Abstract
The inducible metabolites were analyzed in barley leaves inoculated with Bipolaris sorokiniana, the causal agent of spot blotch of barley. HPLC analysis revealed that B. sorokiniana-infected leaves accumulated 4 hydrophilic compounds. They were purified by ODS column chromatography and preparative HPLC. Spectroscopic analyses revealed that they were tyramine (1), 3-(2-aminoethyl)-3-hydroxyindolin-2-one (2), serotonin (3), and 5,5′-dihydroxy-2,4′-bitryptamine (4). Among these, 2 and 4 have not been reported as natural products. They showed antifungal activity in an assay of inhibition of B. sorokiniana conidia germination, suggesting that they play a role in the chemical defense of barley as phytoalexins. The accumulation of 1–4 was examined also in the leaves of rice and foxtail millet. Rice leaves accumulated 2, 3, and 4, whereas foxtail millet leaves accumulated 3 and 4 in response to pathogen attack, suggesting the generality of accumulation of 3 and 4 in the Poaceae species.
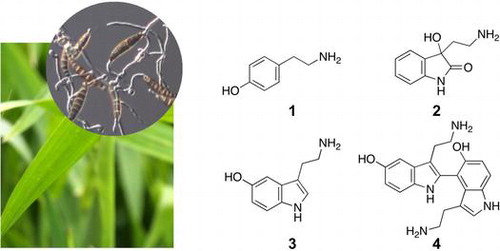
Plants respond to pathogenic infection by activating secondary metabolism, which provides for chemical and physical barrier against pathogens. This is the case for the grass family plants, which have been reported to accumulate a variety of secondary metabolites. For example, in rice (Oryza sativa), the flavonoid phytoalexin sakuranetin was implicated in the defense response to infection with rice blast fungus Magnaporthe grisea.Citation1) Rice leaves have also been shown to produce serotonin in response to pathogen attack.Citation2) The inhibition of serotonin accumulation caused by a mutation in the gene encoding tryptamine 5-hydroxylase,Citation2,3) or by the treatment of seedlings with an inhibitor of tryptophan decarboxylase,Citation4) resulted in a lack of accumulation of brown material in the cell walls and enhanced disease susceptibility. It is likely that serotonin plays a role in the reinforcement of cell walls as a precursor of the brown material.Citation2) The accumulation of serotonin has also suggested to be involved in the protection of plant tissue from reactive oxygene species generated in the interaction with M. grisea.Citation5) The accumulation of serotonin was found in other Poaceae species including, barley (Hordeum vulgare), wheat (Triticum aestivum), foxtail millet (Setaria italica), and Japanese barnyard millet (Echinochloa esculenta), but not in oats (Avena sativa), proso millet (Panicum miliaceum), maize (Zea mays), or sorghum (Sorghum bicolor).Citation6)
In barley, various inducible and constitutive secondary metabolites have been characterized in relation to defense against biological stresses. Lee et al.Citation7) reported an induced accumulation of p-coumaroylagmatine in methyl jasmonate (MeJA)-treated leaves. Similarly, Ogura et al.Citation8) found that accumulation of p-coumaroylputrescine and p-coumaroyl-3-hydroxyputrescine, as well as p-coumaroylagmatine, was induced by treatment of barley leaves with jasmonic acid (JA). This treatment also activated the formation of saponarin conjugates with ferulic and sinapic acids.Citation9) In addition, the accumulation of tryptamine was demonstrated to be elicited by infection with powdery mildew fungus Blumeria graminis f. sp. hordei,Citation10) and the accumulation of serotonin has been shown to be induced by infection with the rice brown spot fungus Bipolaris oryzae.Citation6) On the other hand, the representative defensive compounds that constitutively accumulate in barley are hordatines. Hordatines are antifungal compounds biosynthesized by dimerization of hydroxycinnamoylagmatines and accumulate at high concentrations in young seedlings.Citation11) In addition, gramine accumulates in the young seedlings of some barley cultivars and has attracted attention in view of the relationship with resistance to aphids.Citation12)
It has been demonstrated that plants often activate a multiplicity of secondary metabolic pathways upon infection with a pathogen. Rice plants accumulate diterpenoid phytoalexins and a flavonoid phytoalexin in addition to serotonin and hydroxycinnamic amides.Citation2,13,14) Maize leaves accumulate 2-hydroxy-4,7-dimethoxy-1,4-benzoxazin-3-one glucoside (HDMBOA-Glc), which is an activated form of benzoxazinone glucosidesCitation15) in addition to terpenoid phytoalexins, zealexinsCitation16), and kauralexins.Citation17) This multiplicity of defense compounds is probably important in forming a chemical barrier effective for different classes of pathogens with different sensitivity to each of the defense compounds, and to prevent the pathogens from developing tolerance to plant defense compounds. In the present study, we investigated the inducible secondary metabolites of barley to characterize the defense system with multiple defense compounds in this species.
