Abstract
Oil-in-water (O/W) emulsions are among the dispersion systems commonly used in food, and these emulsions are in thermodynamically unstable or metastable states. In this paper, various methods for preparing O/W emulsions are outlined. Since the commodity value of food is impaired by the destabilization of O/W emulsions, experimental and theoretical approaches to assess the stability of O/W emulsions are overviewed, and factors affecting the dispersion stability of emulsions are discussed based on the DLVO theory and the concept of the stability factor. The oxidation of lipids in O/W emulsions is unhealthy and gives rise to unpleasant odors. Factors affecting the autoxidation of lipids are discussed, and theoretical models are used to demonstrate that a reduction of the oil droplet size suppresses or retards autoxidation. Microencapsulated lipids or oils exhibit distinct features in the oxidation process. Models that explain these features are described. It is demonstrated that a reduction in the oil droplet size is also effective for suppressing or retarding the oxidation of microencapsulated oils.
Graphical abstract
Methods for preparing O/W emulsions, experimental and theoretical approaches to assess the stability of O/W emulsions, and factors affecting the oxidative stability of lipid in O/W emulsion and microencapsulated systems are described.
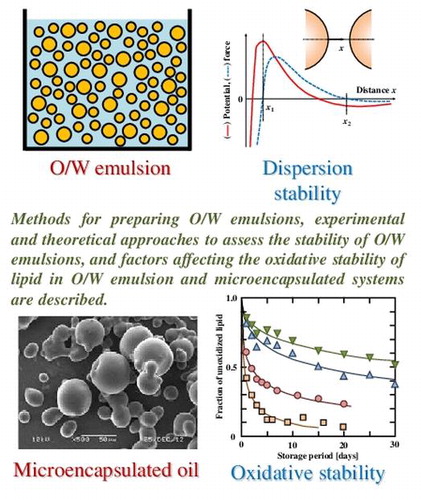
Food is a multicomponent system consisting of water, carbohydrates, proteins, lipids, etc. that forms many types of heterogeneous and homogeneous dispersion systems. A dispersion system consisting of two immiscible liquid phases is designated as an emulsion, and it comprises the dispersion and continuous phases. In oil-in-water (O/W) emulsions, small oil droplets are dispersed in the water phase, while small water droplets are dispersed in an oil phase in water-in-oil (W/O) emulsions. Milk, cream, and dressing are examples of O/W emulsions, and butter and margarine are W/O emulsions.Citation1)
Multiple emulsions, such as oil-in-water-in-oil and water-in-oil-in-water emulsions are used industrially. However, the use of multiple emulsions in the food industry is limited, and their application deserves to be explored further.Citation2,3)
Food O/W emulsions are destabilized by creaming, aggregation or flocculation, coalescence, and oil–water separation during the distribution and storage processes, and this destabilization results in the lowering or loss of the commodity value. Oxidation of lipids in emulsions generates rancidity, also impairing commodity value. In some cases, the products of oxidation can have harmful health effects.
In this paper, attention is confined to O/W emulsions. Methods for preparing O/W emulsions are surveyed, and investigations of factors affecting dispersion and oxidative stability are overviewed. Theories on dispersion and oxidative stability are discussed and their use is introduced.
Both chemical and physical methods are known for the prevention or suppression of lipid oxidation. The use of an antioxidant is an example of a chemical method, while microencapsulation is a physical method. The reasons for retardation or suppression of lipid oxidation will also be discussed.
I. Preparation of O/W emulsions
I.i. Emulsification methods
Mixers, stirrers, and rotor-stator homogenizers have been used for many years to prepare O/W emulsions. The emulsification methods are applicable for preparation of the emulsions even with moderately or highly viscous continuous phase. Rotor-stator homogenizers consist of a fast-spinning inner rotor with a stationary outer sheath (stator), and homogenize the sample through mechanical tearing, shear fluid forces, and/or cavitation. Emulsification using the equipment is usually a batch process, but colloid mills, which are homogenizers of the rotor-stator type, can prepare O/W emulsions continuously.
In high-pressure homogenizers, high pressure is applied to a sample that is passed through a narrow gap. Upon passing through the gap, the pressure decreases precipitously to atmospheric pressure, generating a large shear stress, which results in emulsification. The attainable pressure was 10–40 MPa in the 1990s, but it has improved to 350 MPa, up to 800 MPa in the 2000s.Citation4) The high-pressure homogenizer can re-disperse protein aggregates. Since it produces very stable emulsions, it is widely used in the food industry.Citation4)
The principle of ultrasonic emulsification is unlike that of traditional and high-pressure homogenization, and is based on the cavitation generated by ultrasound irradiation. Although the ultrasonic emulsification has been used industrially due to large energy output, its operation is batch-wise and its throughput is low.Citation5)
Membrane emulsification is a relatively new method, which was proposed at the beginning of 1990s.Citation6) In the direct membrane emulsification method, the phase to be dispersed, which is oil in the case of an O/W emulsion, is passed through a porous membrane into the continuous phase (aqueous phase in an O/W emulsion) to produce emulsions having small droplets. In the premix membrane emulsification technique, a coarse O/W emulsion is treated with the membrane. Membrane emulsification has the advantages of narrow distribution of oil droplet size (high monodispersity) and low energy consumption.
