rubber degrader, Rhizobacter gummiphilus (R. gummiphilus) NS21 belongs to Betaproteobacteria has been isolated.Citation4,20) Strain NS21 formed a clearing zone to produce aldehydes on a latex-overlay agar medium and depolymerized poly(cis-1,4-isoprene). However, the genes responsible for the rubber degradation in Betaproteobacteria have never been identified. In the present study, putative rubber oxygenase gene was isolated and the involvement of this gene in rubber degradation was examined. These studies revealed that the rubber oxygenase which belongs to c-type cytochrome is involved in the rubber degradation of R. gummiphilus NS21.
Materials and methods
Bacterial strains, plasmids, and culture conditions
The strains and plasmids used in this study are shown in Table . R. gummiphilus NS21 and its mutant derivatives were cultivated at 30 °C in Wx minimal salt mediumCitation21) containing 10 mM glutamate (WxG) or 0.2× Luria-Bertani (LB) medium. Growth on natural rubber was examined using natural rubber overlay agar plates. To prepare the natural rubber overlay agar plates, WxG agar medium containing 1.5% agar was used. The same agar medium containing deproteinized natural rubber (DPNR)Citation22) at a final concentration of 0.4% (v/v) was overlaid to form a thin layer on a solid WxG agar medium. Methylibium petroleiphilum (M. petroleiphilum) PM1 was grown on 0.2× LB or Wx minimal salt medium containing 10 mM succinate at 30 °C. Escherichia coli (E. coli) strains were grown in LB medium at 37 °C. If necessary, ampicillin (Ap), kanamycin (Km), and sucrose were added to the medium at the concentrations of 100 mg/liter, 25 mg/liter, and 10% (w/v), respectively.
Table 1. Strains and plasmids used in this study.
DNA manipulations and nucleotide sequencing
DNA manipulations including total DNA isolation and electroporation, and nucleotide sequencing were performed as described in the previous study.Citation23) Nucleotide and amino acid sequence analyses were performed as previously described.Citation24) The Tat signal sequences were predicted using the TatP 1.0 software (http://www.cbs.dtu.dk/services/TatP/).Citation25)
Construction of the Tn5 insertion mutants and cloning of the latA gene
The Tn5 insertion mutant library of NS21 was generated using pSUP5011, which was conjugally transferred from E. coli S17-1 to NS21 through mating. Transformants were selected on 0.2× LB containing Km. A mutant that did not form a clearing zone on DPNR agar medium was selected from the transformants. The total DNA was extracted from the mutant, digested by SalI, and self-ligated. The resulting DNA was employed as a template to amplify the Tn5-inserted sequence using an inverse PCR technique as well as the primer pair IS50R (5′-AGGTTCCGTTCAGGACGC-3′) and SalI Tn5L (5′-GGAGTTCTACCGGCAGTGC-3′). The amplified fragment was used as a probe for colony hybridization to isolate the flanking region of the Tn5 insertion site from the genomic library of NS21, which was constructed using Charomid 9-36 with SacI digests of the NS21 total DNA. Colony and Southern hybridization experiments were performed using the digoxigenin system (Roche, Mannheim, Germany).