Materials and methods
General procedures
1H- and 13C NMR spectra and 2D spectra (COSY, HMQC, and HMBC) were recorded using an Avance II instrument (Bruker, Billerica, MA, USA). High-resolution mass spectra were measured using an Exactive mass spectrometer (Thermo Fisher Scientific, Waltham, MA, USA), and ESI MS and UV-vis spectra were measured with a Quattro Micro API mass spectrometer (Waters, Milford, MA, USA) connected to an Acquity UPLC (Waters) with an ODS column (Acquity BEH C18 Column 2.1 × 50 mm (1.7 μm), Waters). Preparative HPLC was performed with a 10A HPLC system (Shimadzu, Kyoto, Japan) with an ODS column (Cosmosil 10 × 250 mm 5C18-AR-II, Nacalai Tesque, Kyoto, Japan). Circular dichroism (CD) spectra were recorded using a J-820 CD spectrophotometer (JASCO, Tokyo, Japan).
Plant materials and pathogenic fungi
Barley seeds (Hordeum vulgare cv. Asaka Gold) were purchased from JA Inaba (Tottori, Japan). The seeds were immersed in distilled water for one night, and sowed on a mixture (1:1, v/v) of vermiculite (Shoei Sangyo, Okayama, Japan) and culture soil (Iris Ohyama, Sendai, Japan), and incubated at 23 °C with 16 h/8 h light/dark cycles for 14 days. Seeds of rice (Oryza sativa cv. Nipponbare) were stock of Faculty of Agriculture, Tottori University, and seeds of foxtail millet (Setaria italica) were purchased from Tsurushin Shubyo (Matsumoto, Nagano, Japan). Bipolaris sorokiniana (OB-25-1), the causal agent of spot blotch of barley, was a stock from the Faculty of Agriculture, Tottori University. It was cultured on V8 juice agar plates for 21 days at 25 °C using a black light lamp (FL15BL-B, Hitachi, Tokyo, Japan) to obtain conidia. The conidia were suspended in distilled water containing 0.25% Tween 20, washed twice by centrifugation at 1,000×g for 10 min, and resuspended in distilled water containing 0.25% Tween 20. Droplets (5 μL) of the conidial suspension (5 × 105 conidia/mL) were then placed on barley leaf at 1.0-cm intervals. The inoculated seedlings were kept in a moist air tight bag for 24 h, brought out from the bag, and further incubated at 23 °C with 16 h/8 h light/dark cycles. B. oryzae (MAFF No. 305425), the causal agent of rice brown spot, was obtained from the National Institute of Agrobiological Sciences (NIAS) Genebank (http://www.gene.affrc.go.jp/plant/). Bipolaris maydis (HITO771), the causal agent of maize brown spot, was kindly provided by Prof. Chihiro Tanaka in Division of Environmental Science & Technology, Graduate School of Agriculture, Kyoto University. The conidia of B. oryzae and B. maydis were inoculated to the leaves of two-week-old and four-week-old seedlings of rice and foxtail millet, respectively, in a similar ways to those for B. sorokiniana.
