Abstract
Inclusion of the two isomers of citral (E-citral and Z-citral), components of lemongrass oil, was investigated within the confines of various cyclodextrin (α-CD, β-CD and γ-CD) host molecules. Aqueous complex formation constants for E-citral with α-CD, β-CD and γ-CD were determined to be 123, 185, and 204 L/mol, respectively, whereas Z-citral exhibited stronger affinities (157, 206, and 253 L/mol, respectively). The binding trend γ-CD > β-CD > α-CD is a reflection of the more favorable geometrical accommodation of the citral isomers with increasing cavity size. Encapsulation of lemongrass oil within CDs was undertaken through shaking citral:CD (1:1, 1.5:1, and 2:1 molar ratio) mixtures followed by spray drying. Maximum citral retention occurred at a 1:1 molar ratio with β-CD and α-CD demonstrating the highest levels of total E-citral and Z-citral retention, respectively. Furthermore, the β-CD complex demonstrated the slowest release rate of all inclusion complex powders.
Graphical Abstract
The inclusion between two isomers of citral (E-citral and Z-citral) in lemongrass oil and cyclodextrins by spray drying were studied.
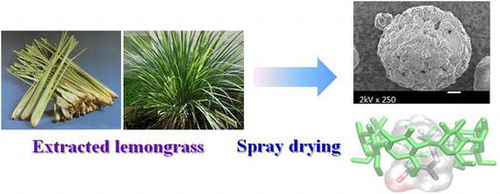
Lemongrass (Cympopogon citratus Stapf) is widely cultivated in Thailand and is extensively employed as an ingredient in Thai cuisine. Citral (3,7-dimethyl-2,6-octadienal), the major component of extracted lemongrass oil (65–80% by weight) is a mixture of two geometric isomers, geranial (trans-citral, E-citral) and neral (cis-citral, Z-citral). Although citrals have been reported to exhibit high antimicrobial activities, they are poorly water solubleCitation1–3) and lose their therapeutic properties on oxidation when exposed to heat, air, and lightCitation4,5). Encapsulation of citral is thus a potential method for enhancing its stability and enabling its biological properties to be retained over timeCitation6–8).
Encapsulation is a process in which core materials are enclosed within the confines of a coating material, most often a molecular containerCitation9,10). Cyclodextrins (CDs), cyclic oligosaccharides produced by enzymatic degradation of starch and possessing a doughnut-shaped ring structure,Citation11,12) have been widely utilized in encapsulation studies. The most commonly used CDs are α-CD, β-CD, and γ-CD, which consist of cyclic arrangements of 6, 7, or 8 glucopyranose units, respectively. While the exterior of CDs is hydrophilic allowing for a degree of water solubility, the central cavity is hydrophobic and permits inclusion complex formation when guest molecules, or part thereof, can fit appropriately and bind inside the cavity. The ability of the CDs to form an inclusion complex with the guest molecule depends upon matching of the cavity size and guest dimensions, and the occurrence of intermolecular forces such as van der Waals forces, hydrophobic interactions, and hydrogen bonds to enable host–guest bindingCitation13,14). For the three CDs mentioned above, the cavity sizes follow the order α-CD < β-CD < γ-CD, so it is necessary to consider carefully the dimensions of CD and guest to enable prediction of complexation behavior. For example, guest molecules having low molecular weights may be comfortably accommodated inside any of these CDs although it is possible that more than one guest molecule may reside inside the cavity. In contrast, guest molecules with higher molecular weights may be bound by more than one CD molecule, or have only one guest per cavity. Inclusion may also enable the reactivity of guest molecules to be tailored in the absence of chemical modificationsCitation15,16).
The aim of this study was to investigate inclusion complex formation between two isomers of citral within CD host molecules, and assess the effect of citral:CDs molar ratio on total citral retention. Furthermore, probing the release characteristics and antimicrobial activities of the spray dried complexes was undertaken with a view toward assessing their utility in practical applications.
