Abstract
Daily activity rhythms that are dominated by internal clocks are called circadian rhythms. A central clock is located in the suprachiasmatic nucleus of the hypothalamus, and peripheral clocks are located in most mammalian peripheral cells. The central clock is entrained by light/dark cycles, whereas peripheral clocks are entrained by feeding cycles. The effects of nutrients on the central and peripheral clocks have been investigated during the past decade and much interaction between them has come to light. For example, a high-fat diet prolongs the period of circadian behavior, a ketogenic diet advances the onset of locomotor activity rhythms, and a high-salt diet advances the phase of peripheral molecular clocks. Moreover, some food factors such as caffeine, nobiletin, and resveratrol, alter molecular and/or behavioral circadian rhythms. Here, we review nutrients and food factors that modulate mammalian circadian clocks from the cellular to the behavioral level.
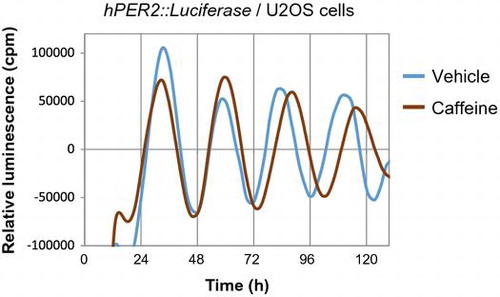
Nutrients and food factors affect phase, amplitude, and period length of circadian clocks, especially in peripheral tissues.
Morning sunlight awakens diurnal animals, whereas sunset evokes sleep. Human activity rhythms are ruled by circadian clocks. Many living creatures have evolved circadian clock systems composed of clock genes, though these are not necessarily conserved beyond kingdoms. The mammalian core clock genes, Per, Cry, Bmal, and Clock form transcriptional negative feedback loops, that generate 24-h oscillations in transcripts.Citation1) The circadian oscillation of clock genes is evident in most tissues throughout the body, and thus cellular activities are differentially tuned between day and night. Nutritional intake is typically available only during the active phase and therefore, the active/rest phase switches the speed of anabolism/catabolism. Indeed, many critical genes associated with energy metabolism regulation, especially nuclear receptors, are expressed in a circadian manner under regulation by clock genes.Citation2,3)
The circadian clock in the suprachiasmatic nucleus (SCN) within the hypothalamus is called the central clock because its destruction disrupts circadian sleep/wake patterns in rodents independently of the total amount of sleep each day.Citation4) The phase of the central clock is synchronized to surrounding light/dark (LD) cycles by signal input from the optic nerve and thus the phases of the central clock are similar, although photosensitivity and electrical activity differs, between diurnal and nocturnal animals.Citation5,6) Other circadian clocks throughout the body are called peripheral clocks and these are entrained by feeding cycles. Therefore, the phases of clocks and the subsequent functional rhythms of metabolism are opposite between diurnal and nocturnal animals.Citation7) Indeed, imposing daytime feeding in nocturnal mice enables phase reversal as diurnal animals, but does not alter the central clock.Citation8,9) These findings show that feeding cycles comprise predominant cues for peripheral clocks regardless of the central clock. This uncoupling between the central and peripheral clocks probably enables a switch between diurnality and nocturnality depending on surrounding circumstances such as food availability, predators, and climate. Furthermore, such switching among similar species has frequently occurred during evolution,Citation10) suggesting that trivial genomic changes result in a substantial change in the timing of activity.
I. Molecular mechanism of resetting circadian clocks
I.i. Central clock
The circadian clock in the SCN is entrained by LD information. Light signals before the predicted sunrise and after the predicted sunset reset the circadian phase forward and backward, respectively, in the SCN. Because part of the optic nerve directly projects into the SCN, light signals are transmitted into SCN via neurotransmitters such as glutamate and pituitary adenylate cyclase-activating peptide.Citation11) Glutamate increases the intracellular cAMP concentration and acutely induces the Per1 gene that harbors a cAMP-responsive element in its promotor region, which results in a phase shift of the clock. The experimental administration of glutamate, N-methyl-D-aspartate (NMDA), or nitric oxide (NO) generators mimics the phase shift by light in cultured SCN slices.Citation12) Modulation of the SCN affects all clocks throughout the body because the clocks in the SCN relay phase information to peripheral clocks via a combination of neural, humoral, and behavioral signals.
Light signals reset the central clock but adjustments can only occur for a few hours each day. Jet lag notably continues for several days after long-distance air travel to the opposite side of the planet. Because the SCN neurons couple with each other, interneuronal communication probably buffers and weakens light signals that are transmitted into only a subset of SCN neurons. Vasopressin is responsible for interneuronal communication in the SCN, and mice lacking vasopressin receptors do not require time to adjust to a new LD condition.Citation13) Moreover, antagonists for V1a and V1b vasopressin receptors in wild-type mice can enhance the speed of re-entrainment to a different LD condition. All the above information together indicates that chemicals can entrain or enhance resetting of the central clock.