Microchannel emulsification is another relatively new method proposed in the middle of the 1990s, and can produce highly monodisperse emulsions with low energy consumption.Citation7) This method utilizes microchannel array plates made of single-crystal silicon, in place of the membrane in membrane emulsification. The driving force for microchannel emulsification is the difference in interfacial tension between the microchannel and the well where the dispersion phase is mixed with the continuous phase.Citation7)
The emulsification methods mentioned above are categorized as physical methods, while phase-inversion emulsification is a chemical method and consumes low energy. O/W emulsions can be inverted to W/O emulsions or vice versa by changing the hydrophile-lipophile balance of the surfactant, by modifying the temperature and concentration of electrolytes, or the volumetric fraction of the dispersion phase.Citation8)
I.ii. Oil droplet size and energy efficiency
Emulsification is a process getting interfacial energy. Therefore, more energy should be applied to prepare emulsions with larger specific surface area by small droplets. However, it should be noted that the energy used up for emulsification is not entirely for this purpose alone. Traditional mechanical homogenization methods consume a lot of energy due to low efficiency, and produce O/W emulsions with oil droplet size of 0.5–100 μm. Although the high-pressure homogenization method also consumes much energy and its efficiency is low, the oil droplet size is usually hundreds of nanometers.Citation8) These emulsification methods have been widely used in many industries, such as food and cosmetics. On the other hand, membrane emulsification can produce O/W emulsions with oil droplet diameters of a few micrometers, with low energy consumption due to high efficiency.Citation8) The energy consumed in microchannel emulsification is less than in membrane emulsification,Citation6) and the energy efficiency can reach ~0.65.Citation7) Recently, it has been reported that phase inversion temperature emulsification produces O/W emulsions with a relatively narrow distribution, and oil droplet diameters of a few dozen micrometers.Citation9)
Introduction of a parameter called energy density,Citation10) which corresponds to the above-mentioned energy output, enables us to quantitatively assess the relationship between oil droplet size and the energy necessary for emulsification.Citation11) The energy density is defined as the energy consumed per unit volume during emulsification. Because the energy density is expressed in pressure units, it can also be regarded as the pressure applied during high-pressure and membrane emulsification.
From the plots of the energy density against the Sauter mean diameter, d32, of oil droplets, we can estimate that a smaller d32 at a specific energy density is indicative of higher energy efficiency. Therefore, the energy density is a useful parameter to compare different emulsification methods. When the energy efficiency is defined as the ratio of interfacial energy obtained during emulsification, which can be estimated from the d32 value, to the energy consumed during emulsification, it can be used for the quantitative comparison of different emulsification methods.Citation12) The energy efficiency was correlated with the energy density for membrane and high-pressure emulsification methods (Fig. ). As mentioned above, the energy efficiency in membrane emulsification is higher than that of high-pressure emulsification.
II. Experimental examination on the dispersion stability of O/W emulsions
II.i. Effect of oil droplet size on the stability
Creaming, flocculation, coalescence, phase-inversion, and Ostwald ripening are examples of physical destabilization of emulsions. The rate of Ostwald ripening in an O/W emulsion is proportional to the solubility of the oil in the aqueous phase.Citation13) The Ostwald ripening is not involved in destabilization of O/W emulsion consisted of two immiscible phases such as triacylglycerol and water, and the destabilization is progressive in the order of creaming, flocculation and coalescence of oil droplets. Creaming occurs due to the difference in density, Δρ, between the continuous and dispersion phases, and the creaming velocity, v, can be calculated by Equation (1) in a dilute emulsion system.
(1)
where d is the oil droplet diameter, g is the gravitational acceleration, and η is the viscosity of the continuous phase. Equation (1) indicates that reductions of Δρ and d are effective for suppressing the creaming as well as increase of η, especially, reduction of oil droplet size, d, would be most effective. Practically, commercially prepared milk is homogenized to decrease the oil droplet size to <1 μm, in order to improve the dispersion stability during distribution. In emulsions with oil droplet diameters in the order of nanometers, the rate of diffusion due to Brownian motion is higher than that of creaming. Such emulsions are very stable against creaming,Citation14) and exhibit no change in the oil droplet distribution for a few months or longer.Citation15)
II.ii. Factors affecting the dispersion stability
Various components can be added to food O/W emulsions for seasoning, addition of favorable texture, creation of functionality, improvement of dispersion or oxidative stability, and elongation of storage. The interaction of these ingredients affects the dispersion stability of the emulsion. Thickening agents are widely used to improve the dispersion stability of emulsions, as well as to improve texture. Appropriate use of thickening agents suppresses creaming by increasing the viscosity of the continuous phase or by the formation of a gel network.Citation16,17) Opposite effects are also recognized depending on the concentration of the thickening agent.
Electrically charged polymers such as ι-carrageenan are adsorbed onto emulsifier molecules with opposite charge, on the interface of oil and aqueous phases. Addition of a small amount of polymer destabilized O/W emulsions, as they promoted the flocculation of oil droplets by bridging. When a large amount of polymer was added to an O/W emulsion, the polymer covered the whole surface of the oil droplet to increase the repulsive force operating between two adjacent oil droplets, and stabilized the contiguous oil droplets.Citation18,19) Electrically neutral polymers are not adsorbed onto the interface between the oil and aqueous phases, and exist in the aqueous phase. If the polymer cannot penetrate into the space between two adjacent oil droplets, a difference in osmotic pressure between this space and the aqueous phase is generated, which acts as a driving force to coalesce the oil droplets. This is called the depletion effect.Citation18)
The above-mentioned bridge flocculation and depletion effects are also observed in emulsions stabilized by proteins.Citation17) Proteins from foods such as milk, egg, and soybean, are used as emulsifiers and stabilizers. As changes in the shape and electric charge of the protein can raise or lower its isoelectric point, the pH also affects the dispersion stability of emulsions containing proteins.Citation18,20)
Salt can be added to food emulsions as seasoning or as a preservative. An increase in the ionic strength of the continuous phase lowers the dispersion stability either by shielding the electric charge of the protein or by changing the ζ-potential of the oil droplets. The effect of ionic strength on the dispersion stability can be qualitatively understood based on the DLVO theory (see section 3.1). However, the addition of salt enhances the surface activity of egg yolk.Citation21)
Competitive adsorption of low molecular emulsifiers (surfactants) and proteins at the interface affects the dispersion stability of the emulsion.Citation22,23) Similarly, low molecular substances generated through lipid oxidation can destabilize O/W emulsions.Citation24) Physiologically active substances such as chitosan and catechin also interact with proteins to destabilize O/W emulsions.Citation25,26)
III. Theoretical approaches to dispersion stability
III.i. DLVO theory
A model describing the colloidal stability quantitatively was proposed by Derjaguin, Laudau, Verwey, and Overveek,Citation27) and is called the DLVO theory. The theory has been used to explain phenomena found in colloidal science, adsorption, and many other fields. Thus, it is no exception to discuss the stability of emulsions based on this model.