In the experiment for the analysis of metabolites in CuCl2-treated leaves, droplets (5 μL) of 3-mM CuCl2 solution in 0.25% Tween 20 were placed on barley leaf at 1.0 cm intervals, instead of conidia suspension.
Extraction and analysis of metabolites
The leaves were excised from the seedlings after incubation, cut into small pieces using a razor blade, and immersed in 80% methanol for 24 h. The extract was analyzed by HPLC using an ODS column (Mightysil RP-18GP 150-4.6 (3 μm), Kanto Kagaku, Tokyo, Japan). HPLC conditions for quantitation of 1–4 were as follows: solvents: 0.5% trifluoroacetic acid in water (A) and acetonitrile (B); elution: 5% B/(A + B); flow rate: 0.6 mL/min, detection: 280 nm; column temperature: 40 °C.
Isolation of 1 and 2
The suspension of conidia of B. sorokiniana was applied using a brush onto third leaves (15.9 g) of barley plants. The leaves were extracted with 10 volumes of 80% methanol 48 h after inoculation. After evaporation, the extract was suspended in 0.1% trifluoroacetic acid solution and applied onto an ODS column (Cosmosil 75C18-OPN, Nacalai Tesque). The column was eluted with 0.1% trifluoroacetic acid solution. The flow-through fraction and 0.1% trifluoroacetic acid fraction were combined, evaporated, and subjected to preparative HPLC. HPLC conditions were as follows: solvents: 0.1% trifluoroacetic acid in water (A) and methanol (B); elution 8% B/(A + B); flow rate: 3.0 mL/min, detection: 280 nm; column temperature: 40 °C.
1 (0.8 mg). ESI MS:m/z 138 [M + H]+, 121 [M-NH2]+, UV-vis: λmax 273 nm. 1H NMR (600 MHz, methanol-d4) δH: 2.85 (2H, t, J = 7.9 Hz), 3.11 (2H, t, J = 7.9 Hz), 6.77 (2H, d, J = 8.5 Hz), 7.09 (2H, d, J = 8.5 Hz).
2 (1.0 mg). ESI MS:m/z 193 [M + H]+, UV-vis: λmax 252 nm, 287 nm.
Isolation of 3 and 4
The conidia of B. sorokiniana were inoculated to barley leaves (98.4 g) in the same way described for isolation of 1 and 2. After 72 h incubation, the leaves were extracted with 80% methanol. The extract was partitioned with n-hexane to remove hydrophobic substances, and evaporated. The residue was suspended in a 0.1% trifluoroacetic acid solution, and subjected to an ODS column (Cosmosil 75C18-PREP, Nacalai Tesque). The column was eluted with 0, 5, and 20% methanol in water containing 0.1% trifluoroacetic acid. Compounds 3 and 4 were eluted 20% methanol fraction. The fraction was evaporated to a small volume, and subjected to preparative HPLC. HPLC conditions were as follows: solvents: 0.1% trifluoroacetic acid in water (A) and acetonitrile (B); elution 7% B/(A + B); flow rate: 3.0 mL/min, detection: 280 nm; column temperature: 40 °C.
3 (5.8 mg). ESI MS:m/z 177 [M + H]+, 160 [M-NH2]+. UV-vis: λmax 275 nm. Citation1H NMR (600 MHz, methanol-d4) δH: 3.04 (2H, t, J = 7.3 Hz), 3.20 (2H, t, J = 7.3 Hz), 6.70 (1H, dd, J = 2.2, 8.5 Hz), 6.92 (1H, d, J = 2.2 Hz), 7.11 (1H, s), 7.20 (1H, d, J = 8.5 Hz).
4 (8.5 mg). ESI MS:m/z 351 [M + H]+. UV-vis: λmax 299 nm.