I.ii. Peripheral clocks
Most cells including even some cultured lines such as Rat-1 fibroblasts, NIH3T3 fibroblasts, and U2OS osteosarcoma cells have molecular circadian clocks.Citation14–16) Similarly, most peripheral tissues show circadian oscillation of clock genes,Citation17) indicating that cell clocks are synchronized locally at least at the tissue level. Actually, the phases of peripheral clocks are similar among many tissues and are predominantly dependent on feeding cycles.Citation8,9,18) Cell clocks are easily desynchronized in culture in contrast to the central clock that couples and synchronizes with itself, indicating that systemic cues constantly entrain peripheral cell clocks in vivo. The speed of entrainment to a novel feeding regimen notably differs among tissues; for example, the liver takes a few days, whereas the heart and lungs take about one week to become entrained.Citation8,9) One explanation for this is differential sensitivity to synchronizers such as insulin. Food intake induces the secretion of insulin that resets some peripheral clocks.Citation19–21) Insulin induces acute Per2 expression in some tissues including liver, adipose, and muscle. Indeed, insulin shifts the circadian phase in explanted liver cultures and adipose tissues, but not in lung tissues (Fig. (A) and (B)).Citation21) Although insulin is a key element for feeding-dependent entrainment of peripheral clocks, mice or rats administered with streptozotocin that induces β-cell death in the pancreas, do not secrete insulin yet feeding-dependent entrainment persists.Citation22,23) These findings indicate that other factors also play roles in the entrainment of peripheral clocks. Rhythms of body temperature that typically correlate with those of feeding, might synchronize a wide range of tissues because temperature cycles entrain the clock in cultured fibroblasts and some peripheral tissues (Fig. (C)).Citation24–27) Physiological levels of oxygen rhythms also HIF1α dependently reset clocks in cultured cells.Citation28) Glucocorticoid also affects the phase of many peripheral clocks (Fig. (D)), and blood concentrations of this steroid hormone oscillate in a circadian manner dependently on the adrenal clock.Citation29,30) Even physical and psychological stress stimuli that induce acute elevations of blood glucocorticoid, can entrain peripheral clocks in mice.Citation31) Oxyntomodulin, a peptide hormone released from gut L cells after nutrient ingestion, is also a candidate synchronizer as it resets the mouse liver clock.Citation32)
Fig. 1. Circadian phase shift by stimuli in cultured mouse explants.
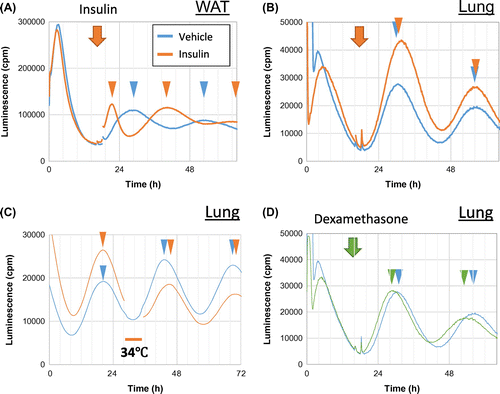
Because each peripheral clock responds to several circadian cues, the effect of a single synchronizer might be buffered and weakened by other cues. Thus, entraining peripheral clocks to a new feeding regimen can typically take more than a few days though depending on the tissues. In contrast, a single stimulus, such as insulin administered to the adipose tissue, can reverse the clock phase under the experimental exclusion of many circadian cues in vitro (Fig. (A)).
I.iii. Chemicals that modulate molecular clocks
Chemical compounds that can alter the circadian period or phase in human U2OS cells have been searched by the high-throughput screening of 1,280 pharmacologically active compounds with diverse structures.Citation33) Small-molecule inhibitors of glycogen synthase kinase 3 (GSK3) are found to shorten the circadian period. It is noteworthy that lithium inhibits GSK3 and lengthens the circadian period,Citation34) and that the activity of shaggy, a homolog of GSK-3, affects the circadian period in Drosophila.Citation35) A different screen of ~60,000 compounds found that KL001 activates the core clock gene CRY and lengthens the circadian period in U2OS cellsCitation36), and another study revealed that some KL001 derivatives shorten or lengthen the circadian period in these cells.Citation37) A screen of 1,260 pharmacologically active compounds showed that inhibitors of casein kinase Iε and/or Iδ (CKIε/δ) lengthen the circadian period in NIH3T3 and U2OS cell lines.Citation38) Some mutations of the CKIε/δ gene and their target PER2 phosphorylation sites have either a short or a long circadian period in Drosophila, hamsters, mice, and humans, indicating that CKIε/δ activity and subsequent PER2 phosphorylation correlates with the length of the circadian period.Citation39–42) The results of these screens indicated that small molecules such as modulators of GSK3, CRY, CKIε/δ, and PER2 regulate the circadian period.