Gene disruption
The latA gene was disrupted by deleting its central region. The fragments containing the N-terminal and C-terminal sequences were connected and inserted in a vector, pK18mobsacB, to generate pDla1 as a latA disruption plasmid. The resulting plasmid was conjugally transferred from E. coli S17-1 to NS21, and the transformants were selected using Km resistance and sucrose-sensitivity on Km- and sucrose-containing 0.2× LB agar plates. The Km-resistant sucrose-sensitive transformants were subjected to a second selection on a sucrose-containing 0.2× LB agar plate to obtain the disrupted mutant using the sacB counterselection system as described previously.Citation26,27)
Rubber degradation assay
To detect the aldehyde groups of the products formed on a DPNR-overlay agar medium, the agar plates were stained with Schiff’s reagent (Wako Pure Chemical Industries, Ltd., Osaka, Japan) for 30 min. After the reagent was removed, non-specific staining was decolorized with 10% (w/v) sodium hydrogen sulfite solution. Gel permeation chromatography (GPC) analysis was employed to examine DPNR depolymerization. The cells of NS21 or the latA mutant were inoculated into 100 ml of WxG medium containing 0.4% (v/v) DPNR and incubated at 30 °C for 7 days. The culture was extracted with pentane, dried in vacuo, and dissolved in 2 ml of tetrahydrofuran. After the solution was filtered with a polytetrafluoroethylene membrane filter (0.2-μm pore size), it was subjected to GPC analysis as previously described.Citation4)
Quantitative reverse transcription-PCR (qRT-PCR) analysis
The NS21 cells were grown on a DPNR-overlay agar medium or WxG agar medium at 30 °C for 3 days. They were collected using centrifugation and washed with 0.9% NaCl. The total RNA was extracted from the cells with ISOGEN II (Nippon Gene Co., Ltd., Tokyo, Japan) according to the manufacturer’s instructions and was treated with RNase-free DNase I (Roche). Single-stranded cDNA was synthesized from 1 μg of total RNA with 100 U of ReverTra Ace reverse transcriptase (Toyobo, Osaka, Japan) and random hexamer primers (Takara Bio Inc., Otsu, Japan) in a 30-μl reaction mixture from ReverTra Ace kit. The cDNA was subjected to qRT-PCR using the StepOne Plus real-time PCR system (Life Technologies, Gaithersburg, Md.). The latART_F (5′-GAAGGCATGGCGTCGTACA-3′) and latART_R (5′-CTGCACCGCCTCGTTGTT-3′) primer pairs used for the qRT-PCR analyses were designed using the Primer Express version 3.0 software program (Life Technologies). Real-time PCR was performed using 50 ng of a cDNA sample, 4 pmol of gene-specific primers, and 10 μl of Fast SYBR Green Master Mix (Life Technologies) in a total reaction volume of 20 μl. The thermal cycling conditions were as follows: 15 s at 95 °C followed by 40 cycles of 15 s at 95 °C and 1 min at 60 °C. To normalize the quantity of RNA in each sample, the 16S rRNA gene was used as an internal standard. Each sample was measured in triplicate, and the means and standard deviations were calculated.
Nucleotide sequence accession number
The nucleotide sequences reported in this study have been deposited in the DDBJ, EMBL, and GenBank nucleotide sequence databases under the accession number, LC073705.
Results
Identification of the rubber degradation gene in strain NS21
Tn5 mutagenesis was performed to obtain a random insertion of Tn5 in the NS21 genome. A Tn5-delivery plasmid pSUP5011, which cannot replicate and is not maintained in NS21, was introduced into NS21. Three thousand Km resistant colonies were screened against formation of clearing zones on a DPNR-overlay agar medium and aldehyde production from DPNR. One mutant strain, NS5-171, did not form a clearing zone and did not produce aldehydes (data not shown), suggesting insertion of a transposon in the gene responsible for rubber degradation in NS21. The flanking region of the Tn5 insertion in NS5-171 was acquired by inverse PCR and used as a probe for southern hybridization with restriction fragments of the NS21 wild-type genomic DNA, indicating that the Tn5-insert region in NS5-171 is in the 7.4-kb SacI fragment (data not shown). The same probe was used in colony hybridization to obtain the plasmid pCSc7 carrying the 7.4-kb SacI fragment from the NS21 genomic library. The nucleotide sequence of the 7.4-kb SacI fragment in pCSc7 was determined, and an open reading frame (ORF) of 2040 bp was found to encode a 73,993-Da polypeptide, which has 36% identity with the roxA-coding rubber oxygenase (RoxA) of Xanthomonas sp. strain 35Y (accession No. AGT20506). The nucleotide sequence of the fragment amplified from the NS5-171 DNA through inverse PCR was determined and indicates that the Tn5 insert was localized between nucleotides 427 and 428 of this ORF. This ORF appeared to code for a rubber oxygenase and was designated as latA. In the 7.4-kb SacI fragment, fifteen complete ORFs coding for more than 200 amino acid residues were identified, but a homology search suggested that no other ORFs than latA have identities with genes coding for rubber degradation enzymes including rubber oxygenases and enzymes for β-oxidation of fatty acids.
