Abstract
Lacquer tree sap, a raw material of traditional paints in East Asia, is hardened through laccase-catalyzed oxidation and the following polymerization of phenolic compound urushiol. In the sap’s water-insoluble fraction, we found two plantacyanins and a ferritin 2 domain-containing protein (TvFe2D, a homolog of Arabidopsis AT1G47980 and AT3G62730). The recombinant TvFe2D protein suppressed the accumulation of laccase-catalyzed oxidation products of a model substrate syringaldazine without decreasing oxygen consumption, the second substrate of laccase. The suppression was also observed when another substrate guaiacol or another oxidizing enzyme peroxidase was used. The functional domain of the suppression was the C-terminal half, downstream of the ferritin 2 domain. The results suggest that this protein may be involved in regulating the sap polymerization/hardening. We also discuss the possibility that homologous proteins of TvFe2D in other plants might be involved in the laccase- or peroxidase-mediated polymerization of phenolic compounds, such as lignin and flavonoids.
Graphical abstract
Sap exuded from the wound site of lacquer tree. The ferritin 2 domain protein in it is a novel candidate regulating urushiol polymerization/hardening.
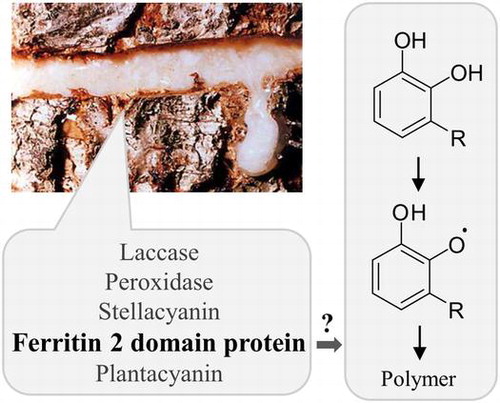
Since prehistoric times, lacquer from Toxicodendron tree sap has been used as a paint and adhesive in Japan, China and Korea. In Japan, wooden bowls and a comb coated with lacquer were found in ruins from 4,000–3,000 B.C.Citation1) In China, lacquer-coated wooden bowls from the Neolithic Age were estimated to date back 6,000 years.Citation2) Over time, lacquer painting techniques have become highly sophisticated, and today, lacquerware still fascinates people due to its high-quality gloss finish.
The tree sap, which contains phenolic monomeric compounds (urushiol in the case of Japanese lacquer tree, T. vernicifluum), is first produced in trunk epithelial cells and is extracellularly secreted into ducts. When the trunk is wounded, the sap exudes from the ducts and its monomeric compounds are oxidized to phenoxyl radicals, catalyzed by laccase (Fig. , Ref. (Citation3)), the most abundant protein in the sap. Oxygen-centered radicals can be transformed into carbon-centered allylic radicals,Citation4) resulting in the formation of a complex polymer network. The successive oxidation by laccase requires oxygen molecules that are supplied from the air because the copper (Cu+) ion in the active site of reduced laccase must be oxidized back to the Cu2+ state. In addition to phenolic compounds and laccase, other substances are also present in the sap, such as proteins and polysaccharides. Of them, stellacyanin, a Cu-containing protein found in the sap’s water-soluble fraction, reduces quinone and semiquinoneCitation5,6) and has a negative effect on the laccase reaction in vitro.Citation7) However, determining what other proteins are in the sap has not been pursued in earnest.
Fig. 1. Oxidation of urushiol by laccase. Structures of R are based on Ref. (Citation8).
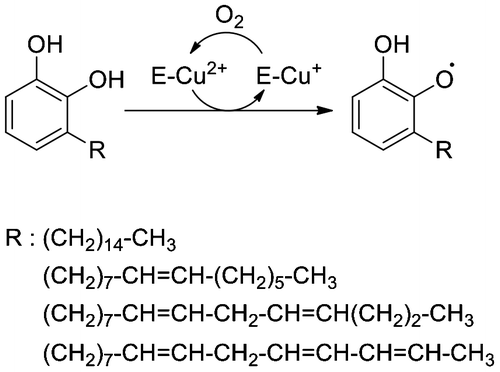
The aim of this study was to find new proteins in Toxicodendron sap and examine their roles in the oxidation/polymerization reactions. Plant laccases as well as peroxidases are primary enzymes that catalyze the deposition of lignin or flavonoid polymers.Citation9–16) The results presented here will help in understanding the mechanisms of tree sap hardening and may help identify the proteins that function with laccase or peroxidase to polymerize lignin and flavonoids in plants.
Materials and methods
Plant materials
T. vernicifluum trees were maintained at the Yakuno Tree and Lacquer Museum (Fukuchiyama, Kyoto, Japan).
Preparation of RNA and the mRNA sequencing (mRNA-Seq) analysis
Fresh young leaves were frozen in liquid nitrogen, and stored at −80 °C until use. RNA was extracted using an RNeasy Plant Mini Kit (Qiagen, Hilden, Germany). The obtained RNA was frozen in liquid nitrogen, and stored at −80 °C. For the mRNA-Seq analysis, using a GS-FLX Titanium sequencer (Roche, Basel, Switzerland), mRNA was purified twice from the RNA sample using a MicroPoly(A) Purist kit (Ambion, Austin, TX, USA). Fragmentation of mRNA by ZnCl2, reverse transcription, addition of adaptors, emulsion PCR, and pyrosequencing were performed according to the manufacturer’s manual. The sequence assembly was performed with the supplied software to generate isotigs (assembled sequences that correspond to estimated full-length mRNAs). Single reads by the GS-FLX sequencer are available through DDBJ Sequence Read Archive under accession number DRR061370. The accession numbers in DDBJ/Genbank/EBI databases are IAAX01000001-IAAX01007304 for the isotigs.