II. Nutrients and food factors that modulate circadian clocks
Nutritional signals modulate cellular circadian clocks or clock systems throughout the body. Some specific nutrients and food factors are also reported to affect circadian clocks (Table ). A high-fat diet in mice under constant darkness prolongs circadian locomotor rhythms that are referred to as free-running rhythms.Citation43) The mechanism remains obscure, but a high dietary intake of fat impacts at least feeding behaviors, large-scale oscillation of hepatic transcripts, and circulating serum lipids.Citation43–45) A high-fat diet PPARγ dependently reorganizes hepatic transcript oscillation Citation44) and PPARδ dependent lipogenesis in the liver regulates muscle usage of fatty acid via PPARα activation.Citation45) Changing the activity of these PPARs can affect circadian rhythms because PPARs control both circadian clocks and lipid homeostasis.Citation46) Activating PPARα using bezafibrate, a drug that helps to treat hyperlipidemia, advances the onset of circadian behavioral rhythms dependently on photoperiod,Citation47,48) and mice without PPARγ or PPARγ coactivator 1α (PGC1α) have altered circadian behavior and energy metabolism.Citation49,50) Metabolic status seems to couple with the behavioral circadian rhythm, that might be integrated by PPARs. Notably, starvation or a ketogenic diet (low-carbohydrate and high-fat) that mimics starvation, advances the onset of daily behavioral rhythms in mice.Citation51,52)
Table 1. Nutrients and food factors that modulate circadian clocks.
Consuming a high-salt diet (4% or 8% NaCl) for more than two weeks advances liver, kidney, and lung clocks in mice.Citation53) Because the rhythms of feeding and drinking behaviors are not affected by high-salt diets, the central clock is not affected. The increase in blood glucose after consuming a high-salt diet is more acute and higher than that after consuming a normal diet, suggesting that the feeding signals to reset peripheral clocks are powerful. The absorption of nutrients from the small intestine depends on sodium concentrations because many transporters of nutrients are co-transporters with Na+ such as sodium/glucose cotransporters (SGLT). Moreover, a high-salt diet increases gene expression of the glucose transporters, Glut2 and Sglt1, in the jejunum, indicating that at least glucose absorption is increased by such a diet. Similarly, the rapid digestion of a starch that causes higher levels of blood glucose and insulin also exerts more powerful entrainment of the mouse liver clock than normal starch.Citation54) Furthermore, fish oil containing docosahexaenoic acid (DHA) and eicosapentaenoic acid (EPA) also accelerates the entrainment of mouse liver clocks by increasing insulin secretion via GPR120.Citation55) The administration of L-ornithine at the early inactive period increases plasma levels of insulin and glucagon-like peptide-1 and advances peripheral clocks in mice.Citation56) Thus, blood glucose uptake and subsequent insulin and/or incretin secretion are key factors for the feeding-dependent entrainment of peripheral clocks, especially those in the liver. Some functional factors in foods modulate molecular circadian clocks. Resveratrol is a grape polyphenol that alters molecular clocks in cultured fibroblasts.Citation57) Adding resveratrol to culture medium delayed the phase of the circadian clock in Rat-1 cells (Fig. ). Caffeine delays the phase of circadian activity through ryanodine receptors in mouse brain slices containing the SCN.Citation58) We showed that caffeine lengthens the circadian rhythm of mouse NIH3T3 cells, human osteosarcoma U2OS cells (Fig. ) and tissue explants of mouse livers.Citation59) Moreover, mice that consume caffeine or coffee ad libitum dose dependently develop prolonged circadian locomotor behavior.Citation59) Caffeine has also caused a phase shift in a cultured cell line and in mice,Citation60) and delays circadian melatonin rhythms in humans.Citation61) The fact that caffeine and/or the caffeine analog theophylline also lengthens the circadian period in Drosophila, Neurospora, Chlamydomonas reinhardtii, and Trifolium repens L.Citation62–64) suggests that caffeine affects circadian clocks at a fundamental level even though genetic clocks are not conserved among bacteria, plants, and animals. Increasing cAMP concentrations using caffeine might be a key factor in lengthening the clocks because cAMP-dependent signaling is a core pacemaker in mammals.Citation65)
Fig. 2. Resveratrol shifts circadian phase of cultured fibroblasts.
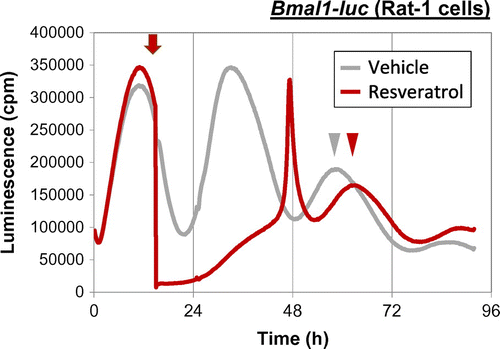
Fig. 3. Caffeine lengthens circadian period of human U2OS cells.
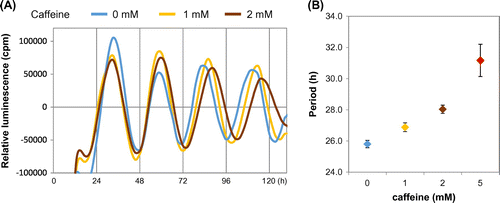
The harmala alkaloid harmine prolongs the circadian period of NIH3T3 cells, mouse embryonic fibroblasts (MEF) and differentiated neuronal cells obtained from mouse embryos, and in cultured slices of mouse SCN.Citation66,67) Harmine extends the half-life of PER2::LUC protein,Citation67) suggesting a stabilization effect on PER2 protein. Palmitate, the prevalent saturated fatty acid in high-fat diets, also prolongs the circadian period of mouse fibroblasts.Citation68) Moreover, acute administration of palmitate induced a phase advance in fibroblasts and differentiated adipocytes. Another food constituent, cinnamic acid, shortens the circadian period in differentiated neuronal cells.Citation69) Interestingly, cinnamic acid also shortens behavioral free-running activity rhythms in mice when delivered by continuous infusion from an osmotic pump.