The theory describes the force between two charged surfaces interacting through the continuous phase, as the sum of the attractive London-van der Waals forces and the electrostatically repulsive Coulombic force. The aggregation or flocculation of oil droplets is described quantitatively. The potential distribution, V, between two particles or oil droplets with the same diameter, d, is approximately expressed by Equation (2) from the Hamaker’s exact equation.
(2)
where A is the Hamaker’s constant, x is the distance or separation between the oil droplets, ε is the dielectric constant of the continuous phase, κ is the Debye’s factor, and ψ0 is the surface potential. For emulsions, ζ-potential is sometimes used instead of the surface potential. The first term on the right-hand side of Equation (2) stems from the electrostatic repulsion and decreases exponentially with an increase in x. The second term represents the van der Waals attraction. The value of the term is infinity at x = 0 (on the surface of oil droplet), and falls in inverse proportion to x. When there is an energy barrier, the potential energy has two inflection points, x1 and x2 (Fig. ). The potential barrier is maximum at x = x1, and a strong attraction operates between the oil droplets at x < x1. At x = x2 (x1 < x2), the potential energy is minimum and the dispersion system is in a metastable state. At this point, where attraction is equal to repulsion, the oil droplets come close to each other to form aggregates. The potential energy approaches zero with increasing x at x > x2. Since flocculation is reversible, the aggregate can be re-dispersed by an extraneous stimulus or thermal energy. When oil droplets gain sufficient energy to overcome the potential barrier, they approach each other at distances shorter than x1. Because of the strong attraction between the oil droplets at x < x1, they cannot be re-dispersed. In such situations, the oil droplets in O/W emulsions coalesce. The DLVO theory, which is expressed by Equation (2), is a simplified and yet effective theory. It is very useful for understanding phenomena observed in food O/W emulsions, such as dispersion, flocculation, and the coalescence of oil droplets.
Fig. 2. Schematics of the distribution of potential (solid curve) and force (broken curve) between two adjacent oil droplets.
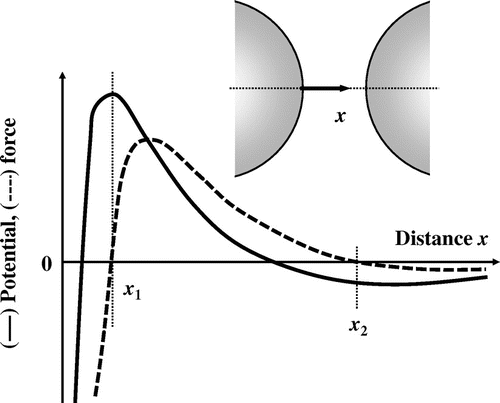
Many oil droplets are dispersed in practical food O/W emulsions, and there is a distribution in the oil droplet size. Since food emulsions have complicated compositions, various interactions other than electrostatic repulsion and van der Waals attraction operate between the oil droplets. Thus, the stability of practical O/W emulsions cannot always be explained by the original DLVO theory. For example, it is empirically known that O/W emulsions with smaller oil droplets are more stable. However, this fact cannot be explained by the original DLVO theory. In this context, the theory has been modified taking into consideration other forces such as the viscous force, hydrogen bonding, hydrophobic interactions, and osmotic pressure.Citation28–Citation32)
III.ii. Stability factor
The DLVO theory assesses the dispersion stability of emulsions based on the height of the energy barrier. This method is applicable only when the energy barrier has a peak. The stability factor, W,Citation27,33) is formulated in terms of the stability and also enables us to discuss it quantitatively.Citation27) The stability factor is defined by the ratio of rate of slow colloidal to fast colloidal aggregation or flocculation (Equation (3)).
(3)
where kB is the Boltzmann constant, and T is the absolute temperature. Fast flocculation is a process formulated by von Smoluchowski, where colloidal particles or oil droplets approach each other exclusively by diffusion. Slow flocculation is a process where a potential barrier based on the DLVO theory exists between the oil droplets. A larger W value indicates that the dispersion system is more stable. The rates for fast and slow flocculation can be obtained experimentally by measuring the change in turbidity of the dispersion system.Citation30,34)
The W value is linearly related to the concentration of the electrolyte on a double logarithmic scale. The Hamaker constant and diffuse potential can be evaluated from the linear relationship.Citation30) However, as in the case of the DLVO theory, the stability factor is also unable to assess the stability of practical dispersion systems. Therefore, a modified stability factor that includes parameters other than electrostatic repulsion has been proposed.Citation30,31)
III.iii. Modified theory incorporating viscous force
The DLVO theory and stability factor are powerful tools and have been modified for a more precise assessment of the stability of practical dispersion systems, by taking into consideration effects other than the van der Waals attraction and electrostatic repulsion. Derjaguin pointed out the drawback of the stability factor in the 1960s.Citation35) As mentioned above, fast flocculation in the stability factor is derived from von Smoluchowski’s diffusion equation. In the derivation, the particles are assumed to diffuse independently. However, it is known that oncoming particles behave differently from isolated particles, and that the effect of viscosity of the continuous phase on the stability should be considered. In this context, Spielman, in 1970, proposed the modified stability factor expressed by Equation (4), in which the viscous force is estimated by the Stokes’ law.