Expression of latA in M. petroleiphilum PM1
To examine the activity of the latA gene product, latA was inserted downstream of the Pm promoter in the broad-host-range RK2-derived vector plasmid, pJB861. The resulting plasmid pJla27, which contains a 2.7-kb NotI fragment carrying latA, was introduced into a host strain, M. petroleiphilum PM1, which is unable to degrade DPNR. The PM1 cells harboring pJla27 were grown for 4 days on a DPNR-overlay agar medium plate containing 10 mM succinate, and the plate was subjected to staining with Schiff’s reagent to detect aldehyde formation. Development of a bright red color on the plate after staining indicates aldehyde formation (Fig. (A)). However, clearing zones around the colonies were not observed. When the PM1 cells harboring the empty vector pJB861 were grown on a plate of the same agar medium, no development of red color was observed after staining. It is suggested that the latA gene product is responsible for the formation of a product with aldehydes from poly(cis-1,4-isoprene), which is a major component of DPNR. Based on the proposed cleavage reaction by rubber oxygenases presented in Fig. (B), poly(cis-1,4-isoprene) is likely converted into products with a terminal aldehyde and ketone groups.Citation6,9,15,17)
Figure 1. Expressions of latA in M. petroleiphilum PM1.
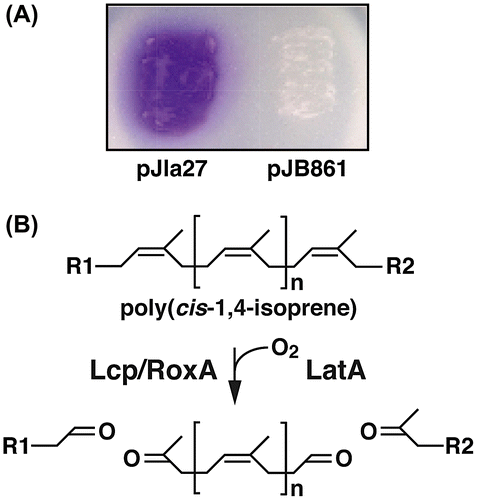
Disruption of the latA gene
The latA gene was deleted using the gene replacement technique with the latA disruption plasmid, pDla1, to examine the role of latA in the degradation of DPNR. The resulting latA mutant strain, DPA7 completely lost its ability to form a clearing zone on a DPNR-overlay agar medium (Fig. (A)). When the same agar medium was stained with Schiff’s regent after the growth of DPA7 on it, no development of red color was observed (Fig. (A)). To confirm whether the deficiency of DPNR degradation was due to the deletion of latA, pJla27 was introduced into the DPA7 cells. Complementation of DPA7 with latA restored the ability to form a clearing zone and generate a product with an aldehyde group on a DPNR-overlay agar medium (Fig. (B)).
Figure 2. The degradation of DPNR by NS21, DPA7, and DPA7 harboring pJla27 or pJB861.
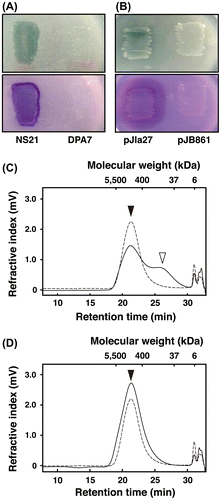
To examine the latA participation in DPNR depolymerization, DPNR degradation by the NS21 and DPA7 cells was analyzed using GPC. When the NS21 cells were incubated with DPNR for 7 days, a peak around 1300 kDa, which was derived from DPNR, decreased, and a peak around 110 kDa increased (Fig. (C)). In contrast, the DPA7 cells neither reduced the peak around 1300 kDa nor increased the peak at 110 kDa during the 7 days of incubation (Fig. (D)). These results indicate that latA is essential for depolymerization and conversion of poly(cis-1,4-isoprene) into a product with an aldehyde group in NS21.
Transcriptional analysis of latA
To investigate the transcriptional induction of latA, qRT-PCR was performed using a primer set designed to amplify an internal region of latA. The total RNA was extracted from the NS21 cells grown on a DPNR overlay or WxG agar media. The mRNA level of latA (14.7 ± 2.8 × 10−7 [mRNA/16S rRNA]) was elevated 4.0-fold in the cells grown on DPNR, indicating that latA transcription is induced during growth in the presence of DPNR.