Bioinformatics
Isotigs were classified according to the gene ontology (GO) of the most similar proteins in the Arabidopsis thaliana TAIR10 database (https://www.arabidopsis.org/tools/bulk/go/index.jsp). Similarity searches against the TAIR10 database were performed using the BLASTX programCitation17) with a cut-off e-value of 1e-10. Sequences of isotigs were manipulated using software installed on Biolinux 8.Citation18) Phylogenetic analysis was performed using clustal W program.Citation19) Domain and signal peptides were identified using the SMART programCitation20) at http://smart.embl-heidelberg.de/. A co-expression analysis of Arabidopsis genes was performed using the ATTED-II databaseCitation21) at http://atted.jp/.
Preparation of lacquer proteins
T. vernicifluum sap was purchased from Satokiyomatsu-shoten (Kyoto, Japan). To remove urushiol, the tree sap (~5 g fresh weight) was suspended in a 10 × volume of acetone, and the insoluble materials were collected by centrifugation at 15,000×g for 5 min at 4 °C. The acetone-treatment and centrifugation were repeated three times. The pellet was then suspended in 10 mM K-phosphate and 1 mM ethylenediaminetetraacetic acid (EDTA), pH 6.0, and centrifuged at 15,000×g for 5 min at 4 °C. The supernatant was submitted to sodium dodecyl sulfate polyacrylamide gel electrophoresis (SDS-PAGE) or ion-exchange chromatography. The pellet was resuspended in the conventional SDS-sampling buffer for SDS-PAGE. Prior to chromatography, polyvinylpolypyrroridone, an absorbent of phenolic compounds, was added to the supernatant and then the polyvinylpolypyrroridone was removed by centrifugation at 4,000×g for 5 min. The obtained supernatant was desalted by gel filtration chromatography using a PD10 column (GE Healthcare, Piscataway, NJ, USA) pre-equilibrated with buffer B (10 mM K-phosphate and 1 mM EDTA at pH 7.0), and loaded onto a UNO Q1 anion-exchange column (Bio-Rad, Hercules, CA, USA) equilibrated with buffer B. Proteins were eluted with a linear gradient of 0–0.5 M NaCl in buffer B at a flow rate of 3.0 mL min−1. The unabsorbed protein fraction was loaded onto a UNO S1 cation-exchange column (Bio-Rad) and eluted with a linear gradient of 0–0.5 M NaCl in buffer B at a flow rate of 3.0 mL min−1.
Protein identification
After SDS-PAGE, protein bands were stained with Coomassie brilliant blue R250 and excised. Tryptic digests were prepared according toCitation22) and mass and MS/MS spectra were obtained using an Autoflex TOF/TOF mass spectrometer (Bruker Daltonics GmbH, Leipzig, German) following the protocol recommended by the manufacturer. Protein identification was performed using the Mascot program (Matrix Science, London, UK) and the de novo assembled mRNA database of T. vernicifluum. For Edman degradation, proteins were transferred to a polyvinylidene fluoride membrane after SDS-PAGE, and protein bands were submitted to Protein Sequencer PPSQ-33A (Shimadzu, Kyoto, Japan) following the protocol recommended by the manufacturer.
Reverse transcription (RT)-PCR cloning of cDNA
Total RNA extracted from T. vernicifluum leaves was reverse transcribed using ReverTra Ace reverse transcriptase (Toyobo, Osaka, Japan) and an oligo(dT) primer. The PCR was carried out using KOD-plus-Neo DNA polymerase (Toyobo) and gene-specific primers designed based on the de novo assembled RNA sequences. The obtained PCR products were inserted into a cloning vector, and the nucleotide sequences were determined. The accession numbers in DDBJ/Genbank/EBI databases are LC159300, LC159301, LC159302, and LC168644 for plantacyanin 1 and 2, the ferritin domain 2-containing protein, and peroxidase, respectively.
Preparation of recombinant proteins
The DNA fragment encoding the mature form of the TvFe2D protein was amplified by gene-specific primers and the cDNA clone as a template. The DNA for its N-terminal sequence was synthesized based on the results of the Edman degradation of the native protein. The PCR product was then inserted into the BamHI—HindIII site downstream of His- and Nus-tags in the expression vector pET-50b (Novagen, Madison, WI, USA) using an In-Fusion HD Cloning Kit (TaKaRa, Shiga, Japan). Plasmids for mutated or truncated TvFe2D proteins were produced by PCR. The fragment amplified by PCR was sequenced to confirm the valid incorporation of nucleotides.
The constructs were introduced into E. coli BL21(DE3) harboring pGro7 (TaKaRa), a plasmid for the co-expression of chaperone Gro7. The protein production was induced by the addition of 0.1 mM isopropyl-β-D-thiogalactopyranoside. The water-soluble proteins were mixed with the histidine-tag affinity resin COSMOGEL His-Accept (Nacalai Tesque, Kyoto, Japan) according to the manufacturer’s protocol. The tag-free protein was released from His- and Nus-tags by PreScission protease (GE Healthcare) according to the manufacturer’s protocol, except that the reaction buffer was changed to 50 mM Tris—Cl, 1 mM EDTA, 100 mM NaCl, 1 mM dithiothreitol, and 25 mM arginine (an additive for suppressing aggregation of the tag-free recombinant protein, as described in Ref. (Citation23)), pH 7.0. After dilution with three volume of ultrapure water, the eluted proteins were loaded onto a Q-Sepharose XL (GE Healthcare) anion-exchange column and eluted with 50 mM K-phosphate and 25 mM arginine, pH7.0. The protein solution was subjected to concentration by 3-kDa cut-off membrane (Vivaspin; Sartorius, Göttingen, Germany) and two cycles of gel filtration chromatography using PD10 columns pre-equilibrated with 100 mM K-phosphate and 25 mM arginine, pH 6.8.