Materials and methods
Materials
α-CD, β-CD, and γ-CD were purchased from Ensuiko Sugar Chemical Co. (Yokohama, Japan). Citral was purchased from Sigma-Aldrich Co. Ltd., (St. Louis, USA). Lemongrass oil was purchased from Thai-China Flavors and Fragrances Industry (Nonthaburi, Thailand). Chloroform and cyclohexanone were purchased from Wako Pure Chemical Industry (Osaka, Japan). The micro-organism used in antimicrobial testing was Candida albicans ATCC 10231, which was obtained from the Department of Medical Sciences (Ministry of Public Health, Thailand). Sabouraud dextrose broth (SDB) and Sabouraud dextrose agar (SDA) were from Difco (New Jersey, USA). Tween 80 (Polyoxyethylene sorbitan mono-oleate) was from VWR International Co. Ltd. (Pennsylvania, USA). Sodium chloride (NaCl) was obtained from Fisher Scientific (Leicestershire, UK). The deionized (DI) water was produced using a MilliQ Plus system (Millipore, Schwalbach, Germany).
Phase solubility study
The phase solubility study was conducted as described by Higuchi and ConnorsCitation17). An excess amount of lemongrass oil (80 μL) was added, in separate experiments, into gas-tight vials containing an aqueous solution of CD (α-CD, β-CD, or γ-CD, concentration 0–10 mmol/L). The vials were shaken at 45 °C for 48 h to ensure equilibrium, and then the supernatants were separated by centrifugation (Kubota, Japan) at 10,000 rpm for 15 min. The supernatants (4 mL) were mixed with chloroform solution (1 mL, 1 μL/mL in cyclohexanone) in a sealed glass tube. The solutions were heated in a water bath at 90 °C for 30 min, with vortexing for 30 s after each 10-min interval. After this time, a 1-μL aliquot of the organic phase containing citrals was analyzed by gas chromatography (GC 2010, Shimadzu Corporation, Japan). Gas chromatography conditions were as follows: injector and detector temperatures 210 and 230 °C, respectively, initial column temperature 50 °C for 1 min followed by heating (10 °C/min) to 230 °C. From the phase solubility diagram the complex formation constant, KC, could be calculated in accordance with Equation (Equation1(1) ), as applied for the formation of a 1:1 inclusion complex.
(1)
Preparation of encapsulated spray-dried powder
All encapsulated powders were produced by spray drying, using α-CD, β-CD, or γ-CD as encapsulating agents. The inclusion complexes between CDs and citrals were prepared from a CD slurry (20 wt% solid in water). Lemongrass oil was added into the CD slurry at such a quantity to afford a predetermined citrals:CD molar ratio (1:1, 1.5:1, or 2:1). The mixtures were then shaken at 250 rpm, 45 °C for 12 h, followed by homogenization at 8000 rpm for 3 min using a high-speed homogenizer (Polytron PT 3100, Kinematica, Japan) affording the liquid feed for spray drying. The encapsulated powders were formulated using a spray dryer (Ohkawara Kakouki, Yokohama, Japan) operating under the following conditions: atomizer rotary speed 30,000 rpm, feed rate 30 mL/min, air flow rate 110 kg/h, inlet temperature 160 °C.
Determination of total citrals retention
The citrals content in each spray-dried powder was determined by solvent extraction with chloroform. The spray-dried powder (50 mg) was dissolved in distilled water (4 mL) and chloroform (1 mL), in a sealed glass tube. The solutions were heated in a water bath at 90 °C for 30 min, with vortexing for 30 s after every 10-min interval. The organic phase (1 μL) containing volatile compounds was analyzed by gas chromatography (GC 2010, Shimadzu Corporation, Japan) with quantification of citrals levels being determined as in the phase solubility study. The total citrals retention during spray drying is defined as the ratio of the quantity of citrals in the encapsulated powder on a dry basis relative to the original citrals level in the feed liquid.
SEM morphology investigations
A scanning electron microscope (JEOL JSM 6060, EVISA, Japan) was used to investigate the microstructure of the inclusion complexes. Spray-dried powders were placed on the SEM holder and coated with Pt–Pd using a magnetron sputter coater. The samples were then analyzed with the SEM operating at an accelerating voltage of 15 kV.
Release characteristics of citrals from spray-dried powders
The spray-dried powder (50 mg) were kept in an open bottle and placed in the chamber (BTL-433, Espec, United Kingdom) for 7 days at 37 °C and 52% RH. After this time, the powder was analyzed for residual amounts of citrals using the extraction method as described above. The citrals retention in the control release experiment was defined as the percentage of citrals content at each time relative to the initial citrals content, and the release rate constant of citrals under the experimental conditions could be derived from the Avrami equation (Equation (Equation2(2) )).