III. Perspectives
Previous studies have revealed the molecular and systemic targets of circadian clock modulation. Mimicking entrainment cues or their signals is an effective way to interfere with circadian systems at the tissue or whole body level. Molecules affecting glutamate receptors or downstream cAMP signaling in the SCN could theoretically cause a phase-shift at the behavioral level like LD signals. Furthermore, agents that induce the secretion of clock-synchronizer hormones including insulin, oxyntomodulin, and glucocorticoids, could similarly entrain some peripheral tissues in the manner of food or stress signals. Indeed, food factors that reinforce insulin secretion such as fish oil, high-salt, and rapidly digested starches, enhance food entrainment in some peripheral tissues.Citation53–55) In contrast, suppressors of insulin signaling might mask feeding-dependent entrainment in insulin-sensitive tissues. Moreover, food factors that alter body temperature or thermosensation such as hot spices might work as phase-modulators in a wide range of peripheral tissues. Factors that alter metabolic states would also affect the circadian system at the behavioral level. A ketogenic diet that promotes lipolysis and body weight loss through mimicking starvation signals, advances the onset of behavioral rhythms,Citation51) whereas a high-fat diet with carbohydrate that imitates satiation signals, prolongs the circadian period.Citation43) Moreover, food factors regulating PPARs might modulate the circadian clock systems like bezafibrate.Citation47,48) Another target of modulating behavioral clocks is intercellular coupling in the SCN. Because neuronal coupling plays a role in resistance to becoming entrained to novel LD cycles, factors that inhibit their communication such as vasopressin signaling inhibitors enable rapid entrainment to novel LD cycles. This should help to accelerate recovery from jet lag. Yet another target is the direct modulation of intracellular molecular clocks. Chemical screening has uncovered molecules that interact with CKIε/δ, GSK-3, CRY, and PER2 that could alter the length of the intercellular circadian period. Regulators of FBXL3 and FBXL21 that theoretically control CRY stabilizationCitation70) must also be able to change the period. Caffeine, theophylline, harmine, palmitate, and cinnamic acid are modulators that work in this manner because they alter period length at the cellular level. Acute inducers of Per and/or Dec genes can similarly cause a phase-shift at the cellular level.Citation71,72) Forskolin, an activator of adenylate cyclase, is popular for clock studies as it induces Per1 expression via cAMP signaling to reset the circadian phase in many types of cells.Citation72,73) Resveratrol is probably this type modulator.Citation57) Because many phase-resetting molecules have been found by chemical screening in vitro,Citation33,38) identifying food components that can reset circadian clocks at least at the cellular level should not be difficult.
Nutritional signals are predominant factors for the entrainment of peripheral clocks that determine cellular active/rest phases. Peripheral clocks control many physiological events in a circadian manner that enables prediction and preparation for subsequent events. Thus, disrupted habits such as eating at irregular meal times can induce a homeostatic imbalance that can lead to obesity and even to lifestyle-related diseases. Recent nutritional studies linked to chronobiology called “chrono-nutrition” have revealed a molecular association between energy homeostasis and the timing of meals.Citation74) Skipping breakfast, eating late at night, and shifted mealtimes can all induce obesity even without an increase in caloric intake.Citation75–80) A systemic review of human epidemiological studies has identified a correlation between shift work and body weight gain.Citation81) In contrast, time-restricted feeding with a high-fat diet prevents the development of obesity in mice derived from consuming a sufficient amount of calories.Citation82,83) Knowledge about circadian clock modulators could help to prevent such a homeostatic imbalance, although regular eating habits is the best way to fine-tune clock rhythms. The citrus flavonoid nobiletin, enhances clock amplitude according to screening in vitro.Citation84) Administering mice with nobiletin and a high-fat diet Clock gene dependently prevents metabolic syndrome, reduction of energy expenditure, and decrease of locomotor activity. This indicates that food factors with the ability to modulate circadian clocks could help to prevent a homeostatic imbalance, although this notion is based on non-human experimental findings. Appropriate maintenance of circadian clocks is important for finely tuned physiological performance from the cellular level to the whole body, and thus to optimal health.
Disclosure statement
No potential conflict of interest was reported by the author.
Funding
This work was supported by the Japan Society for the Promotion of Science (JSPS) KAKENHI [grant number 25702005] (Young Scientists A) and 16K12746 (Challenging Exploratory Research).
