Abstract
Ferulic acid (FA) is a phenol compound found in plants that has anti-inflammatory properties. Indoleamine 2, 3-dioxygenase (IDO) is a tryptophan catabolic enzyme induced in immune cells, including glial cells, during inflammation. Enhanced IDO expression leads to reduced tryptophan levels and increased levels of toxic metabolites, including quinolinic acid. Therefore, inhibition of IDO expression may be effective in suppressing progression of neurodegenerative diseases. In this study, we examined the effect of FA in microglial cells on IDO expression levels and related inflammatory signal molecules. FA suppressed LPS-induced IDO mRNA expression and also suppressed nuclear translocation of NF-κB and phosphorylation of p38 MAPK. However, FA did not affect the production of LPS-induced inflammatory mediators and phosphorylation of JNK. Our results indicate that FA suppresses LPS-induced IDO mRNA expression, which may be mediated by inhibition of the NF-κB and p38 MAPK pathways in microglial cells.
Graphical abstract
Ferulic acid suppresses lipopolysaccharide-induced mRNA expression of indoleamine 2,3-deoxygenase via inhibition of NFκB and p38 MAPK pathways in microglial cells.
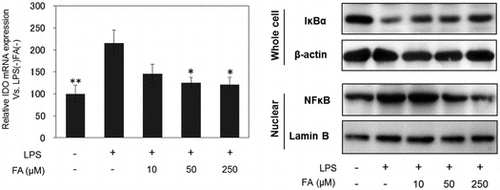
Ferulic acid (FA) is a phenol compound present in the cell wall of plants and also found in fibrous foods of plant origin, such as rice and wheat bran.Citation1) In addition to consuming unrefined foods, supplementation of FA has become possible in recent years. FA has antioxidativeCitation2–4) and anti-inflammatoryCitation5) properties and is reported to exert suppressive effects against obesity,Citation6) hypertension,Citation7,8) and hyperlipidemia.Citation9)
Microglial cells are resident immune cells that play a role in monitoring and maintaining neuronal cells in the central nervous system (CNS).Citation10,11) Conversely, once the inflammatory cytokines, tumor necrosis factor-alpha (TNF-α) and interleukin-6 (IL-6), and nitric oxide (NO) are secreted from microglial cells, they accelerate CNS inflammation.Citation12,13) Signaling involving nuclear factor-kappa B (NF-κB)Citation14,15) or mitogen-activated protein kinases, including extracellular signal-regulated kinase (Erk),Citation16) c-jun N-terminal kinase (JNK),Citation17) and p38 MAPK,Citation16,18) plays an important role in inducing microglial inflammation. Microglial cells are reported to accumulate around affected brain regions in Alzheimer’s, Parkinson’s, and Huntington’s diseases, suggesting they may be involved in inflammation of neurodegenerative diseases.Citation19,20)
Previously, quinolinic acid (QUIN), a tryptophan metabolite, was observed to increase in brains of patients with neurodegenerative diseases.Citation21) QUIN is a ligand of N-methyl-D-aspartate (NMDA) receptors, which are involved in the induction of neuroexcitotoxicity.Citation22) Indoleamine 2, 3-dioxygenase (IDO) is a rate limiting enzyme in the CNS tryptophan-metabolizing pathway, present upstream of this pathway producing QUIN. Moreover, IDO expression is induced in microglial cells during various inflammatory situations,Citation21,23–26) leading to increased QUIN levels and suggesting that inhibition of IDO expression may be effective to suppress neurodegenerative diseases.
FA can pass through the blood–brain barrier that is critical for controlling permeability of materials from the blood to brain.Citation27) Indeed, transvenous FA administration suppresses neuronal cell apoptosis in rats with cerebral ischemic injury.Citation28) Additionally, in vivo studies indicate that FA, or its ethyl ester body, have suppressive effects against amyloid β-induced neurotoxicity via anti-inflammatory properties.Citation29,30) However, little is known about the relationship between microglial IDO expression and the anti-inflammatory effect of FA in the CNS.
Therefore, in the present study, we investigated the effect of FA on IDO expression in microglial cells involved in neurodegenerative disease pathogenesis.