(2)
In this equation, R represents the retention of the volatile, t is the release time, k is the release mechanism, and n is a parameter representing the release mechanism.
In vitro antimicrobial activity
The minimum inhibitory concentration (MIC) and the minimum bactericidal concentration (MBC) of citrals and lemongrass oil were firstly determined by microdilution broth testing against C. albicans ATCC 10231. Briefly, stock solutions of citrals and lemongrass oil were prepared at a concentration of 2,000 μg/mL (in 0.05% v/v Tween 80). All samples were transferred into 96-well plates and serial dilutions performed. Inoculums consisting of 106 CFU/mL were added into each well, followed by incubation at 37 °C for 48 h. Microbial growth was measured using a microplate reader (Molecular Devices, California, USA) at 600 nm. The MIC is defined as the lowest concentration of an antibacterial agent inhibiting visible growth, whereas MBC represents the lowest concentration of an antibacterial agent required for lethality. Following MIC/MBC determination, the antimicrobial activities of spray-dried powders (at the highest total citrals retention) against C. albicans ATCC 10231 were assessed. The spray-dried powders were sterilized with UV irradiation for 1 h and then dissolved in SDB (0.05% v/v Tween 80) at a quantity corresponding to the MIC value. Inoculums consisting of 106 CFU/mL were added to the samples, and the treatment efficiency analyzed after incubation for 0, 1, 3, 5, and 7 h at 37 °C. At each of these times, inoculums were diluted with aqueous NaCl solution (0.85 wt% solid) and each dilution was spread onto SDA by spiral plattering (Spiral Biotech Autoplate 4000, USA), followed by further incubation at 37 °C for 48 h. Antimicrobial activities were measured by counting of viable colonies on the SDA surface. The treatment efficiency was defined as the percentage reduction (% Reduction) in bacterial growth, as expressed by Equation (Equation3(3) ):
(3)
where % Reduction represents the percentage reduction in microbial population. A is the number of colonies on media inoculated with microbes and B is the number of colonies on media with containing microbes and spray-dried powder.
Results and discussion
Phase solubility study
Phase solubility studies are useful for allowing fundamental predictions of inclusion complex formation to be made. Using this method, as reported by Higuchi and Connors,Citation17) the stoichiometry of the inclusion complex and its formation constant were determined in water at various CD concentrations. From this protocol, two types of phase solubility diagram can be obtained: type A (AL, AN, AP solubility curves) when the solubility of the guest molecule increases with increasing CD concentration and type B (Bs, and Bi solubility curves) representing the formation of complexes having limited solubility in aqueous media. The phase solubility profiles for complexes formed between citrals (E-citral and Z-citral) and CDs at 45 °C are illustrated in Fig. . The aqueous solubilities of E-citral and Z-citral alone are approximately 4 mmol/L. Addition of CDs (2–10 mmol/L) to citrals resulted in enhancements in their water solubility, a consequence of inclusion complex formation. Complexation between α-CD and citrals afforded a linear solubility diagram defined as AL typeCitation17), with 1:1 complex stoichiometry. As the relationship is linear the formation constant can be calculated from the slope of the phase solubility diagram (Equation Equation1(1) ). Solubility profiles for citral complex formation with β-CD or γ-CD are consistent with the BS type,Citation17) as the solubilities of both E-citral and Z-citral increased with β-CD and γ-CD concentration up to 4 mmol/L, and subsequently remained constant. Haiyee et al.Citation18) and Waleczek et al.Citation19) previously reported the stoichiometry of BS type interactions could be assumed as being 1:1, with the complex formation constant derivable from the initial slope. The formation constants between E-citral and α-CD, β-CD, and γ-CD at 45°C were 123, 185, and 204 L/mol, respectively, although Z-citral showed slightly higher affinity for CD binding (157, 207, and 253 L/mol, respectively) under identical conditions. The binding strength trend implies that larger cavities allow for more favorable geometrical accommodation of the citrals, as reflected by the order γ-CD > β-CD > α-CD. Our formation constant results are the binding trend similar to those reported by Decock et al.Citation20), who report the formation constant between E-citral and α-CD and β-CD were 66 and 728 L/mol, respectively. Moreover, the complex formation constants between Z-citral and α-CD and β-CD were 93 and 693 L/mol, respectively.