Acknowledgments
I am sincerely grateful to Dr. Katsutaka Oishi (AIST), Dr. Yoshiaki Onishi (AIST), Dr. Koyomi Miyazaki (AIST), Dr. Norio Ishida (AIST), Dr. Kiyoshi Miwa (Ajinomoto Co., Inc.), Prof. Keiko Abe (Univ. of Tokyo), Dr. Masuko Kobori (NFRI), Ms. Mutsumi Sakurai (NFRI), Ms. Yukari Akimoto, and Ms. Nanako Ito (NFRI) for providing valuable advice and expert experimental support.
Notes
Abbreviations: CKI, casein kinase I; DHA, docosahexaenoic acid; EPA, eicosapentaenoic acid; GSK3, glycogen synthase kinase 3; LD, light/dark; PGC1α, PPARγ coactivator 1α; PPAR, peroxisome proliferator-activated receptor; SCN, suprachiasmatic nucleus; SGLT, sodium/glucose cotransporter
References
- Buhr ED, Takahashi JS. Molecular components of the Mammalian circadian clock. Handb Exp Pharmacol. 2013;217:3–27.
- Ribas-Latre A, Eckel-Mahan K. Interdependence of nutrient metabolism and the circadian clock system: importance for metabolic health. Mol Metab. 2016;5:133–152.10.1016/j.molmet.2015.12.006
- Yang X, Downes M, Yu RT, et al. Nuclear receptor expression links the circadian clock to metabolism. Cell. 2006;126:801–810.10.1016/j.cell.2006.06.050
- Stephan FK, Zucker I. Circadian rhythms in drinking behavior and locomotor activity of rats are eliminated by hypothalamic lesions. Proc Natl Acad Sci USA. 1972;69:1583–1586.10.1073/pnas.69.6.1583
- Challet E. Minireview: entrainment of the suprachiasmatic clockwork in diurnal and nocturnal mammals. Endocrinology. 2007;148:5648–5655.10.1210/en.2007-0804
- Ramkisoensing A, Meijer JH. Synchronization of biological clock neurons by light and peripheral feedback systems promotes circadian rhythms and health. Front Neurol. 2015;6:128.
- Kumar Jha P, Challet E, Kalsbeek A. Circadian rhythms in glucose and lipid metabolism in nocturnal and diurnal mammals. Mol Cell Endocrinol. 2015;418(Pt 1):74–88.10.1016/j.mce.2015.01.024
- Damiola F, Le Minh N, Preitner N, et al. Restricted feeding uncouples circadian oscillators in peripheral tissues from the central pacemaker in the suprachiasmatic nucleus. Genes Dev. 2000;14:2950–2961.10.1101/gad.183500
- Stokkan KA, Yamazaki S, Tei H, et al. Entrainment of the circadian clock in the liver by feeding. Science. 2001;291:490–493.10.1126/science.291.5503.490
- Gerkema MP, Davies WIL, Foster RG, et al. The nocturnal bottleneck and the evolution of activity patterns in mammals. Proc R Soc B Biol Sci. 2013;280: 20130508.
- Reppert S, Weaver D. Coordination of circadian timing in mammals. Nature. 2002;418:935–941.10.1038/nature00965
- Ding JM, Chen D, Weber ET, et al. Resetting the biological clock: mediation of nocturnal circadian shifts by glutamate and NO. Science. 1994;266:1713–1717.10.1126/science.7527589
- Yamaguchi Y, Suzuki T, Mizoro Y, et al. Mice genetically deficient in vasopressin V1a and V1b receptors are resistant to jet lag. Science. 2013;342:85–90.10.1126/science.1238599
- Balsalobre A, Damiola F, Schibler U. A serum shock induces circadian gene expression in mammalian tissue culture cells. Cell. 1998;93:929–937.10.1016/S0092-8674(00)81199-X
- Nagoshi E, Saini C, Bauer C, et al. Circadian gene expression in individual fibroblasts. Cell. 2004;119:693–705.10.1016/j.cell.2004.11.015
- Hughes ME, DiTacchio L, Hayes KR, et al. Harmonics of circadian gene transcription in mammals. PLoS Genet. 2009;5:e1000442.10.1371/journal.pgen.1000442
- Hastings M, Reddy A, Maywood E. A clockwork web: circadian timing in brain and periphery, in health and disease. Nat Rev Neurosci. 2003;4:649–661.10.1038/nrn1177
- Yoo S, Yamazaki S, Lowrey P, et al. PERIOD2::LUCIFERASE real-time reporting of circadian dynamics reveals persistent circadian oscillations in mouse peripheral tissues. Proc Natl Acad Sci USA. 2004;101:5339–5346.10.1073/pnas.0308709101
- Yamajuku D, Inagaki T, Haruma T, et al. Real-time monitoring in three-dimensional hepatocytes reveals that insulin acts as a synchronizer for liver clock. Sci Rep. 2012;2:439.