Preparation of 2 by oxidation with bisulfite in the presence of Mn2+
Preparation of 2 was performed according to Horng and Yang.Citation18) Tryptamine (160 mg, 1 mmol) was added to 200 mL 0.1 M K-Pi buffer (pH 5.6). To the solution, 10 mL each of 0.1 M MnSO4 and 0.4 M NaHSO3 was added and air was introduced to the reaction mixture. The mixture was stirred for 3 h, and evaporated to dryness. The residue was extracted by 60 mL ethanol. The ethanol extract was concentrated and dissolved in 3 mL of distilled water. The solution was subjected to preparative HPLC. The amount of 2 was 20.3 mg (yield 11%). HPLC conditions were as follows: column: Cosmosil 20 × 250 mm 5C18-AR-II; solvents: 0.5% trifluoroacetic acid in water (A) and methanol (B); elution 12% B/(A + B); flow rate: 9.0 mL/min, detection: 280 nm; column temperature: 40 °C. 1H- and 13C NMR data of synthesized compound was identical with those of 2 purified from barley leaves.
Enzymatic preparation of 4
Peroxidase reaction was performed in a mixture consisted of 0.4 mM H2O2, 2 mM serotonin, 0.2 μg/mL peroxidase (from horseradish, Wako Pure Chemical Industry, Osaka, Japan) in 5 mM Na-Pi buffer (pH 3.5) with a final volume of 1 L. The reaction time was 30 min, and reaction was stopped by immersing the mixture in a water bath at 100 °C. After adding 1 mL of trifluoroacetic acid, the mixture was applied to an ODS column (Cosmosil 75C18-OPN) equilibrated with 0.1% trifluoroacetic acid. The column was eluted with 0, 5, 10, and 20% methanol containing 0.1% trifluoroacetic acid. Compound 4 was eluted in the 5% methanol fraction. The fraction was evaporated to a small volume and subjected to preparative HPLC. The HPLC conditions were the same as those for the purification of 4 from barley leaves. From the HPLC, we purified two peaks. The first peak was the main peroxidase reaction product (5). The second peak showed the same retention time with 4. The fractions corresponding to these peaks were collected. The analytical HPLC of second peak fraction revealed that the fraction contained 4 and an additional product 6. Thus, the fraction was subjected to a second preparative HPLC, which resulted in the isolation of 4 and 6. The solvents were changed to 0.5% trifluoroacetic acid in water (A) and methanol (B) for the second preparative HPLC, and the isocratic elution with 5% B/(A + B) was applied to separate 4 and 6.
4 (3.0 mg). ESI MS, 1H NMR, and 13C NMR spectra were identical with those of 4 purified from barley leaves.
5 (23.1 mg). ESI MS: m/z 351 ([M + H]+). 1H NMR (600 MHz, methanol-d4) δH: 2.16 (1H, m), 2.36 (1H, m), 2.43 (2H, m), 6.90 (1H, d, J = 8.7 Hz), 7.07 (1H, s), 7.35 (1H, d, J = 8.7 Hz). 13C NMR (150 MHz, methanol-d4) δC: 25.2, 40.8, 110.6, 113.1, 113.2, 113.7, 113.9, 126.8, 128.4, 149.1.
6 (14.1 mg). ESI MS: m/z 525 ([M + H]+). 1H NMR (600 MHz, methanol-d4) δH 2.35 (2H, m), 2.45 (4H, m), 2.55 (1H, m), 2.65 (2H, m), 2.71 (1H, m), 2.75 (1H, m), 2.88 (1H, m), 6.90 (1H, d, J = 8.7 Hz), 6.95 (1H, d, J = 8.6 Hz), 7.11 (1H, s), 7.13 (1H, s), 7.14 (1H, s), 7.29 (1H, d, J = 8.6 Hz), 7.36 (1H, s), 7.38 (1H, d, J = 8.7 Hz). 13C NMR(150 MHz, methanol-d4)δC: 25.15, 25.20, 25.6, 41.1, 42.17, 42.23, 110.9, 111.0, 112.7, 112.9, 113.1, 113.4, 113.4, 115.0, 115.6, 116.6, 122.0, 126.5, 126.9, 127.1, 127.5, 127.9, 128.3, 133.5, 133.6, 134.0, 146.7, 148.5, 149.3. 1H NMR (600 MHz, D2O) δH: 2.37 (2H, m), 2.43 (1H, m), 2.46 (2H, m), 2.59 (2H, m), 2.64 (1H, m), 2.70 (4H, m) 6.990 (1H, d, J = 8.6 Hz),6.991 (1H, d, J = 8.7 Hz), 7.245 (2H, s), 7.251 (1H, s), 7.44 (1H, s), 7.45 (1H, d, J = 8.6 Hz), 7.50 (1H, d, J = 8.7 Hz). 13C NMR (150 MHz, D2O) δC: 24.1, 24.3, 24.4, 40.6, 41.2, 110.2, 110.3, 110.4, 112.9, 113.0, 113.1, 113.5, 113.8, 114.3, 115.0, 120.8, 126.7, 126.9, 127.4, 127.5, 132.6, 132.9, 133.1, 146.0, 147.7, 148.5.