Material and methods
Materials
Lipopolysaccharide (LPS) (E. coli O-111) was purchased from Sigma-Aldrich (St Louis, MO, USA), and FA from Tokyo Chemical Industry (Tokyo, Japan). LPS and FA were dissolved in phosphate-buffered saline (PBS) and dimethylsulfoxide, respectively.
Cell culture
The MG6 cell line, derived from mouse microglial cells,Citation31,32) was obtained from the RIKEN Cell Bank. MG6 cells were cultured in 100 mm dishes in Dulbecco’s modified Eagle’s medium (Nissui Pharmaceutical, Tokyo, Japan) with 10% fetal bovine serum (Biological Industries, Kibbutz Beit Haemek, Israel). Cells were seeded on 12-well plates (0.5 × 106 cells/well) or 60 mm dishes (4.0 × 106 cells/dish) for total RNA or protein extraction, respectively. MG6 cells were treated with LPS (300 ng/mL) and/or FA (10, 50, 250 μM) for 24 h for the detection of TNF-α, IL-6, and NO secretion and mRNA expression levels.
Protein collection was performed after 5 (for p38), 15 (for JNK and Erk), or 30 (for IκBα and NF-κB) min, when activation of these proteins by LPS were clearly observed, according to the results of preliminary experiments (data not shown).
Cell viability assay
Cell viability was determined using CellTiter 96® AQueous One Solution Cell Proliferation Assay (Promega), according to the manufacturer’s protocol. Briefly, cells were seeded on 96-well plates and treated with or without FA (10, 50, 250 μM). After 24 h FA treatment, 20 μL of Cell Titer regent was added to each well, incubated for 15 min at 37 °C, and the absorbance at 450 nm measured.
TNF-α and IL-6 detection
After incubating MG6 cells for 24 h, TNF-α and IL-6 concentrations in the cell medium were measured using ELISA MAX™ (BioLegend, San Diego, CA, USA), according to the manufacturer’s protocol.
Nitric oxide (NO) assay
After incubating MG6 cells for 24 h, NO concentration in the medium was measured by the Griess reaction method.Citation33) Briefly, 100 μL per well of culture medium was applied to 96-well plates, and 100 μL of an equal volume mixture of Griess reagent (0.2% N-1-naphthyl ethylenediamine (Wako pure chemical Co., Osaka, Japan) and 2% sulfanilamide (Sigma-Aldrich) in 10% H3PO4 (Wako)) added to each well. After incubating for 10 min at room temperature, absorbance at 550 nm was measured using an Infinite® F200 Pro (Tecan, Männedorf, Switzerland).
Total RNA extraction and cDNA synthesis
After cell treatments, 500 μL per well of RNAiso plus regent (Takara, Shiga, Japan) was added to cells seeded on 12-well plates. Total RNA was extracted from cells according to the manufacturer’s protocol. Extracted total RNA was reverse-transcribed to cDNA using the PrimeScript® RT Reagent Kit (Takara), according to the manufacturer’s protocol.
Quantitative PCR analysis
Reverse-transcribed cDNA was amplified using SYBR® Premix Ex Taq™ (Takara) and specific primers of the following sequences: IDO 5′-primer (forward), 5′-GGGATGACGATGTTCGAAAG-3′; and 3′-primer (reverse), 5′-GTGGACCCAGACACGTTTTT-3′; 36B4 5′-primer (forward), 5′-TGTGTGTCTGCAGATCGGGTAC-3′; and 3′-primer (reverse), 5′-CTTTGGCGGGATTAGTCGAAG-3′. Reactions and fluorescence detection were performed using ABI PRISM 7000 Sequence Detection System (Life Technologies, Carlsbad, CA, USA). 36B4 mRNA expression levels were used as the internal standard for determination of IDO mRNA expression levels.