- Tahara Y, Otsuka M, Fuse Y, et al. Refeeding after fasting elicits insulin-dependent regulation of Per2 and Rev-erbα with shifts in the liver clock. J Biol Rhythms. 2011;26:230–240.10.1177/0748730411405958
- Sato M, Murakami M, Node K, et al. The role of the endocrine system in feeding-induced tissue-specific circadian entrainment. Cell Rep. 2014;8:393–401.10.1016/j.celrep.2014.06.015
- Oishi K, Kasamatsu M, Ishida N. Gene- and tissue-specific alterations of circadian clock gene expression in streptozotocin-induced diabetic mice under restricted feeding. Biochem Biophys Res Commun. 2004;317:330–334.10.1016/j.bbrc.2004.03.055
- Davidson AJ, Stokkan KA, Yamazaki S, et al. Food-anticipatory activity and liver per1-luc activity in diabetic transgenic rats. Physiol Behav. 2002;76:21–26.10.1016/S0031-9384(02)00680-7
- Saini C, Morf J, Stratmann M, et al. Simulated body temperature rhythms reveal the phase-shifting behavior and plasticity of mammalian circadian oscillators. Genes Dev. 2012;26:567–580.10.1101/gad.183251.111
- Morf J, Rey G, Schneider K, et al. Cold-inducible RNA-binding protein modulates circadian gene expression posttranscriptionally. Science. 2012;338:379–383.10.1126/science.1217726
- Brown SA, Zumbrunn G, Fleury-Olela F, et al. Rhythms of mammalian body temperature can sustain peripheral circadian clocks. Current Biology. 2002;12:1574–1583.10.1016/S0960-9822(02)01145-4
- Buhr ED, Yoo SH, Takahashi JS. Temperature as a universal resetting cue for mammalian circadian oscillators. Science. 2010;330:379–385.10.1126/science.1195262
- Adamovich Y, Ladeuix B, Golik M, et al. Rhythmic oxygen levels reset circadian clocks through HIF1alpha. Cell Metab. 2017;25:93–101.
- Oster H, Damerow S, Kiessling S, et al. The circadian rhythm of glucocorticoids is regulated by a gating mechanism residing in the adrenal cortical clock. Cell Metab. 2006;4:163–173.10.1016/j.cmet.2006.07.002
- Balsalobre A. Resetting of circadian time in peripheral tissues by glucocorticoid signaling. Science. 2000;289:2344–2347.10.1126/science.289.5488.2344
- Tahara Y, Shiraishi T, Kikuchi Y, et al. Entrainment of the mouse circadian clock by sub-acute physical and psychological stress. Sci Rep. 2015;5:11417.10.1038/srep11417
- Landgraf D, Tsang AH, Leliavski A, et al. Oxyntomodulin regulates resetting of the liver circadian clock by food. Elife. 2015;4:e06253.
- Hirota T, Lewis WG, Liu AC, et al. A chemical biology approach reveals period shortening of the mammalian circadian clock by specific inhibition of GSK-3β. Proc Natl Acad Sci USA. 2008;105:20746–20751.10.1073/pnas.0811410106
- Quiroz JA, Gould TD, Manji HK. Molecular effects of lithium. Mol Interventions. 2004;4:259–272.10.1124/mi.4.5.6
- Martinek S, Inonog S, Manoukian AS, et al. A role for the segment polarity gene shaggy/GSK-3 in the Drosophila circadian clock. Cell. 2001;105:769–779.10.1016/S0092-8674(01)00383-X
- Hirota T, Lee JW, St John PC, et al. Identification of small molecule activators of cryptochrome. Science. 2012;337:1094–1097.10.1126/science.1223710
- Oshima T, Yamanaka I, Kumar A, et al. C-H activation generates period-shortening molecules that target cryptochrome in the mammalian circadian clock. Angew Chem Int Ed Engl. 2015;54:7193–7197.10.1002/anie.201502942
- Isojima Y, Nakajima M, Ukai H, et al. CKIε/δ-dependent phosphorylation is a temperature-insensitive, period-determining process in the mammalian circadian clock. Proc Natl Acad Sci USA. 2009;106:15744–15749.10.1073/pnas.0908733106
- Xu Y, Padiath QS, Shapiro RE, et al. Functional consequences of a CKIδ mutation causing familial advanced sleep phase syndrome. Nature. 2005;434:640–644.10.1038/nature03453
- Xu Y, Toh KL, Jones CR, et al. Modeling of a human circadian mutation yields insights into clock regulation by PER2. Cell. 2007;128:59–70.10.1016/j.cell.2006.11.043
- Kloss B, Price JL, Saez L, et al. The Drosophila clock gene double-time encodes a protein closely related to human casein kinase Iε. Cell. 1998;94:97–107.10.1016/S0092-8674(00)81225-8
- Lowrey PL, Shimomura K, Antoch MP, et al. Positional syntenic cloning and functional characterization of the mammalian circadian mutation tau. Science. 2000;288:483–491.10.1126/science.288.5465.483
- Kohsaka A, Laposky AD, Ramsey KM, et al. High-fat diet disrupts behavioral and molecular circadian rhythms in mice. Cell Metab. 2007;6:414–421.10.1016/j.cmet.2007.09.006
- Eckel-Mahan KL, Patel VR, de Mateo S, et al. Reprogramming of the circadian clock by nutritional challenge. Cell. 2013;155:1464–1478.10.1016/j.cell.2013.11.034
- Liu S, Brown JD, Stanya kJ, et al. A diurnal serum lipid integrates hepatic lipogenesis and peripheral fatty acid use. Nature. 2013;502:550–554.10.1038/nature12710
- Chen L, Yang G. PPARs integrate the mammalian clock and energy metabolism. PPAR Res. 2014;2014:653017.