Discussion
In this study, the rubber degradation gene latA from R. gummiphilus NS21 was identified. It has been reported that Lcp of K30 is exported by a Tat-dependent pathway.Citation11) The Tat-dependent pathway is involved in transportation of folded proteins in bacteria, archaea, and plants,Citation28) and is responsible for secretion of the redox proteins carrying catalytic metal ions or cofactors.Citation29) To find Tat signal sequence in LatA, the TatP 1.0 Server program was used. It estimated a putative Tat signal sequence in LatA, which is composed of 31 amino acid residues, MSSQHPWAPARRLSMVLLLGLSAGLAASAQA. The latA mutant neither formed clearing zones nor produced a product with an aldehyde group on a DPNR-overlay agar medium. It was unable to depolymerize DPNR. Thus, the data strongly suggest that latA codes for an extracellular rubber oxygenase in NS21 that is secreted by a Tat-dependent pathway and is responsible for DPNR depolymerization to produce a product with an aldehyde group.
The results from Schiff’s staining a DPNR-overlay agar medium after growth of M. petroleiphilum PM1 expressing latA indicated the accumulation of a product with an aldehyde group. However, no clearing zones around the colonies were observed. The reaction product formed from poly(cis-1,4-isoprene) by LatA appears to be compounds with terminal aldehyde and ketone groups, as indicated by reports on RoxA and Lcp.Citation6,9,15,17) A lack of clearing zones is consistent with the report by Birke and Jendrossek, which suggested that clearing zone formation requires uptake, consumption, and further degradation of products formed from poly(cis-1,4-isoprene).Citation9)
Recently, rubber-oxygenase orthologs were identified in the genomes of myxobacteria including Haliangium ochraceum (H. ochraceum), Myxococcus fulvus (M. fulvus), Corallococcus coralloides (C. coralloides), and Chondromyces apiculatus (C. apiculatus).Citation2) As shown in Fig. , the phylogenetic tree of LatA, RoxA, and their orthologs shows that these enzymes were distinctly separated into two groups, which are represented by LatA and RoxA, respectively. Two rubber oxygenase paralogs were identified in H. ochraceum DSM 14365 and C. coralloides DSM 2259, and three paralogs were identified in C. apiculatus DSM 436. Interestingly, each of these three strains includes one paralog from the LatA group and one from the RoxA group. Although activities of the three RoxA orthologs in the RoxA group were studied by Birke et al.Citation19), activities of the LatA orthologs in the LatA group have not been investigated.
Figure 3. Phylogenetic tree of LatA, RoxA, and their orthologs.
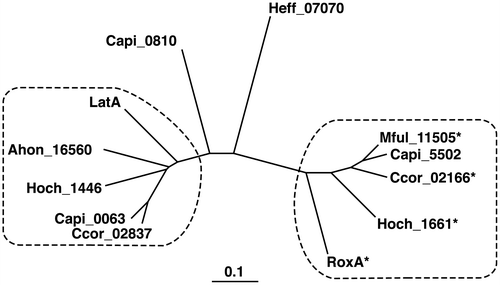
LatA, RoxA, and their orthologs exhibited conservation of two heme binding motifs (CXXCH), which are residues 191–195 and residues 390–394 in RoxA (Fig. ). H641 in RoxA, which is estimated to be the distal axial ligand to the second heme ion (heme 2), is conserved among both the RoxA and LatA group enzymes. Further studies indicate that F317 in RoxA, which is the closest residue to the distal side of the first heme ion (heme 1), is involved in binding of oxygen molecule.Citation16,30) This residue is completely conserved among LatA, RoxA, and their orthologs, which supports the nation that it plays an essential role in the enzyme reaction. The residues A251, I252, L254, I255, F301, and A316 in RoxA form a hydrophobic cavity surrounding the putative active site on the distal side of heme 1.Citation16) All of these residues, except L254 and I255, are conserved among the RoxA group orthologs. L254 and I255 are substituted by the hydrophobic residues I and V, respectively. Among the LatA-group enzymes, I252, L254, and F301 in RoxA are conserved, but A251, I255, and A316 are substituted by the residues D, A, and V, respectively. All of the residues that correspond to I252, L254, I255, F301, and A316 in RoxA are hydrophobic, suggesting that these residues form a hydrophobic cavity in LatA-group enzymes.
Figure 4. Alignments of the deduced amino acid sequences of LatA, RoxA, and their orthologs.