To prepare His- and Nus-tagged proteins, recombinant proteins absorbed to His-Accept resin were eluted by 50 mM K-phosphate, 50 mM NaCl and 150 mM imidazole, pH 8.0, followed by concentration and gel filtration chromatography as described above, except that arginine was not contained in the buffer solution.
Enzyme assays
Laccase activity was measured spectrophotometrically essentially as described by Leonowicz and GrzywnowiczCitation24) in 1 mL of reaction mixture that contained 100 mM K-phosphate and 25 mM arginine, pH 6.8, supplemented with 5 μg mL−1 syringaldazine or 10 mM guaiacol as substrate, and Trametes laccase (M120; Amano Enzyme, Nagoya, Japan) or T. vernicifluum laccase. Accumulation of colored oxidation products,Citation25) generated non-enzymatically from phenoxyl radicals which are products of the laccases, were monitored by the increase in absorbance at 525 nm for syringaldazine (ε525 = 65 mM−1 cm−1) and 470 nm for guaiacol (ε470 = 26.6 mM−1 cm−1). The water-soluble protein fraction of T. vernicifluum sap, obtained by the aforementioned procedure, was used as T. vernicifluum laccase. Specific activity of Trametes laccase was 1.3 nmol syringaldazine-oxidized μg protein−1 min−1. Specific activities of T. vernicifluum laccase were 1.0 nmol syringaldazine-oxidized μg protein−1 min−1 and 0.2 nmol guaiacol-oxidized μg protein−1 min−1. Arginine was not included in the reaction mixture to assay recombinant TvFe2D proteins still having His- and Nus-tags.
Oxygen consumption during the laccase reaction was monitored with an oxygen electrode (YSI model 5300A, Yellow Springs Instrument Co., Yellow Springs, OH, USA) in 3 mL of reaction mixture that contained 100 mM K-phosphate and 25 mM arginine, pH 6.8, supplemented with 25 μg mL−1 syringaldazine and 10 nmol syringaldazine-oxidized mL−1 min−1 of Trametes laccase.
Peroxidase activity was measured essentially as described by Nakano and AsadaCitation26) in 1 mL of reaction mixture that contained 100 mM K-phosphate and 25 mM arginine, pH 6.8, supplemented with 5 μg mL−1 syringaldazine or 10 mM guaiacol as the reducing substrate, 0.1 mM hydrogen peroxide and horseradish peroxidase (Toyobo). Specific activities of horseradish peroxidase were 30 nmol syringaldazine-oxidized μg protein−1 min−1 and 69 nmol guaiacol-oxidized μg protein−1 min−1. Accumulation of the oxidation products were spectrophotometrically monitored as previously described.
All statistical analysis was performed using R version 3.1.2 (https://www.r-project.org/).
Results
Construction of the de novo assembled mRNA sequence database
To identify proteins included in T. vernicifluum sap, we first constructed a database of estimated mRNA sequences. Using a 454-based GS FLX titanium sequencer, 286,977 reads with an average length of 421 nucleotides and an N50 of 488 nucleotides were obtained from mRNAs isolated from young leaves of T. vernicifluum. After the de novo assembly, 7,313 estimated mRNA sequences (called “isotigs”) with an average length of 966 nucleotides and an N50 of 1,070 nucleotides were obtained. Because the sap is produced in leaves as well as in trunks of T. vernicifluum, it was expected that sap-related transcripts were included in the database. Based on sequence homology to A. thaliana proteins, the isotigs were classified according to the GO slim terms of “biological process”, “cellular component” and “molecular function” (Table S1).
Protein identification in T. vernicifluum sap
Proteins were isolated from commercially available T. vernicifluum sap. Water-soluble proteins were separated by anion-exchange chromatography, and the unabsorbed protein fraction was then separated by cation-exchange chromatography. The water-insoluble protein fraction was separated by SDS-PAGE (Fig. ). Each protein band was identified by comparing its mass and the MS/MS spectrum of its tryptic digest with the de novo assembled mRNA database (Table ). In the water-soluble fraction, the most intense protein bands at ~90 kDa (S1 and S6 in Fig. ) were laccase. Proteins in the ladder between 20 and 30 kDa (S8–S12) were all stellacyanin encoded by a single isotig, suggesting that they were modified post-translationally. Small amount of peroxidase was found at ~45 kDa (S7). In contrast to the water-soluble proteins which were reported previously,Citation27–29) proteins in the water-insoluble fraction had not been identified. We determined that this fraction contains high levels of two plantacyanins (P7 and P8) and a protein of unknown function (P1 and P2). We named the latter TvFe2D protein because it contained ferritin 2 domain (pfam13668) at its N-terminal half.
Fig. 2. SDS-PAGE of protein fractions extracted from T. vernicifluum sap. Proteins marked with numbered bands were subjected to MALDI-TOF/MS for identification. (A) Water-soluble and -insoluble fractions of the sap proteins were separated by SDS-PAGE. Protein amounts were those extracted from 9.6 and 6.9 mg of tree sap per lane for water-soluble and -insoluble fractions, respectively. (B) Water-soluble proteins were loaded onto a UNO-Q anion-exchange column and eluted with a linear gradient of 0–0.3 M KCl. The unbound proteins were loaded onto a UNO-S cation-exchange column and eluted under the same conditions.
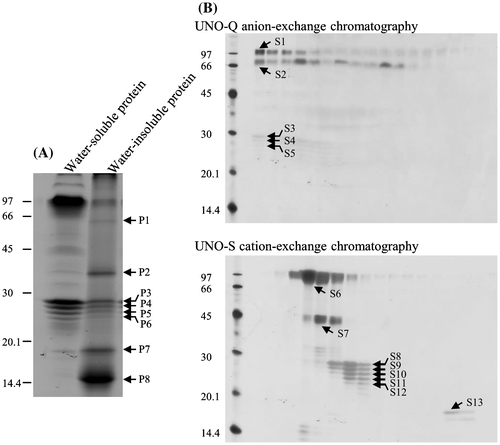
Table 1. Identification of proteins found in tree sap by MALDI-TOF/MS.