Protein extraction
After treatment, cells were washed with PBS and collected in protein extraction buffer (20 mM Tris-HCl, 150 mM NaCl, and 1% Triton) containing protease inhibiter (Calbiochem, La Jolla, CA, USA) and phosphatase inhibitor (Wako) cocktails. Solutes were fractionated using an ultrasonic disintegrator, and lysates centrifuged at 13,000 × g, 4 °C for 5 min. Centrifuged supernatants were collected as total protein solutions. Cell nuclear proteins were extracted by the following method. First, cells were collected in PBS and centrifuged at 1200 × g, 4 °C for 5 min. Collection buffer (25 mM Tris-HCl, 250 mM sucrose, and 1 mM EDTA) containing protease inhibitor cocktail was added to the precipitate. Cell membranes were fractured using a glass-Teflon homogenizer, and homogenates centrifuged at 3000 × g, 4 °C for 5 min. Precipitates were dissolved in RIPA buffer (150 mM NaCl, 50 mM Tris-HCl, 1% Triton, 0.5% sodium deoxycholate, and 0.1% sodium dodecyl sulfate), and the resulting solution was collected as nucleus protein solution. Total protein solution was used to detect IκBα, p38, Erk, and JNK protein expressions, and nucleus protein solution for NF-κB protein expression.
Western blot analysis
Protein solutions were separated by 10% sodium dodecyl sulfate polyacrylamide gel electrophoresis and transferred to polyvinylidene difluoride membranes (Millipore, Billerica, MA, USA). Membranes were incubated in 5% skimmed milk or nonfat dried milk solution for 1 h and then washed three times in PBS or TBS containing 0.05% Tween 20 (PBST and TBST). Next, membranes were treated overnight with the following antibodies: IκBα (1:200) (sc-371), Lamin B (1:1000) (sc-6217) (Santa Cruz Biotechnology, Santa Cruz, CA, USA), NF-κB (1:1000) (#4764), p-p38 (1:100) (#9215), p38 (1:1000) (#9212S), p-Erk (1:750) (#9101S), Erk (1:1000) (#4695), p-JNK (1:300) (#9251), JNK (1:1000) (#9252), or β-actin (1:1000) (#4967) (all from Cell Signaling Technology, Beverly, MA, USA). After washing unreacted primary antibodies with PBST or TBST, membranes were treated with secondary antibody, specifically, anti-rabbit IgG horseradish peroxidase (HRP) conjugate (1:1000–5000) (W4018) (Promega, Madison, WI, USA). Membranes were treated with Immobilon Western Chemiluminescent HRP Substrate (Millipore), and fluorescence detected by Fuji LAS-1000 (Fujifilm, Tokyo, Japan). Luminescence intensity was quantified using Image J software (National Institutes of Health, Bethesda, MD, USA).
Statistical analysis
All values were shown as mean ± standard error (SE). Results were analyzed by Tukey’s test after one-way ANOVA or Shirley–Williams test for the detection of significant differences using Excel-Toukei ver. 6.0 (ESUMI, Co., Ltd., Tokyo, Japan). A value of *p < 0.05 was considered statistically significant.
Results
Inflammatory marker production
First, we measured the cytotoxicity of FA. 250 μM FA without LPS treatment suppressed cell viability (supplemental Fig. 1). However, >90% survival rate were observed in cells treated with 10–250 μM of FA in the presence of LPS (Supplemental Fig. 2). FA did not affect cell viability in the presence of LPS.
Production of proinflammatory cytokines, such as TNF-α and IL-6, precede IDO expression during CNS inflammation.Citation34,35) Therefore, we measured levels of these cytokines as markers of inflammation. In addition, we measured NO, which is also used as a marker of inflammation and considered a putative suppressor of IDO expression.Citation36)
TNF-α (Fig. (A)) and IL-6 (Fig. (B)) productions were increased in LPS-stimulated MG6 cells, and FA did not affect these production levels. NO production was also induced by LPS treatment and was not affected by FA (Fig. (C)).
IDO mRNA expression
Our preliminary experiments indicated that IDO mRNA expression maximally increases after 24 h of LPS treatment (data not shown). Thus, we examined the effect of FA on IDO mRNA expression in microglial cells treated with LPS for 24 h. FA suppressed LPS-induced IDO mRNA expression in a dose-dependent manner (Fig. ).