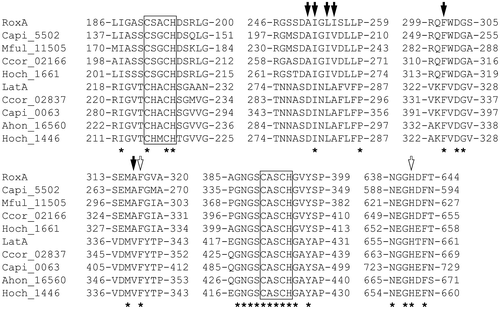
A transcriptional analysis of latA revealed that DPNR or its intermediate metabolite induces latA transcription. A putative transcriptional regulator gene is located immediately upstream from latA. The deduced amino acid sequence of this gene product showed 26–30% identity with those of the LysR-type transcriptional regulators from Bacillus subtilis SMY, Synechococcus sp. PCC 6803, and Sinorhizobium meliloti Rm1021.Citation31–33) This gene may be involved in the transcriptional regulation of latA. The transcriptional regulator, promoter element, and transcription start site for latA as well as the uptake system for the intermediate metabolite is expected to clarify the transcriptional regulatory mechanism of the latA gene in NS21.
Author contributions
Daisuke Kasai and Shunsuke Imai contributed equally to this work. Daisuke Kasai, Shunsuke Imai, and Masao Fukuda designed the study and wrote the manuscript. Daisuke Kasai, Shunsuke Imai, Shota Asano, and Michiro Tabata performed the experiments and analyzed the data. So Iijima, Naofumi Kamimura, and Eiji Masai helped to interpret the data and discussed the results. All authors reviewed the paper.
Disclosure statement
No potential conflict of interest was reported by the authors.
Funding
This research was partly supported by the Japan Science and Technology Agency/Japan International Cooperation Agency, Science and Technology Research Partnership for Sustainable Development (JST/JICA, SATREPS) and JST Adaptable and Seamless Technology Transfer Program through Target-driven R&D (JST A-STEP). This study was also funded by Japan Society for the Promotion of Science (JSPS) KAKENHI, [grant number 15H05639], [grant number 16K12631].
Acknowledgments
We are indebted to Prof. Dr. Seiichi Kawahara of Nagaoka University of Technology for provision of latex and DPNR.
References
- Backhaus RA. Rubber formation in plants – a minireview. Israel J Bot. 1985;34:283–293.
- Bröker D, Dietz D, Arenskötter M, et al. The genomes of the non-clearing-zone-forming and natural-rubber-degrading species Gordonia polyisoprenivorans and Gordonia westfalica harbor genes expressing Lcp activity in Streptomyces strains. Appl Environ Microbiol. 2008;74:2288–2297.10.1128/AEM.02145-07
- Ibrahim EM, Arenskötter M, Luftmann H, et al. Identification of Poly(cis-1,4-isoprene) degradation intermediates during growth of moderately thermophilic actinomycetes on rubber and cloning of a functional lcp homologue from Nocardia farcinica strain E1. Appl Environ Microbiol. 2006;72:3375–3382.10.1128/AEM.72.5.3375-3382.2006
- Imai S, Ichikawa K, Muramatsu Y, et al. Isolation and characterization of Streptomyces, Actinoplanes, and Methylibium strains that are involved in degradation of natural rubber and synthetic poly(cis-1,4-isoprene). Enzyme Microb Technol. 2011;49:526–531.10.1016/j.enzmictec.2011.05.014
- Linos A, Reichelt R, Keller U, et al. A Gram-negative bacterium, identified as Pseudomonas aeruginosa AL98, is a potent degrader of natural rubber and synthetic cis-1,4-polyisoprene. FEMS Microbiol Lett. 2000;182:155–161.10.1111/fml.2000.182.issue-1
- Jendrossek D, Reinhardt S. Sequence analysis of a gene product synthesized by Xanthomonas sp. during growth on natural rubber latex. FEMS Microbiol Lett. 2003;224:61–65.10.1016/S0378-1097(03)00424-5
- Rose K, Tenberge KB, Steinbüchel A. Identification and characterization of genes from Streptomyces sp. strain K30 responsible for clear zone formation on natural rubber latex and poly(cis-1,4-isoprene) rubber degradation. Biomacromolecules. 2005;6:180–188.10.1021/bm0496110