Plantacyanin is a blue copper protein and belongs to the plastocyanin-like domain (pfam02298)-containing protein family, as does stellacyanin. A phylogenetic analysis among their estimated amino acid sequences and 45 plastocyanin-like domain-containing proteins of Arabidopsis indicated that both T. vernicifluum plantacyanins were in a clade containing five Arabidopsis proteins (not shown). An alignment of their amino acid sequences is shown in Fig. . Of the five Arabidopsis proteins, plantacyanin (AT2G02850) showed the highest identity to both T. vernicifluum plantacyanins (38 and 45% for T. vernicifluum plantacyanin 1 and 2, respectively). The Arabidopsis plantacyanin is estimated to be located extracellularly or in the plasma membrane in the Arabidopsis thaliana TAIR10 database.
Fig. 3. Alignment of T. vernicifluum plantacyanins and Arabidopsis homologs. The estimated amino acid sequences of the two T. vernicifluum plantacyanin was compared with five Arabidopsis proteins that were clustered in the same clade in a phylogenetic tree constructed by the ClustalW program. N-terminal regions in Tv plantacyanin 1 and 2 (double-lined above) were predicted to be signal peptides. The plastocyanin-like domain (pfam02298) is underlined. The C-terminal extension (double-underlined) in two Arabidopsis uclacyanins were predicted to be transmembrane domain. PC, plantacyanin; ENOD22, early nodulin-like protein 22; UC, uclacyanin
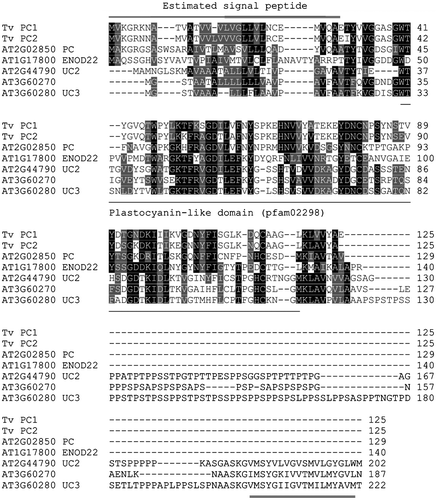
Unfortunately, the nucleotide sequence of an isotig that encodes the TvFe2D protein lacked the N-terminal region. Thus, the N-terminal amino acid sequence of the mature protein was determined by the Edman degradation of the protein separated by SDS-PAGE. The C-terminal 10 residues of the obtained sequence, APAAFGGPIIADDQDRIQVA, were overlapped with the translated 5′ terminal end sequence of the isotig, and thus, the whole amino acid sequence of the mature protein was obtained (Fig. ). There were two similar sequences in Arabidopsis, AT1G47980 (62% identity) and AT3G62730 (68% identity), both of which are estimated to be extracellular proteins in the TAIR10 database.
Effect of the TvFe2D protein on laccase- and peroxidase-mediated oxidation
To examine whether the TvFe2D protein affected the laccase reaction, we created a recombinant protein in which the mature form of TvFe2D protein was N-terminally fused with His- and Nus-tags (Fig. ). After removing the tags, the recombinant TvFe2D protein was added to a solution supplemented with commercially available fungal laccase (isolated from Trametes sp.) and the model substrate syringaldazine. Syringaldazine is oxidized by laccase to form phenoxyl radical, and is then converted non-enzymatically into the colored oxidation product, which can be monitored spectrophotometrically. Compared with a reaction mixture supplemented with bovine serum albumin (BSA) or no protein, the accumulation of the colored oxidation product was suppressed by addition of the TvFe2D protein (Fig. A). The suppression gradually diminished after a reaction time of 50 s, suggesting that some compounds or proteins were necessary for TvFe2D to retain continuous suppression activity. Based on increases in the colored oxidation product during 5–20 s after the reaction started, the accumulation rate of the colored oxidation product was calculated to decrease to 36% after the addition of the TvFe2D protein compared with the control experiment containing no protein (Fig. ). The suppression was dependent on the amount of TvFe2D protein (Fig. ). The suppression was also observed when T. vernicifluum laccase, containing small fraction of peroxidase, was used instead of the fungal laccase, suggesting that suppression occurred in T. vernicifluum sap. The TvFe2D protein also suppressed the accumulation of the colored oxidation product of guaiacol, another model compound for the laccase assay (Fig. ). In addition to laccase, suppression was also observed when horseradish peroxidase was used (Fig. )—peroxidase also oxidizes the model substrates to form phenoxyl radicals, which are then non-enzymatically converted into colored oxidation products. The suppression was barely observed when the pH of the reaction mixture was lowered from 6.8 to 6.3 (not shown), suggesting that the suppression was pH dependent.
Fig. 5. Recombinant TvFe2D proteins used in this study. (A) Fusion proteins of Nus-tag, His-tag and TvFe2D protein. In Nus-TvFe2D ΔFe, five residues conserved in the Fe-binding site of the ferritin domain were mutated. In Nus-TvFe2D Cterm, the region 180–282 downstream of the ferritin 2 domain was fused to the tag protein. (B) SDS-PAGE of the recombinant proteins purified by His-tag affinity resin. (C) SDS-PAGE of tag-free TvFe2D, purified by anion-exchange chromatography after cleaving HRV3C protease site of Nus-TvFe2D.