- Shirai H, Oishi K, Kudo T, et al. PPARα is a potential therapeutic target of drugs to treat circadian rhythm sleep disorders. Biochem Biophys Res Commun. 2007;357:679–682.10.1016/j.bbrc.2007.04.002
- Oishi K, Shirai H, Ishida N. PPARα is involved in photoentrainment of the circadian clock. NeuroReport. 2008;19:487–489.10.1097/WNR.0b013e3282f7968f
- Liu C, Li S, Liu T, et al. Transcriptional coactivator PGC-1α integrates the mammalian clock and energy metabolism. Nature. 2007;447:477–481.10.1038/nature05767
- Yang G, Jia Z, Aoyagi T, et al. Systemic PPARγ deletion impairs circadian rhythms of behavior and metabolism. PLoS ONE. 2012;7:e38117.10.1371/journal.pone.0038117
- Oishi K, Uchida D, Ohkura N, et al. Ketogenic diet disrupts the circadian clock and increases hypofibrinolytic risk by inducing expression of plasminogen activator inhibitor-1. Arterioscler Thromb Vasc Biol. 2009;29:1571–1577.10.1161/ATVBAHA.109.190140
- Challet E, Solberg LC, Turek FW. Entrainment in calorie-restricted mice: conflicting zeitgebers and free-running conditions. Am J Physiol. 1998;274:R1751–61.
- Oike H, Nagai K, Fukushima T, et al. High-salt diet advances molecular circadian rhythms in mouse peripheral tissues. Biochem Biophys Res Commun. 2010;402:7–13.10.1016/j.bbrc.2010.09.072
- Itokawa M, Hirao A, Nagahama H, et al. Time-restricted feeding of rapidly digested starches causes stronger entrainment of the liver clock in PER2::LUCIFERASE knock-in mice. Nutr Res. 2013;33:109–119.10.1016/j.nutres.2012.12.004
- Furutani A, Ikeda Y, Itokawa M, et al. Fish oil accelerates diet-induced entrainment of the mouse peripheral clock via GPR120. PLOS ONE. 2015;10:e0132472.10.1371/journal.pone.0132472
- Fukuda T, Haraguchi A, Kuwahara M, et al. l-Ornithine affects peripheral clock gene expression in mice. Sci Rep. 2016;6:34665.10.1038/srep34665
- Oike H, Kobori M. Resveratrol regulates circadian clock genes in rat-1 fibroblast cells. Biosci Biotechnol Biochem. 2008;72:3038–3040.10.1271/bbb.80426
- Ding JM, Buchanan GF, Tischkau SA, et al. A neuronal ryanodine receptor mediates light-induced phase delays of the circadian clock. Nature. 1998;394:381–384.
- Oike H, Kobori M, Suzuki T, et al. Caffeine lengthens circadian rhythms in mice. Biochem Biophys Res Commun. 2011;410:654–658.10.1016/j.bbrc.2011.06.049
- Narishige S, Kuwahara M, Shinozaki A, et al. Effects of caffeine on circadian phase, amplitude and period evaluated in cells in vitro and peripheral organs in vivo in PER2::LUCIFERASE mice. Br J Pharmacol. 2014;171:5858–5869.10.1111/bph.2014.171.issue-24
- Burke TM, Markwald RR, McHill AW, et al. Effects of caffeine on the human circadian clock in vivo and in vitro. Sci Transl Med. 2015;7: 305ra146.
- Wu MN, Ho K, Crocker A, et al. The effects of caffeine on sleep in Drosophila require pka activity, but not the adenosine receptor. J Neurosci. 2009;29:11029–11037.10.1523/JNEUROSCI.1653-09.2009
- Feldman JF. Circadian periodicity a neurospora: alteration by inhibitors of cyclic AMP phosphodiesterase. Science. 1975;190:789–790.10.1126/science.173018
- Goodenough JE, Bruce VG. The effects of caffeine and theophylline on the phototactic rhythm of chlamydomonas reinhardii. Biological Bulletin. 1980;159:649–655.10.2307/1540830
- O’Neill JS, Maywood ES, Chesham JE, et al. cAMP-dependent signaling as a core component of the mammalian circadian pacemaker. Science. 2008;320:949–953.10.1126/science.1152506
- Onishi Y, Oishi K, Kawano Y, et al. The harmala alkaloid harmine is a modulator of circadian Bmal1 transcription. Biosci Rep. 2012;32:45–52.10.1042/BSR20110002
- Kondoh D, Yamamoto S, Tomita T, et al. Harmine lengthens circadian period of the mammalian molecular clock in the suprachiasmatic nucleus. Biol Pharm Bull. 2014;37:1422–1427.10.1248/bpb.b14-00229
- Kim SM, Neuendorff N, Chapkin RS, et al. Role of inflammatory signaling in the differential effects of saturated and poly-unsaturated fatty acids on peripheral circadian clocks. EBioMedicine. 2016;7:100–111.10.1016/j.ebiom.2016.03.037
- Oishi K, Yamamoto S, Oike H, et al. Cinnamic acid shortens the period of the circadian clock in mice. Biochemistry and Biophysics Reports. Forthcoming.