- Birke J, Rother W, Jendrossek D. Latex clearing protein (Lcp) of Streptomyces sp. strain K30 Is a b-type cytochrome and differs from rubber oxygenase A (RoxA) in its biophysical properties. Appl Environ Microbiol. 2015;81:3793–3799.10.1128/AEM.00275-15
- Birke J, Jendrossek D. Rubber oxygenase and latex clearing protein cleave rubber to different products and use different cleavage mechanisms. Appl Environ Microbiol. 2014;80:5012–5020.10.1128/AEM.01271-14
- Bode HB, Kerkhoff K, Jendrossek D. Bacterial degradation of natural and synthetic rubber. Biomacromolecules. 2001;2:295–303.10.1021/bm005638h
- Yikmis M, Arenskötter M, Rose K, et al. Secretion and transcriptional regulation of the latex-clearing protein, Lcp, by the rubber-degrading bacterium Streptomyces sp. strain K30. Appl Environ Microbiol. 2008;74:5373–5382.10.1128/AEM.01001-08
- Hiessl S, Schuldes J, Thürmer A, et al. Involvement of two latex-clearing proteins during rubber degradation and insights into the subsequent degradation pathway revealed by the genome sequence of Gordonia polyisoprenivorans strain VH2. Appl Environ Microbiol. 2012;78:2874–2887.10.1128/AEM.07969-11
- Luo Q, Hiessl S, Poehlein A, et al. Insights into the microbial degradation of rubber and gutta-percha by analysis of the complete genome of Nocardia nova SH22a. Appl Environ Microbiol. 2014;80:3895–3907.10.1128/AEM.00473-14
- Hiessl S, Böse D, Oetermann S, et al. Latex clearing protein–an oxygenase cleaving poly(cis-1,4-isoprene) rubber at the cis double bonds. Appl Environ Microbiol. 2014;80:5231–5240.10.1128/AEM.01502-14
- Braaz R, Fischer P, Jendrossek D. Novel type of heme-dependent oxygenase catalyzes oxidative cleavage of rubber (poly-cis-1,4-isoprene). Appl Environ Microbiol. 2004;70:7388–7395.10.1128/AEM.70.12.7388-7395.2004
- Seidel J, Schmitt G, Hoffmann M, et al. Structure of the processive rubber oxygenase RoxA from Xanthomonas sp. Proc Natl Acad Sci USA. 2013;110:13833–13838.10.1073/pnas.1305560110
- Braaz R, Armbruster W, Jendrossek D. Heme-dependent rubber oxygenase RoxA of Xanthomonas sp. cleaves the carbon backbone of poly(cis-1,4-isoprene) by a dioxygenase mechanism. Appl Environ Microbiol. 2005;71:2473–2478.10.1128/AEM.71.5.2473-2478.2005
- Schmitt G, Seiffert G, Kroneck PM, et al. Spectroscopic properties of rubber oxygenase RoxA from Xanthomonas sp., a new type of dihaem dioxygenase. Microbiology. 2010;156:2537–2548.10.1099/mic.0.038992-0
- Birke J, Röther W, Schmitt G, et al. Functional identification of rubber oxygenase (RoxA) in soil and marine myxobacteria. Appl Environ Microbiol. 2013;79:6391–6399.10.1128/AEM.02194-13
- Imai S, Yoshida R, Endo Y, et al. Rhizobacter gummiphilus sp. nov., a rubber-degrading bacterium isolated from the soil of a botanical garden in Japan. J Gen Appl Microbiol. 2013;59:199–205.10.2323/jgam.59.199
- Kasai D, Kamimura N, Tani K, et al. Characterization of FerC, a MarR-type transcriptional regulator, involved in transcriptional regulation of the ferulate catabolic operon in Sphingobium sp. strain SYK-6. FEMS Microbiol Lett. 2012;332:68–75.10.1111/fml.2012.332.issue-1
- Chaikumpollert O, Yamamoto Y, Suchiva K, et al. Protein-free natural rubber. Colloid Polym Sci. 2012;290:331–338.10.1007/s00396-011-2549-y
- Masai E, Yamada A, Healy JM, et al. Characterization of biphenyl catabolic genes of gram-positive polychlorinated biphenyl degrader Rhodococcus sp. strain RHA1. Appl Environ Microbiol. 1995;61:2079–2085.