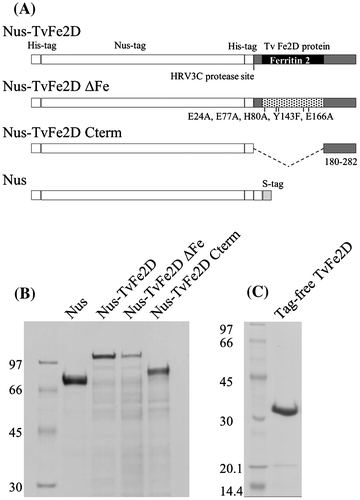
Fig. 6. Effect of the TvFe2D protein on syringaldazine oxidation by Trametes laccase. (A) Accumulation of oxidized syringaldazine monitored by spectrophotometer at 525 nm. The reaction mixture contained 5 μg mL−1 syringaldazine and 2.1 nmol syringaldazine-oxidized mL−1 min−1 in the control (thin line). BSA (47.4 μg mL−1, dotted line) or tag-free TvFe2D protein (47.4 μg mL−1, corresponding to 1.5 nmol mL−1, thick line) was included. (B) Oxygen consumption. Five times the concentration of syringaldazine, laccase, BSA and tag-free TvFe2D protein were contained compared with those in A.
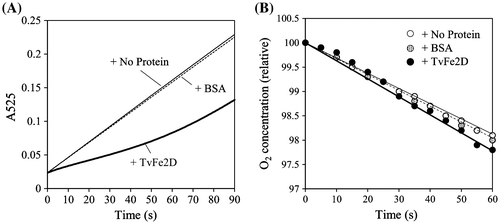
Fig. 7. Suppression of the TvFe2D protein on the accumulation of the colored oxidation products of laccases and peroxidase. Various amounts of BSA (dotted bar) or tag-free TvFe2D protein (black bar) were included in the reaction solution for the enzymatic assays of Trametes laccase, T. vernicifluum laccase or horseradish peroxidase. Syringaldazine or guaiacol was used as the substrate. The protein amount of 47.4 μg mL−1 corresponds to 1.5 nmol mL−1 of tag-free TvFe2D protein. Enzyme activities were 1.7–2.1 nmol oxidation products min−1 mL−1 in the control (white bar). The results are presented as means ± standard deviations (n = 5). Student’s t test was performed between BSA and TvFe2D samples.
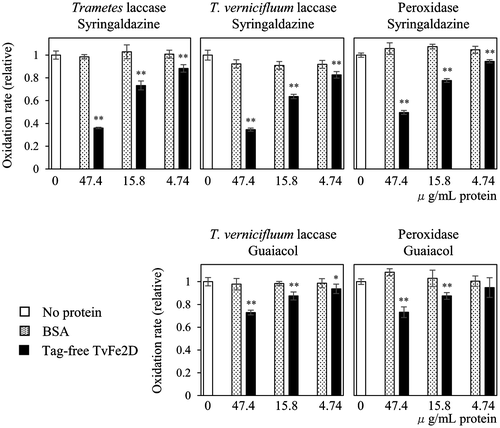
Laccase reaction consumes oxygen so that the Cu+ ion in the active site of reduced laccase is oxidized back to the Cu2+ state. Oxygen consumption was monitored during oxidation of syringaldazine by the fungal laccase in the presence or absence of TvFe2D protein (Fig. (B)). This experiment used five times the concentrations of syringaldazine, laccase, and BSA or TvFe2D protein compared with those in the spectrophotometric assay of Fig. (A), due to the lower sensitivity of the oxygen electrode. In contrast to the suppression of the accumulation of colored oxidation product, oxygen consumption was not suppressed by addition of the TvFe2D protein. Assuming the oxygen content in the air-saturated solution was equivalent to that in air-saturated water at 1 atm, with 0.253 μmol mL−1,Citation30) rates for oxygen consumption were 5.26 ± 0.19, 5.29 ± 0.52 and 6.00 ± 0.62 nmol O2 min−1 mL−1 (means ± standard deviations, n = 4), when no protein, BSA or tag-free TvFe2D protein was included, respectively. One-way analysis of variance (ANOVA) indicated no significant differences among them (p > 0.05). This result indicates that the TvFe2D protein suppressed accumulation of the colored oxidation products without inhibiting the laccase reaction.
Investigation of the functional domain in the TvFe2D protein
To investigate the functional domain responsible for the suppression of the accumulation of oxidation products, mutant TvFe2D proteins were created (Fig. ). In addition to the tag-free TvFe2D protein, a TvFe2D protein N-terminally fused with His- and Nus-tags suppressed the accumulation of the colored oxidation products of syringaldazine produced by the fungal laccase (Fig. ). The mutant TvFe2D proteins still had similar suppression activities when the ferritin 2 domain in N-terminal was deleted or when the mutation was incorporated into the five amino acid residues (E24, E77, H80, Y143, and E166) that constitute a putative iron-binding site in the ferritin 2 domain, which are conserved among the ferritin domains of various organisms.Citation31) These results indicate that the functional domain for the suppression is located in C-terminal. This region did not contain a known motif when queried against public protein motif databases, suggesting that it may contain a novel domain.
Fig. 8. Suppression of TvFe2D mutants on the accumulation of oxidized syringaldazine produced by Trametes laccase. For Nus (gray bar), Nus-TvFe2D, Nus-TvFe2DΔFe and Nus-TvFe2D Cterm (black bar), 96, 134, 134, and 105 μg, respectively, of recombinant proteins (corresponding to 1.5 nmol each) were included in 1 mL of reaction solution. The laccase activities were 1.8 nmol-oxidized syringaldazine min−1 mL−1 in the control (white bar). The results are presented as means ± standard deviations (n = 5).