(4)
where D12 is the relative Brownian diffusion coefficient and depends on the viscosity of continuous phase, particle size, and the distance between particles. is the diffusion coefficient that is free from the viscous force due to sufficient separation of the particles.
We proposed a model where the viscous force from the Stokes’ law was combined with the DLVO theory in order to assess the stability of O/W emulsions in a cream layer.Citation28) The force operating on an oil droplet is given by Equation (5), which is obtained by differentiating Equation (2) in terms of the distance or separation x.
(5)
A kinetic equation, which describes the balance of forces operating on the oil droplet is expressed by Equation (6), taking into consideration the viscous force from the Stokes’ law.
(6)
where ρs is the density of the oil droplet, and u (u = dx/dt) is the migration velocity of the oil droplet. As mentioned above, the higher stability of O/W emulsions with smaller oil droplets cannot be explained by the DLVO theory. However, computational calculations based on our modified model revealed this phenomenon. The higher stability for smaller oil droplets indicates that the activation energy is higher for their coalescence.Citation36) The computational simulation showed that the activation energy for oil droplets less than 1 μm in diameter was in the range 10–103 kJ/mol (Fig. ), which is similar to the activation energies of many chemical and physical changes. We can evaluate the rate constant for coalescence of oil droplets from the activation energy. The change in oil droplet size during coalescence was simulated under the assumption that the coalescence obeyed second-order kinetics.Citation11) The simulation indicated that in emulsions containing smaller oil droplets, the distribution of the oil droplet size changed more slowly, that is, the emulsion is more stable. It was also revealed that O/W emulsions with higher monodispersity were more stable.
IV. Lipid oxidation in O/W emulsion
IV.i. Effect of oil droplet size
It is generally noted that the reduction of oil droplet size in O/W emulsions promotes lipid oxidation due to the increase in specific surface area. However, because many factors are related to the oxidation of lipids in O/W emulsions, it is not easy to assess the effect of oil droplet size. Some contradictory results have been reported. Lethauant et al.Citation37) reported that lipids in smaller oil droplets (d32=0.4–8.5 μm) are prone to oxidation due to higher consumption of oxygen and the formation of conjugated dienes. Cercaci et al.Citation38) also showed the increased oxidation in O/W emulsions with small oil droplets (d32=0.4–8.5 μm), based on the measurement of hydroperoxide and hexanol formation. On the other hand, no significant effect of the oil droplet size (d32=0.26–2.6 μm) was observed, as measured by hydroperoxide formation.Citation39) Furthermore, Nakaya et al.Citation40) and Belhaj et al.Citation41) demonstrated a higher stability for smaller oil droplets based on the measurement of hydroperoxide formation, for oil droplets of d32=0.8–9 μm, and the absorbance due to conjugated diene, respectively.
In these reports, oxidation was assessed by different methods, such as hexanol formation, decrease of dissolved oxygen in the aqueous phase, decrease of oxygen in the gas phase (head space), and peroxide values. Different indications for the oxidation rate were also used such as the duration of induction period, half-life, and rate constant for oxidation. In addition to the difference in methods for assessing the oxidation, the emulsification methodCitation42) and composition of the O/W emulsionCitation39) also affected the oxidation. These facts indicate the experimental difficulty in assessing the effect of oil droplet size on the oxidative stability. Thus, a theoretical approach would be helpful to understand the effect, as ideal conditions in which parameters other than the oil droplet size can be fixed, are easily realizable in computational simulations.
The lipid autoxidation rate in O/W emulsions can be described by a rate expression of the autocatalytic type (Equation (7)), where the rate is proportional to the product of the concentrations of the unoxidized and oxidized lipids.Citation43,44)
(7)
where Y is the fraction of unoxidized lipid, t is the time, and k is the rate constant. Integration of Equation (7) under the initial condition of Y = Y0 at t = 0 gives Equation (8).
(8)
where Y0 is a parameter to be introduced for solving Equation (8) and reflects the initial state of the lipid to be oxidized. The plots of ln[(1 − Y)/Y] against t yield a straight line, and the rate constant k and the parameter Y0 are estimated from the slope and the intercept of the line, respectively.
As understood from Equation (7), the lipids in an oil droplet are not oxidized until one lipid molecule is oxidized. Once one lipid molecule is oxidized, the oxidation of the rest of the lipid molecules in the oil droplet cannot be prevented. Since the oil droplets are isolated from each other by the continuous phase and the emulsifier layer on the surface, it is assumed that the oxidized molecules in one oil droplet do not affect the lipid molecules in other oil droplets. Under this assumption, lipid oxidation in O/W emulsions was analyzed stochastically by computer simulations based on Equation (7). The simulation showed that when the size of the oil droplet reduced, the induction period for lipid oxidation is elongated to retard the oxidation.Citation45) Polydispersity in oil droplet size scarcely affected the lipid oxidation process for oil droplets of median diameter ranging between 100 nm and 3 μm.Citation46)
Oil droplets are covered by emulsifier molecules on the interface between the oil and aqueous phases, and the hydrophobic moiety of the emulsifier is anchored to the oil droplet. The hydrophobic moiety is an unoxidizable or hardly oxidizable component such as a saturated acyl group. The reduction of oil droplet size increases the specific surface area of the oil droplet, and the volumetric fraction of the hydrophobic moiety in the oil droplet become large. Anchoring of the hydrophobic moieties into the oil phase dilutes the lipid to be oxidized. This effect was proposed by Nakaya et al.,Citation40) and we described it quantitatively in Equation (9).Citation47)
(9)
where k0 is the rate constant in bulk systems or for large oil droplets, a is the area occupied by the emulsifier molecule, VL is the volume of the hydrophobic moiety of emulsifier, and dH is the thickness of the hydrophilic moiety of the emulsifier adsorbed on the surface of the oil droplet. Equation (9) indicates that lipid oxidation for smaller oil droplets is suppressed or retarded to a greater extent. Simulation for commonly used emulsifiers, such as polyglycerol fatty acid ester, indicated that the suppression or retardation of lipid oxidation upon reducing the oil droplet size became significant for O/W emulsions with oil droplet diameter ≤200 nm (Fig. ).