The effect of CD type and citrals:CD mole ratio on total citrals retention
As reported earlier the major components of extracted lemongrass oil are E-citral (41.6% w/w) and Z-citral (31.78% w/w)Citation21) although these components are poorly water soluble and volatile. Accordingly, it was of interest to investigate the level of citral retention after encapsulation and this was achieved by varying the citrals:CD molar ratio during the fabrication process prior to quantification of citrals levels using gas chromatography. Figure indicates that the total citrals retention decreased as loading levels increased, consistent with citrals being fully bound at low loadings and the presence of unbound citral in the powder at high citral:CD molar ratios. Higher citral:CD ratios result in CD cavities exhibiting full occupancyCitation22,23) with excess citrals being loosely held within the powder structure, resulting in loss during spray dryingCitation24). The maximum total citrals retention was observed at a citrals:CD molar ratio of 1:1. Furthermore, the degree of total retention of E-citral in CDs follows the order β-CD > γ-CD > α-CD, different to that of Z-citral (α-CD > β-CD > γ-CD)Citation25). According to the phase solubility results, the aqueous complex formation constants between citrals and CDs follow the order γ-CD > β-CD > α-CD, implying that γ-CD best accommodates citral guest molecules. However, the lower retention in γ-CD is a consequence of the less than optimal fit of the entire citral molecule within the cavityCitation25), resulting in their loss during spray drying and accounting for the lower total retention in this host system.
Scanning electron microscopy
The morphologies of all particles were investigated using scanning electron microscopy (SEM). Figure (a)–(c) shows SEM images of native α-CD, β-CD, and γ-CD particles, respectively. While the crystalline structure of native CDs is evident the encapsulated spray-dried powders prepared from α-CD and γ-CD resemble spherical aggregates (Fig. (d)–(f)), which occur as a result of agglomeration during spray drying. It is possible that the water solubility of CDs (γ-CD > α-CD > β-CD) may play a role in their dissolution, and the formation of inter-particle bridges at particle contact points on re-dryingCitation26). In contrast, spray-dried powder prepared from β-CD is observed to form crystalline particles, possibly due to the lower solubility of this host in comparison to other CDs.
Release characteristics of spray-dried powders
The controlled-release behavior of the encapsulated spray-dried powders (1:1 citrals:CD) was examined at 37 °C and 52% relative humidity. Figure highlights citrals release being more rapid in all CD systems during the first day of storage, with the release rate subsequently slowing and reaching a near plateau toward the end of the study period (c.a. 5 days). Considering the effect of CD type on citrals retention, the release rate of both citrals included in β-CD was lower than for that bound in other CDs. These results are consistent with those of total citral retention as discussed earlier, and indicate preferential inclusion of citrals within β-CD over the other systemsCitation27). Moreover, E-citral had a higher release rate than Z-citral. This result may be due to Z-citral could perfectly fit inside CDs cavity better than E-citral, similarly results with Ruktanonchai et al.Citation21).
Fig. 4. Release behavior of E-citral (a) and Z-citral (b) from CD inclusion complexes at 37 °C, 52% relative humidity. α-CD (△), β-CD (○), γ-CD (□). Dashed lines represent calculated values by Avrami Equation.
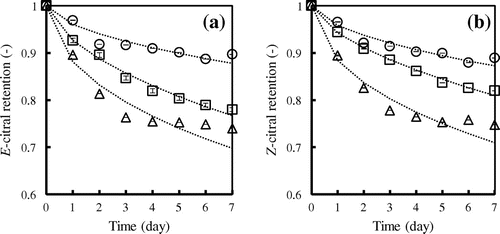
Although both geometrical isomers of citral have the same molecular weight, their size difference may impart some effects on the release mechanism. The Avrami equationCitation28,29) (Equation Equation2(2) ) was used to evaluate the release rate constant, and hence the mechanism, of citrals release. Plotting ln(–lnR) (y-axis) against lnt (x-axis) afforded the Avrami parameters n and k, which are given in Table . Values of n < 1 indicated that release of included citrals from the complexes corresponds to the diffusion-limited kinetic model. The mechanism of molecular diffusion can be explained by the presence of water in the powder, which interacts with the polar surface of the CDs. Over time, citral molecules can undergo replacement by water which represents a reverse situation to that of inclusion complex formation. The higher values of k for α-CD and γ-CD complexes than β-CD complexes indicate greater levels of diffusion in the former systems, which is consistent with β-CD being able to encapsulate citrals more effectively as discussed earlier.