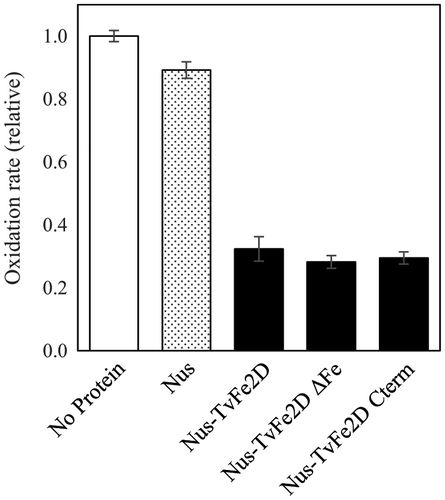
Discussion
Major proteins in the water-insoluble fraction
In this study, we found two plantacyanins (1 and 2) and a ferritin 2 domain-containing protein (TvFe2D) in the water-insoluble fraction of the tree sap, in addition to previously reported laccase, peroxidase and stellelacyanin in the water-soluble fraction. High levels of these proteins in the sap suggested that they may work in combination with laccase in urushiol oxidation/polymerization.
Possible role of plantacyanin
Dong et al.Citation32) suggested, in Arabidopsis, that plantacyanin is involved in guiding pollen tubes into styles during reproduction in flowers, but they also reported that the plantacyanin gene was expressed in various tissues, including cotyledons, hypocotyls and the vascular bundles of roots, in seedlings, and mesophyll cells, vascular bundles and guard cells in adult plants, suggesting its involvement in processes other than reproduction. Plantacyanin contains Cu ions and has a sequence similarity with stellacyanin.Citation33) The reduced form of stellacyanin can reduce quinone and semiquinone,Citation5,6) and has negative effects on laccase reactions.Citation7) The plantacyanins may thus regulate laccase reactions through their redox reaction.
Possible role of the TvFe2D protein
The TvFe2D protein suppressed laccase- and peroxidase-mediated accumulation of the colored products of two model compounds: syringaldazine and guaiacol. In the spectrophotometric enzyme assay, we monitored accumulation of the colored oxidation products, which were generated by non-enzymatic conversion of the phenoxyl radicals, produced from the model substrates by laccase or peroxidase. Currently, the mechanism of this suppression is unknown. Laccase and peroxidase produce the same phenoxyl radicals but the two enzymes are structurally unrelated and have different catalytic mechanisms. The oxidation is catalyzed by Cu ion in laccase, while in peroxidase, the substrate is oxidized by heme iron that has been pre-oxidized by hydrogen peroxide.Citation34) The fact that suppression occurred in assays of the two unrelated enzymes suggested that suppression may not be due to the direct interaction of the TvFe2D protein with the enzymes. The suppression might be due to an unknown TvFe2D activity involving the oxidation products, such as scavenging or reducing the phenoxyl radicals or their oxidation products. This idea is supported by the finding that the TvFe2D protein did not inhibit oxygen consumption during the laccase reaction.
The functional domain for the suppression was shown to be located in the C-terminal half of the TvFe2D protein, where we could find no reported domain. In addition to the N-terminal ferritin 2 domain, the C-terminal region is well conserved in homologs of Arabidopsis (Fig. ) and other plants (not shown). The function of the N-terminal ferritin 2 domain is currently unknown.
The suppression activity of TvFe2D protein suggests that the protein may be involved in urushiol polymerization and sap hardening, through regulating the accumulation of oxidized urushiol generated by laccase.
TvFe2D protein homologs in other plants
Homologs of TvFe2D-coding genes in several plant species, often called pcc13-62, were reported to be up-regulated in response to desiccation (Ref. [Citation35] and references therein). Homologous genes were also found in some bacteria. The mutational inactivation of two homologous genes in a bacterium, Deinococcus radiodurans, was reported to greatly sensitize this species to desiccation,Citation36) suggesting that the genes are related to desiccation tolerance. Zha et al.Citation37) reported that MS-desi, a protein of the bean Mucuna sempervirens (Phaseoleae) homologous to pcc13-62 and the TvFe2D protein, accumulated in the floral nectar and inhibited citrate synthase (acetyl-CoA + H2O + oxaloacetate = citrate + CoA) in vitro. This is the only report suggesting a biochemical function for the protein family. We do not currently know if there is a common mechanism among citrate synthase inhibition, desiccation tolerance, and the suppression of accumulation of oxidation products by laccase/peroxidase. The latter two might involve scavenging or reducing free radicals produced by oxidative stress under desiccation stress or by laccase/peroxidase reaction.
The TvFe2D protein’s involvement in the laccase/peroxidase reaction in the sap suggests that its homologs may be involved in oxidation/polymerization of phenolic compounds such as flavonoids and lignin monomers, which are substrates of laccase and peroxidase. In particular, laccase isoforms 4 (AT2G38080), 11 (AT5G03260), 15 (AT5G48100), and 17 (AT5G60020) are primary enzymes that catalyze lignin deposition in Arabidopsis.Citation10–13) In addition, laccase isoform 15 is involved in flavonoid deposition in the seed coat.Citation9) Peroxidases were also reported to be involved in lignin deposition.Citation14–16) A co-expression analysis using the ATTED-II database indicated that AT3G62730, encoding an Arabidopsis protein homologous to TvFe2D, was co-expressed with genes for three laccase isoforms and four peroxidase isoforms (Table ). In contrast, neither laccase nor peroxidase were found in the top 300 mutual-ranked genes that were co-expressed with AT1G47980, another TvFe2D homolog. These findings suggest that AT3G62730 may be involved in laccase/peroxidase-mediated biological processes. Two plantacyanin homologs (AT1G17800 and AT2G02850) in Arabidopsis were also co-expressed with laccase- or peroxidase-encoding genes (Table ), suggesting they may also be involved in laccase/peroxidase-mediated biological processes. To our knowledge, biological roles of laccase and peroxidase listed in Table are still obscure, except laccase isoform 15. However, laccase isoforms 5 and 12 may be also involved in lignin deposition because these two genes were shown to be co-expressed with genes for monolignol biosynthesis.Citation38)
Table 2. Arabidopsis laccase and peroxidase genes which are co-expressed with genes for plantacyann and TvFe2D protein homologs in the top 300 mutual rank.