Fig. 4. Dependence of the rate constant of oxidation on the initial mean diameters of oil droplets. The curve was drawn based on Equation (9). Inset: The oxidation process of methyl linoleate in an O/W emulsion at 40 °C. Initial mean diameters of oil droplets were 43 nm (Δ), 79 nm (○), and 0.92 μm (◊), and the curves were calculated by Equation (8).
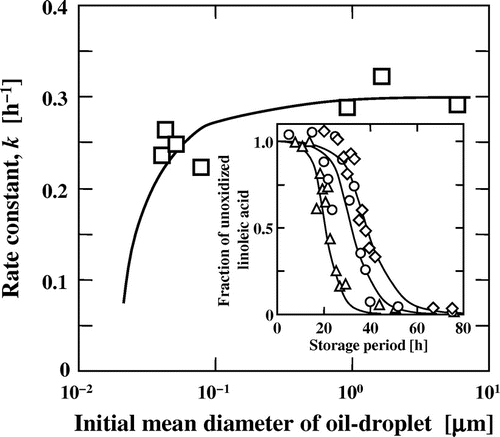
IV.ii. Effect of antioxidant
Lipid oxidation is initiated through the formation of alkyl free radicals by withdrawing hydrogen atoms from unsaturated fatty acids (initiation reaction). This radical reacts with unoxidized molecules to form other radicals, and oxidation proceeds rapidly (chain reaction). The radicals react with each other and the oxidation is terminated (termination reaction). In order to suppress oxidation, it is effective to add substances (antioxidants) to quench the radicals or inactivate radical-formation accelerators such as Fe2+. The action of antioxidants in O/W emulsions depends on its concentration, reactivity, partitioning between aqueous phase and the interface, interaction with other substances, the oil-phase component, pH and ionic strength of aqueous phase, and temperature.Citation48)
α-Tocopherol and ascorbyl palmitate are examples of commonly used antioxidants, and function by quenching the lipid radicals. The antioxidative abilities of these substances are higher than those of trolox and ascorbic acid which are analogs of α-tocopherol and ascorbyl palmitate, respectively.Citation48) The difference in antioxidative ability of various substances can be explained by the polar paradox.Citation48,49) Polar antioxidants exist in the aqueous phase, while those that are apolar exist in the oil phase to quench the radical directly. However, there are exceptions that cannot be explained by the paradox. Apart from the polarity of the antioxidant, factors such as the interaction of antioxidant with other substances should be noted.Citation50)
Chelating agents (e.g. EDTA) exhibit antioxidative activity by inactivating or chelating metal ions that initiate or promote lipid oxidation. Xanthan gum and α-carrageenan, which are used as thickeners in the preparation of O/W emulsions, can also chelate the metal ions.Citation51,52) Proteins used as emulsifiers are negatively charged at pH higher than their isoelectric point, and have the ability to suppress lipid oxidation by chelating the metal ions.Citation53) An excess amount of emulsifier or micelles existing in the aqueous phase can also interact with radicals or metal ions to suppress lipid oxidation.Citation54)
V. Autoxidation of microencapsulated oil
V.i. Factors affecting autoxidation of microencapsulated oil
Oil microencapsulation consists of two steps: the first is the homogenization of oil or lipid with a dense solution of wall material to produce the O/W emulsion, followed by the rapid dehydration of the resultant O/W emulsion to yield microcapsules. Encapsulation of oil can suppress or retard the oxidation,Citation55,56) the extent of which depends on the kind of wall material,Citation57,58) ratio of core material (oil) to wall material,Citation59,60) drying method and its conditions,Citation61,62) and storage conditions of microcapsules.Citation63) Since the exposed oil on the surface of the microcapsule is prone to oxidation, the surface oil content, which is defined as the ratio of the amount of oil exposed on the microcapsule surface to the whole oil in the microcapsule, would be related to the susceptibility of microencapsulated oil to autoxidation. The critical surface tension of oil to wall material is an indication in the selection of wall material to microencapsulate the oil droplet.Citation64) Smaller oil droplets in O/W emulsions exhibited higher stability against oxidation after microencapsulation.Citation59,65)
Fig. shows the autoxidation processes of linoleic acid (lipid) encapsulated with different weight ratios of gum arabic.Citation59) The microencapsulated lipids showed two distinct features in their oxidation: one is the rapid progress of oxidation in the early stage of storage, and another is the extremely slow oxidation or leveling-off in the oxidation at a certain fraction of unoxidized lipid (Y∞), in the late stage of storage. The effect of the ratio of lipid to wall material on the autoxidation of microencapsulated lipids was analyzed based on the model of percolation, and the two-dimensional model could satisfactorily explain the observed effects.Citation60) The lipid in the microcapsules could not be completely extracted with an organic solvent, and a certain fraction of the lipid remained in the microcapsules.Citation66) This fraction could be interpreted based on the model of percolation,Citation60) and correlated with the Y∞ value.Citation67)
V.ii. Models describing the oxidation process of microencapsulated oil
It is commonly thought that the retarded oxidation of microencapsulated oil is due to the diffusional barrier of the dehydrated layer of wall material. We discussed the validity of this idea based on a diffusion-reaction model where the lipid oxidation rate and the diffusion of oxygenCitation68) in microcapsules are formulated, and the model could not express the above-mentioned features in the oxidation of microencapsulated lipids.Citation69)
We supposed that lipid molecules interact with the wall material within the microcapsules to different extents, and that lipid molecules interacting more strongly are harder to oxidize. This difference in oxidation susceptibility was thought to be reflected in the free energy of activation, ΔG≠, while the distribution of ΔG≠ was assumed to be Gaussian, with a standard deviation of σ and the mean value, . The overall fraction of unoxidized lipid in a microcapsule at any time t,
, can be expressed by Equation (10).Citation69,70)
(10)
where R is the gas constant, is the rate constant corresponding to
, and Y0 is the initial value of Y. This model expressed the features well (solid curves in Fig. ).