Assay for inhibition of germination of B. sorokiniana conidia
Compounds 1–4 were dissolved in 10 mM Na-Pi buffer (pH 6.0). The 12.5 μL droplets of solutions were placed on a slide glass, and mixed with 12.5 μL of suspension of B. sorokiniana conidia. The slide glass was placed in a Petri dish with a layer of wet filter paper. The Petri dish was covered by an air-tight bag to maintain high humidity, and incubated at 28 °C for 4 h. The numbers of germinated conidia were counted using a microscope (Olympus BX43, Tokyo, Japan).
Results
Identification of compounds induced by infection of B. sorokiniana in barley leaves
The conidia of B. sorokiniana were inoculated to the third leaves of 14-day-old barley seedlings. The leaves were extracted with 80% methanol 48 h after inoculation, and the changes in metabolites were analyzed by HPLC. As shown in Fig. , we detected the accumulation of four compounds 1–4 in the leaves inoculated with B. sorokiniana.
Fig. 1. Chromatograms of extracts of Bipolaris sorokiniana-infected, control, and intact leaves. The suspension of conidia of B. sorokiniana (inoculation) or distilled water containing 0.25% Tween 20 (control) was placed on third leaves of two-week-old barley seedlings. The leaves were extracted 48 h after inoculation and subjected to high-performance liquid chromatography (HPLC) analysis. Leaves without any treatment (intact) was also extracted and analyzed by HPLC.
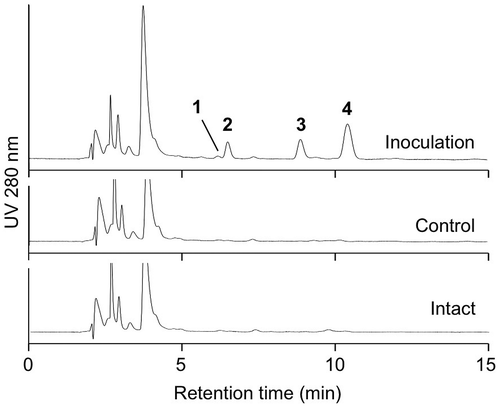
Compounds 1 and 2 were purified from 15.9 g of barley leaves inoculated with B. sorokiniana. Molecular weight of 1 was determined to be 137 by electrospray ionization–mass spectrometry (ESI–MS), suggesting the presence of a nitrogen atom. Two doublet signals (δH 7.09, d, J = 8.5 Hz, 2H, and δH 6.77, d, J = 8.5 Hz, 2H) and two triplet signals (δH 3.11, t, J = 7.9 Hz, 2H, and δH 2.85, t, J = 7.9 Hz, 2H) on 1H NMR spectrum indicated the presence of a p-substituted benzene ring and an ethylene group, respectively. These data suggested that 1 was tyramine (Fig. ). Because the retention time of 1 on HPLC as well as UV and 1H NMR spectra was identical to those of the authentic compound, we concluded that 1 was tyramine.