Importance of lacquer in the future
Lacquer is an example of green chemistry. It is a 100% bio-based material that hardens through enzyme-based polymerization, in contrast to other industrial paints that are produced from fossil fuel resources and hardened by evaporating their organic solvents. Presently, lacquer’s weakness is the inability to increase its production. To understand the mechanisms behind lacquers’ polymerization and hardening would help us to understand the molecular basis of flavonoids and lignin polymerization in plants. This understanding would help us to develop environmentally friendly paint produced from flavonoid- or lignin-related compounds, which could be produced on a large scale.
Author contributions
SK performed the experiments and data analyses, and wrote the manuscript. SK, HO, and TS designed the study. TI, JI, MI, YT, NA, and YI performed the experiments. KH contributed to the protein sequencing. All of the authors contributed to manuscript revisions and approved the final version.
Disclosure statement
No potential conflict of interest was reported by the authors.
Funding
This work was partially supported by the Bunjiro Yamamoto Memorial Urushi Research Foundation of Kyoto City (S. K., Y. I., and T. S.) and the Kyoto Prefectural University fund (ACTR).
Supplemental material
Supplemental material for this article can be accessed at http://dx.doi.org/10.1080/09168451.2017.1289814
TBBB_1289814_Supplementary_File.pdf
Download PDF (13.1 KB)Acknowledgments
We wish to thank Dr Tomomi Ujihara for her aid in preparing the figures.
Notes
Abbreviation: TvFe2D, Toxicodendron vernicifluum ferritin 2 domain-containing.
References
- Kuraku Y. Origins of the use of urushi in Japan and its development. In: Brommelle NS, Smith P, editors. Urushi: Proceedings of the urushi study group. Tokyo: Getty Publications; 1988; p. 45–50.
- Zhong ZB. The protection of ancient Chinese Lacquerware. In: Brommelle NS, Smith P, editors. Urushi: Proceedings of the urushi study group. Tokyo: Getty Publications; 1988. p. 71–78.
- Oshima R, Yamauchi Y, Watanabe C, et al. Enzymic oxidative coupling of urushiol in sap of the lac tree, Rhus vernicifera. J Org Chem. 1985;50(15):2613–2621. doi:10.1021/jo00215a002
- Harigaya S, Honda T, Rong L, et al. Enzymatic dehydrogenative polymerization of urushiols in fresh exudates from the lacquer tree, Rhus vernicifera DC. J Agric Food Chem. 2007;55(6):2201–2208. doi:10.1021/jf063161g
- Holwerda RA, Knaff DB, Gray GO, et al. Reactivity of cuprous stellacyanin as a quinone and semiquinone reductase. Biochemistry. 1981;20(15):4336–4340. doi:10.1021/bi00518a016
- Knaff DB, Harsh CE, Holwerda RA. Spectroscopic studies of stellacyanin derivatives. Biochemistry. 1981;20(15):4333–4336. doi:10.1021/bi00518a015
- Wan Y-Y, Lu R, Akiyama K, Okamoto K, Honda T, Du Y-M, et al. Effects of lacquer polysaccharides, glycoproteins and isoenzymes on the activity of free and immobilised laccase from Rhus vernicifera. International Journal of Biological Macromolecules. 2010;47(1):76–81. doi:10.1016/j.ijbiomac.2010.03.016
- Yamauchi Y, Oshima R, Kumanotani J. Configuration of the olefinic bonds in the heteroolefinic side-chains of Japanese lacquer urushiol. J Chromatogr A. 1982;243(1):71–84. doi:10.1016/S0021-9673(00)88165-0
- Pourcel L. Transparent Testa10 encodes a laccase-like enzyme involved in oxidative polymerization of flavonoids in arabidopsis seed coat. The Plant Cell Online. 2005 Jan;17(11):2966–2980. doi:10.1105/tpc.105.035154
- Liang M, Davis E, Gardner D, et al. Involvement of AtLAC15 in lignin synthesis in seeds and in root elongation of Arabidopsis. Planta. 2006 Sep;224(5):1185–1196. doi:10.1007/s00425-006-0300-6
- Berthet S, Demont-Caulet N, Pollet B, et al. Disruption of LACCASE4 and 17 results in tissue-specific alterations to lignification of arabidopsis thaliana stems. The Plant Cell. 2011 Jan;23(3):1124–1137. doi:10.1105/tpc.110.082792
- Zhao Q, Nakashima J, Chen F, et al. LACCASE is necessary and nonredundant with peroxidase for lignin polymerization during vascular development in arabidopsis. The Plant Cell. 2013 Jan;25(10):3976–3987. doi:10.1105/tpc.113.117770
- Schuetz M, Benske A, Smith RA, et al. Laccases direct lignification in the discrete secondary cell wall domains of protoxylem. Plant Physiol. 2014;166(2):798–807. doi:10.1104/pp.114.245597
- Cosio C, Ranocha P, Francoz E, et al. The class III peroxidase PRX17 is a direct target of the MADS-box transcription factor AGAMOUS-LIKE15 (AGL15) and participates in lignified tissue formation. New Phytol. 2016.doi:10.1111/nph.14127
- Fernández-Pérez F, Pomar F, Pedreño MA, et al. Suppression of arabidopsis peroxidase 72 alters cell wall and phenylpropanoid metabolism. Plant Sci. 2015;239:192–199. doi:10.1016/j.plantsci.2015.08.001