The effects of oil fraction and oil droplet size within microcapsules on the surface oil content were examined by simulating the two- and three-dimensional models of percolation.Citation71) The simulation suggested that the surface oil content was lower when the oil content was lower in the microcapsules and that smaller droplets were more favorable for the production of microcapsules wherein the oil was hardly oxidized.
The surface-oil contents of microcapsules with differing oil-droplet-to-microcapsule size ratios were estimated based on a two-dimensional percolation model to examine the effects of differences in the ratio distribution. The surface-oil contents of microcapsules with various oil fraction volumes and different ratios were evaluated. The variance in the distribution of the oil-droplet-to-microcapsule size ratio had no significant effect on the surface-oil content at any oil fraction in the microcapsules.Citation72)
Disclosure statement
No potential conflict of interest was reported by the authors.
References
- McClements DJ. Food emulsions: principles, practices, and techniques. Boca Raton (FL): CRC Press; 2015.10.1201/b18868
- Guzey D, McClements DJ. Formation, stability and properties of multilayer emulsions for application in the food industry. Adv Colloid Interface Sci. 2006;128–130:227–248.10.1016/j.cis.2006.11.021
- Jiménez-Colmenero F. Potential applications of multiple emulsions in the development of healthy and functional foods. Food Res Int. 2013;52:64–74.10.1016/j.foodres.2013.02.040
- Floury J, Desrumaux A, Lardières J. Effect of high-pressure homogenization on droplet size distributions and rheological properties of model oil-in-water emulsions. Innov Food Sci Emerg Technol. 2000;1:127–134.10.1016/S1466-8564(00)00012-6
- Walstra P. Emulsion Stability. In: Schuster D, editor. Encyclopedia of emulsion technology. Boca Raton (FL): CRC Press; 1996. p. 1–62.
- Lambrich U, Schubert H. Emulsification using microporous systems. J Memb Sci. 2005;257:76–84.10.1016/j.memsci.2004.12.040
- Sugiura S, Nakajima M, Iwamoto S, et al. Interfacial Tension driven monodispersed droplet formation from microfabricated channel array. Langmuir. 2001;17:5562–5566.10.1021/la010342y
- Jafari SM, Assadpoor E, He Y, et al. Re-coalescence of emulsion droplets during high-energy emulsification. Food Hydrocoll. 2008;22:1191–1202.10.1016/j.foodhyd.2007.09.006
- Tadros T, Izquierdo P, Esquena J, et al. Formation and stability of nano-emulsions. Adv Colloid Interface Sci. 2004;108–109:303–318.10.1016/j.cis.2003.10.023
- Raikar NB, Bhatia SR, Malone MF, et al. Experimental studies and population balance equation models for breakage prediction of emulsion drop size distributions. Chem Eng Sci. 2009;64:2433–2447.10.1016/j.ces.2009.01.062
- Katsuki K, Miyagawa Y, Matsuno R, et al. Evolution of the size distribution of oil-droplets over time in oil-in-water emulsions. Jpn J Food Eng. 2015;16:231–234.
- Miyagawa Y, Shima M, Matsuno R, et al. Energy efficiency of different emulsification methods: a comparative evaluation. Jpn J Food Eng. 2015;16:71–74.
- Taylor P. Ostwald ripening in emulsions: estimation of solution thermodynamics of the disperse phase. Adv Colloid Interface Sci. 2003;106:261–285.10.1016/S0001-8686(03)00113-1
- Solans C, Izquierdo P, Nolla J, et al. Nano-emulsions. Curr Opin Colloid Interface Sci. 2005;10:102–110.10.1016/j.cocis.2005.06.004
- Sadurní N, Solans C, Azemar N, et al. Studies on the formation of O/W nano-emulsions, by-low-energy emulsification methods, suitable for pharmaceutical applications. Eur J Pharm Sci. 2005;26:438–445.10.1016/j.ejps.2005.08.001
- Dickinson E. Hydrocolloids at interfaces and the influence on the properties of dispersed systems. Food Hydrocoll. 2003;17:25–39.10.1016/S0268-005X(01)00120-5
- Damodaran S. Protein stabilization of emulsions and foams. J. Food Sci. 2005;70:54–66.
- Dickinson E. Proteins at interfaces and in emulsions stability, rheology and interactions. Phys Chem Chem Phys. 1998;94:1657–1669.