Expression of NF-κB and MAPK pathway proteins
NF-κB and MAPK are crucial pathways for inducing expression of inflammatory mediators.Citation37,38) We found LPS-induced degradation of NF-κB inhibitor alpha (IκBα), nuclear localization of NF-κB (Fig. (A)), phosphorylation of p38 MAPK (Fig. (B)) were suppressed by FA in a dose-dependent manner. Erk Phosphorylation was slightly attenuated by 250 μM of FA treatment (Fig. (B)). LPS-induced phosphorylation of JNK (Fig. (B)) was not affected by FA.
Discussion
In response to inflammatory stimuli, microglial cells produce many inflammatory substances including TNF-α, IL-6, and NO.Citation12,13,39–41) In addition to the direct effect of TNF-α, IL-6, NO, and other inflammatory elements, enhanced IDO expression in microglial cells or astrocytes is an important mechanistic factor in CNS inflammation.Citation40–42) Inflammatory cytokines produced in glial cells in the early period of inflammation promote further inflammation through cross talk between glial and neuronal cells.Citation36,37) Induced IDO expression in activated microglial cells and astrocytes,Citation43) enhances tryptophan degradation and QUIN production and causes neuronal excitotoxicity.Citation44) Increased QUIN induces neuronal death by NMDA glutamate receptor activation, leading to CNS failure.Citation44,45) Moreover, amyloid β, which accumulates in Alzheimer’s disease lesions, induces IDO expression and QUIN production, suggesting IDO involvement in neurodegenerative diseases.Citation21) Thus, regulation of IDO expression through diet may be useful for the prevention of neurodegenerative diseases. Yamamoto et al. reported that some kind of phytochemicals suppress IDO expression.Citation46) However, there is no information whether FA can suppress IDO expression. In this study, we investigated the effect of FA on IDO expression in microglial cells.
We treated microglial cells with LPS to mimic the inflammatory state in neurodegenerative patients. LPS is an endotoxin derived from Gram-negative bacteria and used experimentally to induce inflammation.Citation47)
In the present study, we found LPS-induced IDO mRNA expression was significantly suppressed by FA treatment in microglial cells.
Fujigaki et al. reported that NF-κB and p38 MAPK are involved in gene expression of IDO, and the predicted NF-κB response element is located in the 5’ upstream region of the IDO gene.Citation48) JNK is also involved in the regulation of IDO expression.Citation49) Therefore, to examine the suppressive mechanism of IDO expression by FA, we investigated expression of NF-κB and MAPK-signaling pathway proteins.
In the unstimulated state, IκBα binds to transcriptional factor NF-κB and inhibits nuclear localization of it. However, phosphorylation of IκBα causes its degradation and allows NF-κB to translocate to the nucleus.Citation50) Erk, JNK, and p38 are MAPK family members that are phosphorylated and undergo nuclear translocation in response to inflammatory stimuli.Citation51)
In this study, LPS-induced degradation of IκBα in cytoplasm and nuclear translocation of NF-κB were both suppressed by FA treatment in a dose-dependent manner. Furthermore, LPS-induced phosphorylation of p38 was attenuated by FA treatment. The effect of FA on LPS-induced Erk phosphorylation was not remarkable, and FA did not affect JNK phosphorylation. These results suggest that inhibition of p38 phosphorylation and nuclear translocation of NF-κB would be involved in the inhibitory mechanism of IDO expression by FA.
In this experiment, production of the inflammatory factors TNF-α, IL-6, and NO, were not affected by FA treatment under this experimental condition, which may be caused by maintained phosphorylation level of JNK and Erk.
FA affects IDO expression in microglial cells; hence, it is feasible that FA may have a suppressive effect on CNS inflammation in vivo by the same mechanism. IDO is closely involved in neurodegenerative disease pathogenesis.Citation52,53) Thus, suppression of IDO expression by oral intake of FA supplements or food containing large amounts of FA including unrefined grains may be helpful for avoiding CNS inflammation.
Author contributions
Y.E. designed the study. M.K. and H.K. conducted the experiments. M.K., S.H. and Y.E. drafted the manuscript. All authors read and approved the final manuscript.
Disclosure statement
No potential conflict of interest was reported by the authors.
Funding
This work was supported by grants-in-aid to Y.E. from the Ministry of Education, Culture, Sports, Science and Technology of Japan.