- Barros J, Serk H, Granlund I, et al. The cell biology of lignification in higher plants. Ann Bot Annals Bot. 2015;115(7):1053–1074. doi:10.1093/aob/mcv046
- Jülich A. Implementations of BLAST for parallel computers. Computer applications in the biosciences: CABIOS. 1995;11(1):3–6. doi:10.1093/bioinformatics/11.1.3
- Field D, Tiwari B, Booth T, et al. Open software for biologists: from famine to feast. Nat Biotechnol. 2006;24(7):801–803. doi:10.1038/nbt0706-801
- Thompson JD, Higgins DG, Gibson TJ. CLUSTAL W: improving the sensitivity of progressive multiple sequence alignment through sequence weighting, position-specific gap penalties and weight matrix choice. Nucleic Acids Res. 1994;22(22):4673–4680. doi:10.1093/nar/22.22.4673
- Schultz J, Milpetz F, Bork P, et al. SMART, a simple modular architecture research tool: Identification of signaling domains. Proc Nat Acad Sci. 1998;95(11):5857–5864. doi:10.1073/pnas.95.11.5857
- Aoki Y, Okamura Y, Tadaka S, et al. ATTED-II in 2016: a plant coexpression database towards lineage-specific coexpression. Plant Cell Physiol. 2015 Jun;57(1):e5. Doi:10.1093/pcp/pcv165
- Jiménez CR, Huang L, Qiu Y, et al. In-gel digestion of proteins for MALDI-MS fingerprint mapping. In: Coligan JE, Dunn BM, Ploegh HL, Speicher DW, Wingfield PT, editors. Current protocols in protein science. New York (NY): Wiley; 2003. p. 16.4.1–16.4.5. doi:10.1002/0471140864.ps1604s14
- Tsumoto K, Umetsu M, Kumagai I, et al. Role of arginine in protein refolding, solubilization, and purification. Biotechnol Prog. 2004 Jan;20(5):1301–1308. doi:10.1021/bp0498793
- Leonowicz A, Grzywnowicz K. Quantitative estimation of laccase forms in some white-rot fungi using syringaldazine as a substrate. Enzyme Microb Technol. 1981;3(1):55–58. doi:10.1016/0141-0229(81)90036-3
- Hapiot P, Pinson J, Neta P, et al. Electrochemical behaviour of syringaldazine, a colorimetric redox reagent. J Electroanal Chem. 1993;353(1–2):225–235. doi:10.1016/0022-0728(93)80299-W
- Nakano Y, Asada K. Hydrogen peroxide is scavenged by ascorbate-specific peroxidase in spinach chloroplasts. Plant Cell Physiol. 1981;22(5):867–880.
- Peisach J, Levine WG, Blumberg WE. Structural properties of stellacyanin, a copper mucoprotein from Rhus vernicifera, the Japanese lac tree. J Biol Chem. 1967;242(12):2847–2858.
- Yoshida H. LXIII.—Chemistry of lacquer (Urushi). Part I. Communication from the chemical society of Tokio. J Chem Soc, Trans. 1883;43:472–486.10.1039/CT8834300472
- Suzuki S. Spectroscopic studies on lacquer peroxidase from Rhus vernicifera and its derivatives. J Inorg Biochem. 1991;44(4):267–276. doi:10.1016/0162-0134(91)84032-5
- Walker D. The use of the oxygen electrode and fluorescence probes in simple measurements of photosynthesis. 1987. Available from: http://citeseerx.ist.psu.edu/viewdoc/download?doi=10.1.1.129.2517&rep=rep1&type=pdf
- Andrews SC. The ferritin-like superfamily: evolution of the biological iron storeman from a rubrerythrin-like ancestor. Biochim Biophys Acta, Gen Subj. 2010;1800(8):691–705. doi:10.1016/j.bbagen.2010.05.010
- Dong J. Plantacyanin plays a role in reproduction in arabidopsis. Plant Physiol. 2005;138(2):778–789. doi:10.1104/pp.105.063388
- Nersissian AM, Valentine JS, Immoos C, et al. Uclacyanins, stellacyanins, and plantacyanins are distinct subfamilies of phytocyanins: plant-specific mononuclear blue copper proteins. Protein Sci. 1998;7(9):1915–1929. doi:10.1002/pro.5560070907
- Dunford HB, Dunford HB. Peroxidases and catalases: biochemistry, biophysics, biotechnology, and physiology. Hoboken, NJ: John Wiley & Sons; 2010.
- Delahaie J, Hundertmark M, Bove J, et al. LEA polypeptide profiling of recalcitrant and orthodox legume seeds reveals ABI3-regulated LEA protein abundance linked to desiccation tolerance. J Exp Bot. 2013;64(14):4559–4573. doi:10.1093/jxb/ert274
- Battista JR, Park M-J, McLemore AE. Inactivation of two homologues of proteins presumed to be involved in the desiccation tolerance of plants sensitizes deinococcus radiodurans R1 to desiccation. Cryobiology. 2001;43(2):133–139. doi:10.1006/cryo.2001.2357
- Zha H-G, Liu T, Zhou J-J, et al. MS-desi, a desiccation-related protein in the floral nectar of the evergreen velvet bean (Mucuna sempervirens Hemsl): molecular identification and characterization. Planta. 2013 Sep;238(1):77–89. doi:10.1007/s00425-013-1876-2
- Ehlting JCBC, Mattheus N, Aeschliman DS, et al. Global transcript profiling of primary stems from Arabidopsis thaliana identifies candidate genes for missing links in lignin biosynthesis and transcriptional regulators of fiber differentiation. The Plant J. 2005 Apr;42(5):618–640. doi:10.1111/j.1365-313X.2005.02403.x