- Cao Y, Dickinson E, Wedlock DJ. Creaming and flocculation in emulsions containing polysaccharide. Food Hydrocoll. 1990;4:185–195.10.1016/S0268-005X(09)80151-3
- Dalgleish DG, Goff HD, Brun JM. Exchange reactions between whey proteins and caseins in heated soya oil-in-water emulsions systems—overall aspects of the reaction. Food Hydrocoll. 2002;16:303–311.10.1016/S0268-005X(01)00103-5
- Le Denmat M, Anton M, Beaumal V. Characterisation of emulsion properties and of interface composition in O/W emulsions prepared with hen egg yolk, plasma and granules. Food Hydrocoll. 2000;14:539–549.10.1016/S0268-005X(00)00034-5
- Cornec M, Wilde PJ, Gunning PA, et al. Emulsion stability as affected by competitive adsorption between an oil-soluble emulsifier and milk proteins at the interface. J Food Sci. 1998;63:39–43.10.1111/jfds.1998.63.issue-1
- Nakamura A, Maeda H, Corredig M. Competitive adsorption of soy soluble polysaccharides in oil-in-water emulsions. Food Res Int. 2004;37:823–831.10.1016/j.foodres.2004.04.005
- Berton-Carabin CC, Ropers MH, Genot C. Lipid oxidation in oil-in-water emulsions: involvement of the interfacial layer. Compr Rev Food Sci Food Saf. 2014;13:945–977.10.1111/crf3.2014.13.issue-5
- Chuah AM, Kuroiwa T, Kobayashi I, et al. Effect of chitosan on the stability and properties of modified lecithin stabilized oil-in-water monodisperse emulsion prepared by microchannel emulsification. Food Hydrocoll. 2009;23:600–610.10.1016/j.foodhyd.2008.03.014
- Ru Q, Yu H, Huang Q. Encapsulation of epigallocatechin-3-gallate (EGCG) using oil-in-water (O/W) submicrometer emulsions stabilized by ι-carrageenan and β-lactoglobulin. J Agric Food Chem. 2010;58:10373–10381.10.1021/jf101798m
- Verwey EJW, Overbeek JTG. Theory of the stability of liophobic colloid. North Chelmsford (MA): Courier Corporation; 1999.
- Fuchs N., Über die Stabilität und Aufladung der Aerosole [About the stability and charging of aerosols]. Z Physik. 1934;89:736–743.10.1007/BF01341386
- Adachi S, Imagi J, Matsuno R. Model for estimation of the stability of emulsions in a cream layer. Biosci Biotechnol Biochem. 1992;56:495–498.10.1271/bbb.56.495
- Adachi S, Nagao K, Asawathamakittee S, et al. Effect of polymer additives on the stability of O/W emulsions. Food Sci Technol Res. 1996;2:203–208.
- Ortega-Vinuesa JL, Martı́n-Rodrı́guez A, Hidalgo-Álvarez R. Colloidal stability of polymer colloids with different interfacial properties: mechanisms. J Colloid Interface Sci. 1996;184:259–267.10.1006/jcis.1996.0619
- Spielman LA. Viscous interactions in Brownian coagulation. J Colloid Interface Sci. 1970;33:562–571.10.1016/0021-9797(70)90008-1
- Grasso D, Subramaniam K, Butkus M, et al. A review of non-DLVO interactions in environmental colloidal systems. Rev Environ Sci Biotechnol. 2002;1:17–38.10.1023/A:1015146710500
- Reerink H, Overbeek JTG. The rate of coagulation as a measure of the stability of silver iodide sols. Discuss Faraday Soc. 1954;18:74–84.10.1039/df9541800074
- Derjaguin BV. Summarizing remarks, colloid stability. Discuss Faraday Soc. 1966;42:317–321.10.1039/df9664200317
- Miyagawa Y, Katsuki K, Matsuno R, et al. Effect of oil droplet size on activation energy for coalescence of oil droplets in an O/W emulsion. Biosci Biotechnol Biochem. 2015;79:1695–1697.10.1080/09168451.2015.1039482
- Lethuaut L, Métro F, Genot C. Effect of droplet size on lipid oxidation rates of oil-in-water emulsions stabilized by protein. J Am Oil Chem Soc. 2002;79:425–430.10.1007/s11746-002-0500-z
- Cercaci L, Rodriguez-Estrada MT, Lercker G, et al. Phytosterol oxidation in oil-in-water emulsions and bulk oil. Food Chem. 2007;102:161–167.10.1016/j.foodchem.2006.05.010
- Osborn HT, Akoh CC. Effect of emulsifier type, droplet size, and oil concentration on lipid oxidation in structured lipid-based oil-in-water emulsions. Food Chem. 2004;84:451–456.10.1016/S0308-8146(03)00270-X
- Nakaya K, Ushio H, Matsukawa S, et al. Effects of droplet size on the oxidative stability of oil-in-water emulsions. Lipids. 2005;40:501–507.10.1007/s11745-005-1410-4
- Belhaj N, Arab-Tehrany E, Linder M. Oxidative kinetics of salmon oil in bulk and in nanoemulsion stabilized by marine lecithin. Process Biochem. 2010;45:187–195.10.1016/j.procbio.2009.09.005
- Mao LK, Xu DX, Yang J, et al. Effects of small and large molecule emulsifiers on the characteristics of beta-carotene nanoemulsions prepared by high pressure homogenization. Food Technol Biotechnol. 2009;47:336–342.
- Özilgen S, Özilgen M. Kinetic model of lipid oxidation in foods. J Food Sci. 1990;55:498–498.10.1111/jfds.1990.55.issue-2
- Adachi S, Ishiguro T, Matsuno R. Autoxidation kinetics for fatty acids and their esters. J Am Oil Chem Soc. 1995;72:547–551.10.1007/BF02638855
- Kikuchi K, Yamamoto S, Shiga H, et al. Effect of reducing oil droplet size on lipid oxidation in an oil-in-water emulsion. Jpn J Food Eng. 2014;15:43–47.
- Miyagawa Y, Kikuchi K, Shiga H, et al. Effect of polydispersity in oil-droplet size on lipid oxidation in oil-in-water emulsions. Jpn J Food Eng. 2016;3:91–94.
- Imai H, Maeda T, Shima M, et al. Oxidation of methyl linoleate in oil-in-water micro- and nanoemulsion systems. J Am Oil Chem Soc. 2008;85:809–815.10.1007/s11746-008-1257-3
- Frankel EN. Lipid oxidation. Dundee: The Oil Press; 1998.