- Hirano A, Yumimoto K, Tsunematsu R, et al. FBXL21 regulates oscillation of the circadian clock through ubiquitination and stabilization of cryptochromes. Cell. 2013;152:1106–1118.10.1016/j.cell.2013.01.054
- Nakashima A, Kawamoto T, Honda KK, et al. DEC1 modulates the circadian phase of clock gene expression. Mol Cell Biol. 2008;28:4080–4092.10.1128/MCB.02168-07
- Yagita K, Okamura H. Forskolin induces circadian gene expression of rPer1, rPer2 and dbp in mammalian rat-1 fibroblasts. FEBS Lett. 2000;465:79–82.10.1016/S0014-5793(99)01724-X
- Balsalobre A, Marcacci L, Schibler U. Multiple signaling pathways elicit circadian gene expression in cultured Rat-1 fibroblasts. Curr Biol. 2000;10:1291–1294.10.1016/S0960-9822(00)00758-2
- Oike H, Oishi K, Kobori M. Nutrients, clock genes, and chrononutrition. Curr Nutr Rep. 2014;3:204–212.10.1007/s13668-014-0082-6
- Oike H, Sakurai M, Ippoushi K, et al. Time-fixed feeding prevents obesity induced by chronic advances of light/dark cycles in mouse models of jet-lag/shift work. Biochem Biophys Res Commun. 2015;465:556–561.10.1016/j.bbrc.2015.08.059
- Yasumoto Y, Hashimoto C, Nakao R, et al. Short-term feeding at the wrong time is sufficient to desynchronize peripheral clocks and induce obesity with hyperphagia, physical inactivity and metabolic disorders in mice. Metabolism. 2016;65:714–727.10.1016/j.metabol.2016.02.003
- Alexander KE, Ventura EE, Spruijt-Metz D, et al. Association of breakfast skipping with visceral fat and insulin indices in overweight latino youth. Obesity. 2009;17:1528–1533.10.1038/oby.2009.127
- Colles SL, Dixon JB, O’Brien PE. Night eating syndrome and nocturnal snacking: association with obesity, binge eating and psychological distress. Int J Obes (Lond). 2007;31:1722–1730.10.1038/sj.ijo.0803664
- Arble DM, Bass J, Laposky AD, et al. Circadian timing of food intake contributes to weight gain. Obesity. 2009;17:2100–2102.10.1038/oby.2009.264
- Arble DM, Vitaterna MH, Turek FW. Rhythmic leptin is required for weight gain from circadian desynchronized feeding in the mouse. PLoS ONE. 2011;6:e25079.10.1371/journal.pone.0025079
- van Drongelen A, Boot CR, Merkus SL, et al. The effects of shift work on body weight change – a systematic review of longitudinal studies. Scand J Work Environ Health. 2011;37:263–275.10.5271/sjweh.3143
- Hatori M, Vollmers C, Zarrinpar A, et al. Time-restricted feeding without reducing caloric intake prevents metabolic diseases in mice fed a high-fat diet. Cell Metab. 2012;15:848–860.10.1016/j.cmet.2012.04.019
- Chaix A, Zarrinpar A, Miu P, et al. Time-restricted feeding is a preventative and therapeutic intervention against diverse nutritional challenges. Cell Metab. 2014;20:991–1005.10.1016/j.cmet.2014.11.001
- He B, Nohara K, Park N, et al. The small molecule nobiletin targets the molecular oscillator to enhance circadian rhythms and protect against metabolic syndrome. Cell Metab. 2016;23:610–621.10.1016/j.cmet.2016.03.007
- Engelmann W. Slowing down of circadian-rhythms by lithium ions. Z Naturforsch C J Biosci C. 1973;28:733–736.
- Hofmann K, Gunderothpalmowski M, Wiedenmann G, et al. Further evidence for period lengthening effect of Li+ on circadian-rhythms. Z Naturforsch C J Biosci C. 1978;33:231–234.
- Li J, Lu WQ, Beesley S, et al. Lithium impacts on the amplitude and period of the molecular circadian clockwork. PLoS ONE. 2012;7:e33292.10.1371/journal.pone.0033292
- Matsuo H, Iwamoto A, Otsuka T, et al. Effects of time of L-ornithine administration on the diurnal rhythms of plasma growth hormone, melatonin, and corticosterone levels in mice. Chronobiol Int. 2015;32:225–234.10.3109/07420528.2014.965312
- Bollig I, Mayer K, Mayer WE, et al. Effects of cAMP, theophylline, imidazole, and 4-(3,4-dimethoxybenzyl)-2-imidazolidone on the leaf movement rhythm of Trifolium repens-a test of the cAMP-hypothesis of circadian rhythms. Planta. 1978;141:225–230.10.1007/BF00387893
- Bakhtiarova A, Taslimi P, Elliman SJ, et al. Resveratrol inhibits firefly luciferase. Biochem Biophys Res Commun. 2006;351:481–484.10.1016/j.bbrc.2006.10.057