Supplemental material
Supplemental material for this article can be accessed at http://dx.doi.org/10.1080/09168451.2016.1274636.
TBBB_1274636_Supplemental_Data.pdf
Download PDF (275.3 KB)References
- Zhao Z, Moghadasian MH. Chemistry, natural sources, dietary intake and pharmacokinetic properties of ferulic acid: a review. Food Chem. 2008;109:691–702.10.1016/j.foodchem.2008.02.039
- Kikuzaki H, Hisamono M, Hirose K, et al. Antioxidant properties of ferulic acid and its related compounds. J Agric Food Chem. 2002;50:2161–2168.10.1021/jf011348w
- Scott BC, Butler J, Halliwell B, et al. Evaluation of the antioxidant actions of ferulic acid and catechins. Free Rad Res Commun. 1993;19:241–253.10.3109/10715769309056512
- Kanski J, Aksenova M, Stoyanova A, et al. Ferulic acid antioxidant protection against hydroxyl and peroxyl radical oxidation in synaptosomal and neuronal cell culture systems in vitro: structure-activity studies. J Nutr Biochem. 2002;13:273–281.10.1016/S0955-2863(01)00215-7
- Sudheer AR, Muthukumaran S, Devipriya N, et al. Influence of ferulic acid on nicotine-induced lipid peroxidation, DNA damage and inflammation in experimental rats as compared to N-acetylcysteine. Toxicol. 2008;243:317–329.
- Son MJ, Rico CW, Nam SH, et al. Influence of oryzanol and ferulic acid on the lipid metabolism and antioxidative status in high fat-fed mice. J Clin Biochem Nutr. 2010;46:150–156.10.3164/jcbn.09-98
- Suzuki A, Kagawa D, Fujii A, et al. Short- and long-term effects of ferulic acid on blood pressure in spontaneously hypertensive rats. Am J Hypertens. 2002;15:351–357.10.1016/S0895-7061(01)02337-8
- Ardiansyah Ohsaki Y, Shirakawa H, Koseki T, et al. Novel effects of a single administration of ferulic acid on the regulation of blood pressure and the hepatic lipid metabolic profile in stroke-prone spontaneously hypertensive rats. J Agric Food Chem.. 2008;56:2825–2830.
- Jung EH, Kim SR, Hwang IK, et al. Hypoglycemic effects of a phenolic acid fraction of rice bran and ferulic acid in C57BL/KsJ-db/db mice. J Agric Food Chem. 2007;55:9800–9804.10.1021/jf0714463
- Streit WJ. Microglia as neuroprotective, immunocompetent cells of the CNS. Glia. 2002;40:133–139.
- Hanisch UK, Kettenmann H. Microglia: active sensor and versatile effector cells in the normal and pathologic brain. Nat Neurosci. 2007;10:1387–1394.10.1038/nn1997
- Liu B, Hong JS. Role of microglia in inflammation-mediated neurodegenerative diseases: mechanisms and strategies for therapeutic intervention. J Pharmacol Exp Ther. 2003;304:1–7.10.1124/jpet.102.035048
- Minagar A, Shapshak P, Fujimura R, et al. The role of macrophage/microglia and astrocytes in the pathogenesis of three neurologic disorders: HIV-associated dementia, Alzheimer disease, and multiple sclerosis. J Neurolog Sci. 2002;202:13–23.10.1016/S0022-510X(02)00207-1
- Mattson MP, Camandola S. NF-κB in neuronal plasticity and neurodegenerative disorders. J Clin Invest. 2001;107:247–254.10.1172/JCI11916
- Camandola S, Mattson MP. NF-κB as a therapeutic target in neurodegenerative diseases. Expert Opin Ther Targets. 2007;11:123–132.10.1517/14728222.11.2.123
- Koistinaho M, Koistinaho J. Role of p38 and p44/42 mitogen-activated protein kinases in microglia. Glia. 2002;40:175–183.10.1002/(ISSN)1098-1136
- Hidding U, Mielke K, Waetzig V, et al. The c-Jun N-terminal kinase in cerebral microglia: immunological functions in the brain. Bochem Pharmacol. 2002;64:781–788.10.1016/S0006-2952(02)01139-5
- Lee YB, Schrader JW, Kim SU. p38 MAP kinase regulates TNF-production in human astrocytes and microglia by multiple mechanisms. Cytokine. 2000;12:874–880.10.1006/cyto.2000.0688
- Block ML, Hong JS. Microglia and inflammation-mediated neurodegeneration: multiple triggers with a common mechanism. Prog Neurobiol. 2005;76:77–98.10.1016/j.pneurobio.2005.06.004
- D’Andrea MR, Cole GM, Ard MD. The microglial phagocytic role with specific plaque types in the Alzheimer disease brain. Neurobiol Aging. 2004;25:675–683.10.1016/j.neurobiolaging.2003.12.026
- Guillemin GJ, Brew BJ, Noonan CE, et al. Indoleamine 2,3-dioxygenase and quinolinic acid Immunoreactivity in Alzheimer’s disease hippocampus. Neuropathol Appl Neurobiol. 2005;31:395–404.10.1111/nan.2005.31.issue-4
- Ganong AH, Cotman CW. Kynurenic acid and quinolinic acid act at N-methyl-D-aspartate receptors in the rat hippocampus. J Pharmacol Exp Ther. 1985;236:293–299.