Table 1. Release rate constants (k) and parameters representing the release mechanism (n) of E-citral and Z-citral in lemongrass oil from CD inclusion complexes at 37 °C and 52% RH.
Antimicrobial activities of spray-dried powders
As the minimum inhibitory (MIC) and MBCs obtained from pure citrals (250 and 1000 μg/mL, respectively) proved similar to those of lemongrass oil this suggests that these are the major antimicrobial components in the oil itself. Figure highlights the impact of included citrals on the percentage reduction in growth of C. albicans as a function of time. Based on these results, α-CD complexes show the most pronounced antimicrobial effects, with 91.65% reduction in microbial levels after 1 h, and 100% after 3 h exposure. These results are consistent with the release rate order for included citrals (α-CD < γ-CD < β-CD), and indicate that the antimicrobial activities of both E-, and Z-citral are retained during the encapsulation process. In related work, Leimann et al.Citation1) have reported the MIC values of microencapsulated citrals toward E. coli (22.32 mg/mL), and S. aureus (2.79 mg/mL).
Conclusion
Molecular encapsulation within the cavity of CDs is an effective method for preventing the loss of citrals during spray drying. α-, and β-CDs proved most efficient for the encapsulation of E-citral and Z-citral, respectively, with all CDs showing maximum total citrals retention at a 1:1 M ratio of citrals:CD. The encapsulation efficiency was found to not be solely dependent on the complex formation constant, but was also influenced by the spray drying process. On storage, β-CD complexes exhibited slower release rates than the γ-CD, and α-CD complexes, with citral release being consistent with the limited diffusion kinetic model.
Funding
The work was financially supported by the Chulalongkorn University [Contract No. RES_57_411_21_076]; The Thailand Research Fund [grant number DPG5880003], [grant number IRG5780014].
Disclosure statement
No potential conflict of interest was reported by the authors.
Acknowledgment
We acknowledge the Center of Excellence in Particle Technology, Chulalongkorn University, Kagawa University, and National Nanotechnology Center, National Science and Technology Development Agency for providing facilities for this work.
References
- Leimann FV, Gonçalves OH, Machado RAF, et al. Antimicrobial activity of microencapsulated lemongrass essential oil and the effect of experimental parameters on microcapsules size and morphology. Mat Sci Eng. 2009;29:430–436.10.1016/j.msec.2008.08.025
- Silva CB, Guterres SS, Weisheimer V, et al. Antifungal activity of the lemongrass oil and citral against Candida spp. Braz J Infect Dis. 2008;12(1):63–66.
- Tzortzakis NG, Economakis CD. Antifungal activity of lemongrass (Cympopogon citratus L.) essential oil against key postharvest pathogens. Innov Food Sci Emerg Technol. 2007;8:253–258.10.1016/j.ifset.2007.01.002
- Ueno T, Masuda H, Ho CT. Formation mechanism of p-methylacetophenone from citral via a tert-alkoxy radical intermediate. J Agric Food Chem. 2004;52:5677–5684.10.1021/jf035517j
- Kimura K, Nishimura H, Iwata I, et al. Deterioration mechanism of lemon flavor. 2. Formation mechanism of off-odor substances arising from citral. J Agric Food Chem. 1983;31:801–804.10.1021/jf00118a030
- Lu Z, Cheng B, Hu Y, et al. Complexation of resveratrol with cyclodextrins: solubility and antioxidant activity. Food Chem. 2009;113:17–20.10.1016/j.foodchem.2008.04.042
- Jafari SM, Assadpoor E, He Y, et al. Encapsulation efficiency of food flavours and oils during spray drying. Drying Tech. 2008;26:816–835.10.1080/07373930802135972
- Zhang A, Liu W. Inclusion effect of alpha-cyclodextrin on chemical degradation of malathionin water. Arch Environ Contam Toxicol. 2008;54(3):355–362.10.1007/s00244-007-9055-8
- Jafari SM, Assadpoor E, Bhandari B, et al. Nano-particle encapsulation of fish oil by spray drying. Food Res Int. 2009;41:172–183.
- Madene A, Jacquot M, Scher J, et al. Flavour encapsulation and controlled release – a review. Int J Food Sci Tech. 2006;41:1–21.10.1111/ifs.2006.41.issue-1
- Szejtli J. Past, present, and future of cyclodextrin research. Pure Appl Chem. 2004;76:1825–1845.