- Porter WL, Black ED, Drolet AM. Use of polyamide oxidative fluorescence test on lipid emulsions: contrast in relative effectiveness of antioxidants in bulk versus dispersed systems. J Agric Food Chem. 1989;37:615–624.10.1021/jf00087a009
- Waraho T, McClements DJ, Decker EA. Mechanisms of lipid oxidation in food dispersions. Trends Food Sci Technol. 2011;22:3–13.10.1016/j.tifs.2010.11.003
- Shimada K, Fujikawa K, Yahara K, et al. Antioxidative properties of xanthan on the autoxidation of soybean oil in cyclodextrin emulsion. J Agric Food Chem. 1992;40:948–954.
- Chen B, McClements DJ, Decker EA. Role of continuous phase anionic polysaccharides on the oxidative stability of menhaden oil-in-water emulsions. J Agric Food Chem. 2010;58:3779–3784.10.1021/jf9037166
- Faraji H, McClements DJ, Decker EA. Role of continuous phase protein on the oxidative stability of fish oil-in-water emulsions. J Agric Food Chem. 2004;52:4558–4564.10.1021/jf035346i
- Berton-Carabin CC, Ropers MH, Genot C. Lipid oxidation in oil-in-water emulsions: Involvement of interfacial layer. Compr Rev Food Sci Food Saf. 2014;13:94–977.
- Imagi J, Muraya K, Yamashita D, et al. Retarded oxidation of liquid lipids entrapped in matrixes of saccharides or proteins. Biosci Biotechnol Biochem. 1992;56:1236–1240.10.1271/bbb.56.1236
- Kikuchi S, Fang X, Shima M, et al. Oxidation of arachidonoyl glycerols encapsulated with saccharides. Food Sci Technol Res. 2006;12:247–251.10.3136/fstr.12.247
- Minemoto Y, Fang X, Hakamata K, et al. Oxidation of linoleic acid encapsulated with soluble soybean polysaccharide by spray-drying. Biosci Biotechnol Biochem. 2002;66:1829–1834.10.1271/bbb.66.1829
- Fang X, Watanabe Y, Adachi S, et al. Microencapsulation of linoleic acid with low- and high-molecular-weight components of soluble soybean polysaccharide and its oxidation process. Biosci Biotechnol Biochem. 2003;67:1864–1869.10.1271/bbb.67.1864
- Minemoto Y, Hakamata K, Adachi S, et al. Oxidation of linoleic acid encapsulated with gum arabic or maltodextrin by spray-drying. J Microencapsulation. 2002;19:181–189.10.1080/02652040110065468
- Minemoto Y, Adachi S, Matsuno R. Autoxidation of Linoleic acid encapsulated with polysaccharides of differing weight ratio. Biosci Biotechnol Biochem. 1999;63:866–869.10.1271/bbb.63.866
- Minemoto Y, Adachi S, Matsuno R. Comparison of oxidation of methyl linoleate encapsulated with gum arabic by hot-air-drying and freeze-drying. J Agric Food Chem. 1997;45:4530–4534.10.1021/jf970465h
- Fang X, Shima M, Adachi S. Effects of drying conditions on the oxidation of linoleic acid encapsulated with gum arabic by spray-drying. Food Sci Technol Res. 2005;11:380–384.10.3136/fstr.11.380
- Minemoto Y, Adachi S, Matsuno R. Effect of relative humidity during storage on the autoxidation of linoleic acid encapsulated with a polysaccharide by hot-air-drying and freeze-drying. Food Sci Technol Res. 2001;7:91–93.10.3136/fstr.7.91
- Adachi S, Iwase H, Matsuno R. Relationship between the amount of lipid exposed at the surface of the lipid emulsions encapsulated by a polymer and the critical surface tension of the dehydrated polymer film. Nippon Shokuhin Kagaku Kogaku Kaishi. 1995;42:137–139.10.3136/nskkk.42.137
- Nakazawa R, Shima M, Adachi S. Effect of oil-droplet size on the oxidation of microencapsulated methyl linoleate. J Oleo Sci. 2008;57:225–232.10.5650/jos.57.225
- Minemoto Y, Adachi S, Matsuno R. Solvent-extraction processes of linoleic acid encapsulated with gum arabic, pullulan or maltodextrin at different weight ratios. Food Sci Technol Res. 1999;5:289–293.10.3136/fstr.5.289
- Minemoto Y, Adachi S, Matsuno R. Autoxidation and solvent-extraction processes of linoleic acid encapsulated with a polysaccharide by freeze-drying. Food Sci Technol Res. 2000;6:221–224.10.3136/fstr.6.221
- Adachi S, Muraya K, Ishiguro T, et al. Apparent diffusion coefficient of oxygen through dehydrated protein or saccharide films. J Pack Sci Technol. 1994;3:93–101.
- Ishido E, Minemoto Y, Adachi S, et al. Heterogeneity during autoxidation of linoleic acid encapsulated with a polysaccharide. J Food Eng. 2003;59:237–243.10.1016/S0260-8774(02)00463-6
- Ishido E, Hakamata K, Minemoto Y, et al. Oxidation process of linoleic acid encapsulated with a polysaccharide by spray-drying. Food Sci Technol Res. 2001;8:85–88.
- Kikuchi K, Yamamoto S, Shiga H, et al. Surface oil content of microcapsules containing various oil fractions and oil-droplet sizes. Jpn J Food Eng. 2013;14:169–173.
- Kikuchi K, Yamamoto S, Shiga H, et al. Effects of the oil-droplet size and entire oil fraction in microcapsules on the interior oil fraction. Jpn J Food Eng. 2015;16:303–305.