- Sardar AM, Reynolds GP. Frontal cortex indoleamine-2,3-dioxygenase activity is increased in HIV-l-associated dementi. Neurosci Lett. 1995;187:9–12.10.1016/0304-3940(95)11324-P
- Chen Y, Stankovic R, Cullen KM, et al. The kynurenine pathway and inflammation in amyotrophic lateral sclerosis. Neurotox Res. 2010;18:132–142.10.1007/s12640-009-9129-7
- Hoshi M, Saito K, Murakami Y, et al. Marked increases in hippocampal neuron indoleamine 2, 3-dioxygenase via IFN-g-independent pathway following transient global ischemia in mouse. Neurosci Res. 2009;63:194–198.10.1016/j.neures.2008.12.003
- O’Connor JC, Lawson MA, Andre′ C, et al. Lipopolysaccharide-induced depressive-like behavior is mediated by indoleamine 2,3-dioxygenase activation in mice. Mol Psychiatr. 2009;14:511–522.10.1038/sj.mp.4002148
- Qin J, Chen DW, Hu HY, et al. Body distribution of RGD-mediated liposome in brain-targeting drug delivary. Pharm Soc Jpn. 2007;127:1497–1501.
- Cheng CY, Sua SY, Tang NY, et al. Ferulic acid provides neuroprotection against oxidative stress-related apoptosis after cerebral ischemia/reperfusion injury by inhibiting ICAM-1 mRNA expression in rats. Brainres. 2008;1209:136–150.
- Yan JJ, Cho JY, Kim HS, et al. Protection against b-amyloid peptide toxicity in vivo with long-term administration of ferulic acid. Br J Pharmacol. 2001;133:89–96.10.1038/sj.bjp.0704047
- Perluigi M, Joshi G, Sultana R, et al. In vivo protective effects of ferulic acid ethyl ester against amyloid-beta peptide 1–42-induced oxidative stress. J Neurosci Res. 2006;84:418–426.10.1002/(ISSN)1097-4547
- Nakamichi K, Saiki M, Kitani H, et al. Suppressive effect of simvastatin on interferon–induced expression of CC chemokine ligand 5 in microglia. Neurosci Lett. 2006;407:205–210.10.1016/j.neulet.2006.08.044
- Takenouchi T, Ogihara K, Sato M, et al. Inhibitory effects of U73122 and U73343 on Ca2+ influx and pore formation induced by the activation of P2X7 nucleotide receptors in mouse microglial cell line. Biochim Biophys Acta. 2005;1726:177–186.10.1016/j.bbagen.2005.08.001
- Guevara I, Iwanejko J, Dembińska-Kieć A, et al. Determination of nitrite / nitrate in human biological material by the simple Griess reaction. Clin Chim Acta. 1998;274:177–188.10.1016/S0009-8981(98)00060-6
- Merrill JE, Benveniste EN. Cytokines in inflammatory brain lesions: helpful and harmful. Trends Neurosci. 1996;19:331–338.10.1016/0166-2236(96)10047-3
- McGeer PL, McGeer EG. The inflammatory response system of brain: implications for therapy of Alzheimer and other neurodegenerative diseases. Brain Res Rev. 1995;21:195–21834.10.1016/0165-0173(95)00011-9
- Thomas SR, Mohr D, Stocker R. Nitric oxide inhibits indoleamine 2,3-dioxygenase activitiyn interferon-γ primed mononuclear phagocyte. J Biol Chem. 1994;269:14457–14464.