- Li Z, Wang M, Wang F, et al. γ-cyclodextrin: a review on enzymatic production and applications. Appl Microbiol Biotechnol. 2007;77:245–255.10.1007/s00253-007-1166-7
- Astray G, Gonzalez-Barreiro C, Mejuto JC, et al. A review on the use of cyclodextrins in foods. Food Hydrocolloids. 2009;23:1631–1640.10.1016/j.foodhyd.2009.01.001
- Del Valle EMM. Cyclodextrins and their uses: a review. Process Biochem. 2004;39:1033–1046.10.1016/S0032-9592(03)00258-9
- Karathanos VT, Mourtzinos I, Yannakopoulou K, et al. Study of the solubility, antioxidant activity and structure of inclusion complex of vanillin with β-cyclodextrin. Food Chem. 2007;101:652–658.10.1016/j.foodchem.2006.01.053
- Sajeesh S, Sharma CP. Cyclodextrin–insulin complex encapsulated polymethacrylic acid based nanoparticles for oral insulin delivery. Int J Pharm. 2006;325:147–154.10.1016/j.ijpharm.2006.06.019
- Higuchi T, Connors KA. Phase-solubility techniques. Adv Anal Chem Inst. 1965;4:117–212.
- Haiyee ZA, Saim N, Said M, et al. Characterization of cyclodextrin complexes with turmeric oleoresin. Food Chem. 2009;114:459–465.10.1016/j.foodchem.2008.09.072
- Waleczek KJ, Marques HMC, Hempel B, et al. Phase solubility studies of pure (−)-α-bisabolol and camomile essential oil with β-cyclodextrin. Euro J Pharm Biopharm. 2003;55:247–251.10.1016/S0939-6411(02)00166-2
- Decock G, Landy D, Surpateanu G, et al. Study of the retention of aroma components by cyclodextrins by static headspace gas chromatography. J Inclusion Phenom Macrocyclic Chem. 2008;62:297–302.10.1007/s10847-008-9471-z
- Ruktanonchai UR, Srinuanchai W, Saesoo S, et al. Encapsulation of citral isomers in extracted lemongrass oil with cyclodextrins: molecular modeling and physicochemical characterizations. Biosci Biotech Biochem. 2011;75:2340–2345.10.1271/bbb.110523
- Toro-Sanchez CL, Ayala-Zavala JF, Machi L, et al. Controlled release of antifungal volatiles of thyme essential oil from β-cyclodextrin capsules. J Inclusion Phenom Macrocycl Chem. 2010;67:431–441.10.1007/s10847-009-9726-3
- Fernandes LP, Oliveira WP, Sztatisz J, et al. Solid state studies on molecular inclusions of Lippia sidoides essential oil obtained by spray drying. J Therm Anal Cal. 2009;95:855–863.10.1007/s10973-008-9149-1
- Liu XD, Furuta T, Yoshii H, et al. Cyclodextrin encapsulation to prevent the loss of l -menthol and its retention during drying. Biosci Biotechnol Biochem. 2000;64:1608–1613.10.1271/bbb.64.1608
- Reineccius TA, Reineccius GA, Peppard TL. Encapsulation of flavors using cyclodextrins: comparison of flavor retention in Alpha, Beta, and Gamma types. J Food Sci. 2002;67:219–225.
- Buffo RA, Probst K, Zehentbauer G, et al. Effects of agglomeration on the properties of spray-dried encapsulated flavours. Flavour Fragr J. 2002;17:292–299.10.1002/(ISSN)1099-1026
- Reineccius TA, Reineccius GA, Peppard TL. Comparison of flavor release from alpha-, Beta- and Gamma-cyclodextrins. J Food Sci. 2003;68:1234–1239.
- Yoshii H, Soottitantawat A, Liu XD, et al. Flavor release from spray dried maltodextrin/gum arabic or soy matrices as a function of storage relative humidity. Innovative Food Sci Emerg Tech. 2001;2:55–61.10.1016/S1466-8564(01)00019-4
- Soottitantawat A, Takayama K, Okamura K, et al. Microencapsulation of l-menthol by spray drying and its release characteristics. Innovative Food Sci Emerg Tech. 2005;6:163–170.10.1016/j.ifset.2004.11.007