Compound 2 had the molecular formula C10H12N2O2 as revealed by high-resolution ESI MS (m/z 193.0981, [M + H]+). Four signals of aromatic protons (δH 7.07, 7.22, 7.42, and 7.48) on 1H NMR spectrum indicated the presence of a 1,2-disubstituted benzene ring (Table ). The chemical shift (δC 141.0) of an aromatic carbon suggested the linkage of nitrogen atom to the carbon. Two multiplet signals (δH 3.08 and 2.29) that were correlated with each other in a HH COSY spectrum suggested the presence of an ethylene group. A linkage of the ethylene group to a nitrogen atom was also suggested by the chemical shift (δH 3.08) of one of the ethylene proton signals. The two 13C NMR signals (δC 181.3 and 76.0) were assigned to a carbonyl carbon and a quaternary carbon bearing an oxygen atom, respectively, because of their chemical shifts. HMBC spectrum showed the correlations between three proton signals (δc 2.29, 3.08, and 7.48) and the quaternary carbon (δC 76.0) and between the proton signal (δH 2.29) and the carbonyl carbon (δC 181.3) as shown in Fig. . On the basis of these data, we constructed two possible structures 2′ and 2″ in Fig. . We performed database search and found two compounds A and B that have similar structures to 2′ and 2″, respectively.Citation19) As shown in Table , the chemical shifts of Citation13C NMR signals of 2 except for those corresponding to the ethylene group were very close to those of the indolin-2-one structure in compound A, but were somewhat different from those of aromatic, carbonyl, and quaternary carbons in B. Thus, we concluded that 2 was 3-(2-aminoethyl)-3-hydroxyindolin-2-one. This is the first isolation of 2 as free form from natural sources. We measured CD spectra of 2 in methanol at various concentrations, but did not detect significant peaks in the range between 200 and 500 nm, suggesting that 2 was a racemic mixture.
Table 1. 1H- (600 MHz) and 13C-NMR (150 MHz) spectroscopic data of compound 2 in D2O.
Fig. 3. HMBC (indicated by arrows from 1H to 13C) and COSY (indicated by bold lines) for 2, and two possible structures 2′ and 2”. The chemical structure of 2 was determined by the comparison of chemical shifts of 13C-NMR signals with those of compounds A and B (Chen et al. (2010) Tetrahedron 66: 1441.19).
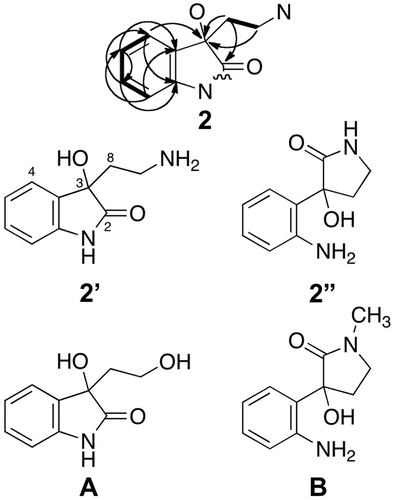
Table 2. Comparison of 13C-NMR (150 MHz) chemical shifts of 2 with those of compounds A ((R)-3-hydroxy-3-(2-hydroxyethyl)indolin-2-one) and B ((-)-donaxaridine) in DMSO-d6.
To confirm the identity of the compound, we prepared 2 by oxidation of tryptamine with bisulfite in the presence of Mn2+ in accordance with the method described by Horng and Yang.Citation18) The synthesized compound showed the 1H- and 13C NMR spectra identical to 2 isolated from barley leaves.