- May MJ, Ghosh S. Signal transduction through NF-kB. Immunol Today. 1998;19:80–88.10.1016/S0167-5699(97)01197-3
- Kyriakis JM, Avruch J. Mammalian mitogen-activated protein kinase signal transduction pathways activated by stress and inflammation. Physiol Rev. 2001;81:807–869.
- Hanisch UK. Microglia as a source and target of cytokines. Glia. 2002;40:140–155.10.1002/(ISSN)1098-1136
- Zhang JM, An J. Cytokines, inflammation and pain. Int Anesthesiol Clin. 2007;45:27–37.10.1097/AIA.0b013e318034194e
- Nussler AK, Billiar TA. Inflammation, immunoregulation, and inducible nitric oxide synthase. J Leukoc Biol. 1993;54:171–178.
- Kwidzinski E, Bechmann I. IDO expression in the brain: a double-edged sword. J Mol Med. 2007;85:1351–1359.10.1007/s00109-007-0229-7
- Guillemin GJ, Smythe G, Takikawa O, et al. Expression of indoleamine 2,3-dioxygenase and production of quinolinic acid by human microglia, astrocytes, and neurons. Glia. 2004;49:15–23.
- Guillemin GJ. Quinolinic acid, the inescapable neurotoxin. FEBS J. 2012;279:1356–1365.10.1111/j.1742-4658.2012.08485.x
- Orlando LR, Alsdorf SA, Penney JB Jr, et al. The role of group I and group II metabotropic glutamate receptors in modulation of striatal NMDA and quinolinic acid toxicity. Exp Neurol. 2001;167:196–204.10.1006/exnr.2000.7542
- Yamamoto R, Yamamoto Y,Imai S, et al. Effects of various phytochemicals on indoleamine 2,3-dioxygenase 1 activity: galanal is a novel, competitive inhibitor of the enzyme., PLoS One. 2014;9:e88789.
- Raetz CRH, Whitfield C. Lipopolysaccharide endotoxins. Annu Rev Biochem. 2002;71:635–700.10.1146/annurev.biochem.71.110601.135414
- Fujigaki H, Saito K, Fujigaki S, et al. The signal transducer and activator of transcription 1a and interferon regulatory factor 1 are not essential for the induction of indoleamine 2,3-dioxygenase by lipopolysaccharide: involvement of p38 mitogen-activated protein kinase and nuclear factor-κb pathways, and synergistic effect of several proinflammatory cytokines. J Biochem. 2006;139:655–662.10.1093/jb/mvj072
- Wang Y, Lawson MA, Dantzer R, et al. LPS-induced indoleamine 2,3-dioxygenase is regulated in an interferon-γ independent manner by a JNK signaling pathway in primary murine microglia. Brain Behav Immun. 2010;24:201–209.
- Karin M, Greten FR. NF-κB: linking inflammation and immunity to cancer development and progression. Nat Rev Immunol. 2005;5:749–759.10.1038/nri1703
- Zhang W, Liu HT. MAPK signal pathways in the regulation of cell proliferation in mammalian cells. Cell Res. 2002;12:9–18.10.1038/sj.cr.7290105
- Guillemin GJ, Brew BJ, Noonan CE, et al. Indoleamine 2,3-dioxygenase and quinolinic acid Immunoreactivity in Alzheimer’s disease hippocampus. Neuropathol Appl Neurobiol. 2005;31:395–404.10.1111/nan.2005.31.issue-4
- Maes M, Yirmyia R, Noraberg J, et al. The inflammatory & neurodegenerative (I&ND) hypothesis of depression: leads for future research and new drug developments in depression. Metab Brain Dis. 2009;24:27–53.10.1007/s11011-008-9118-1