- Kasai D, Fujinami T, Abe T, et al. Uncovering the protocatechuate 2,3-cleavage pathway genes. J Bacteriol. 2009;191:6758–6768.10.1128/JB.00840-09
- Bendtsen JD, Nielsen H, Widdick D, et al. Prediction of twin-arginine signal peptides. BMC Bioinf. 2005;6:167.10.1186/1471-2105-6-167
- Sharp JO, Sales CM, LeBlanc JC, et al. An inducible propane monooxygenase is responsible for N-nitrosodimethylamine degradation by Rhodococcus sp. strain RHA1. Appl Environ Microbiol. 2007;73:6930–6938.10.1128/AEM.01697-07
- van der Geize R, Hessels GI, van Gerwen R, et al. Unmarked gene deletion mutagenesis of kstD, encoding 3-ketosteroid Δ1-dehydrogenase, in Rhodococcus erythropolis SQ1 using sacB as counter-selectable marker. FEMS Microbiol Lett. 2001;205:197–202.10.1016/S0378-1097(01)00464-5
- Yen MR, Tseng YH, Nguyen EH, et al. Sequence and phylogenetic analyses of the twin-arginine targeting (Tat) protein export system. Arch Microbiol. 2002;177:441–450.10.1007/s00203-002-0408-4
- Lee PA, Tullman-Ercek D, Georgiou G. The bacterial twin-arginine translocation pathway. Annu Rev Microbiol. 2006;60:373–395.10.1146/annurev.micro.60.080805.142212
- Birke J, Hambsch N, Schmitt G, et al. Phe317 is essential for rubber oxygenase RoxA activity. Appl Environ Microbiol. 2012;78:7876–7883.10.1128/AEM.02385-12
- Bohannon DE, Sonenshein AL. Positive regulation of glutamate biosynthesis in Bacillus subtilis. J Bacteriol. 1989;171:4718–4727.10.1128/jb.171.9.4718-4727.1989
- MacLean AM, Anstey MI, Finan TM. Binding site determinants for the LysR-type transcriptional regulator PcaQ in the legume endosymbiont Sinorhizobium meliloti. J Bacteriol. 2008;190:1237–1246.10.1128/JB.01456-07
- Omata T, Gohta S, Takahashi Y, et al. Involvement of a CbbR homolog in low CO2-induced activation of the bicarbonate transporter operon in cyanobacteria. J Bacteriol. 2001;183:1891–1898.10.1128/JB.183.6.1891-1898.2001
- Nakatsu CH, Hristova K, Hanada S, et al. Methylibium petroleiphilum gen. nov., sp. nov., a novel methyl tert-butyl ether-degrading methylotroph of the Betaproteobacteria. Int J Syst Evol Microbiol. 2006;56:983–989.10.1099/ijs.0.63524-0
- Hanahan D. Studies on transformation of Escherichia coli with plasmids. J Mol Biol. 1983;166:557–580.10.1016/S0022-2836(83)80284-8
- Simon R. High frequency mobilization of gram-negative bacterial replicons by the in vitro constructed Tn5-Mob transposon. Mol Gen Genet. 1984;196:413–420.10.1007/BF00436188
- Short JM, Fernandez JM, Sorge JA, et al. λ ZAP: a bacteriophage λ expression vector with in vivo excision properties. Nucleic Acids Res. 1988;16:7583–7600.10.1093/nar/16.15.7583
- Saito I, Stark GR. Charomids: cosmid vectors for efficient cloning and mapping of large or small restriction fragments. Proc Natl Acad Sci USA. 1986;83:8664–8668.10.1073/pnas.83.22.8664
- Schäfer A, Tauch A, Jäger W, et al. Small mobilizable multi-purpose cloning vectors derived from the Escherichia coli plasmids pK18 and pK19: selection of defined deletions in the chromosome of Corynebacterium glutamicum. Gene. 1994;145:69–73.10.1016/0378-1119(94)90324-7
- Blatny JM, Brautaset T, Winther-Larsen HC, et al. Improved broad-host-range RK2 vectors useful for high and low regulated gene expression levels in gram-negative bacteria. Plasmid. 1997;38:35–51.10.1006/plas.1997.1294