Compounds 3 and 4 were purified from 98.4 g of barley leaves inoculated with conidia of B. sorokiniana. ESI MS indicated that the molecular weight of 3 was 176, suggesting that 3 was serotonin. Because the retention time on HPLC as well as UV and 1H NMR spectra of 3 was identical to those of the authentic compound, we concluded that 3 was serotonin (Fig. ). The induction of serotonin in barley was reported previously.Citation6)
Compound 4 had the molecular formula C20H22N4O2 as revealed by high-resolution ESI MS (m/z 351.1813, [M + H]+). On the basis of the molecular formula, we assumed that 4 was a dehydrodimer of serotonin. As shown in Table , six signals in 1H NMR and 16 signals in 13C NMR were detected in the aromatic regions, suggesting the presence of two indole rings. The lack of a singlet signal and a doublet signal with a small coupling constant in 1H NMR of serotonin suggested the linkage of two indole rings between the 2 and 4 positions. Thus, 4 was considered to be 5,5′-dihydroxy-2,4′-bitryptamine, the dehydrodimer of serotonin connected between the 2 and 4 positions. Supplementary Fig. shows the HMBC and HH COSY correlations, which were consistent with the proposed structure. 4 has not been reported as a natural product.
Table 3. 1H- (600 MHz) and 13C-NMR (150 MHz) spectroscopic data of compound 4 in methanol-d4.
The formation of 4 from serotonin was reported by electrochemical oxidationCitation20) and by peroxidase reaction.Citation21) Thus, we carried out a peroxidase reaction with serotonin as the substrate. We purified two dehydrodimers C and D and a dehydrotrimer E of serotonin from the reaction mixture by HPLC. The 1H- and 13C NMR spectra of D were identical to those of 4, confirming that 4 was a dehydrodimer of serotonin. Dehydrodimer C and dehydrotrimer E were identified as 5,5′-dihydroxy-4,4′-bitryptamine (5) and 5,5′,5″-trihydroxy-4,4′:6,4″-tertryptamine (6), respectively, on the basis of 1H- and 13C NMR spectra (Supplementary Fig. ). It is interesting that 5 was detected also in the leaves of rice that accumulate serotonin at a high concentration because of overexpression of tryptophan decarboxylase genes.Citation22)
Determination of 1–4 levels in B. sorokiniana-infected and CuCl2-treated leaves and their antifungal activities
The kinetics of the accumulation of 1–4 were investigated in B. sorokiniana-infected leaves (Fig. ). The amounts of 1 reached a maximum 24 h after inoculation. The increase of 1 was detected also in the control and intact leaves, probably because of the aging of the leaves. Compound 2 started to increase 24 h after inoculation, and continued to increase 96 h after inoculation. Kinetics of accumulation of 3 and 4 were similar of each other; 3 and 4 started to increase 24 h after inoculation, reached maximum after 48 h, and then gradually decreased.
Fig. 4. Kinetics of accumulation of inducible compounds, 1 (A), 2 (B), 3 (C), and 4 (D). Conidia of Bipolaris sorokiniana were inoculated onto third leaves of two-week-old barley seedlings (black bars). As the control, distilled water containing 0.25% Tween 20 was placed on the leaves (gray bars). The contents of metabolites without any treatment were also analyzed (intact bars). At time zero, only intact leaves were analyzed. The error bars indicate the standard deviations obtained from at least three replicates.
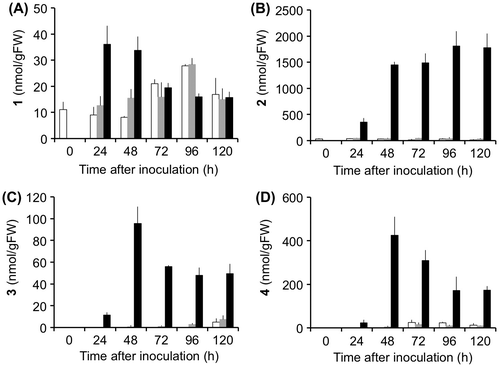
To verify that barley leaves produce 1–4 without inoculation of B. sorokiniana, we treated the third leaves with 3-mM CuCl2 for 72 h, and analyzed accumulation of 1–4. The amount of 1 was 16.0 nmol/gFW, and other compounds were not detected in control leaves. In the leaves treated with CuCl2, the amounts of 1–4 increased to 38.8, 3320, 97.6, and 31.7 nmol/gFW, respectively.
Among the amines that accumulated in the B. sorokiniana-infected leaves, 4 showed the strongest inhibition activity for germination of conidia of B. sorokiniana, followed by 2, 1, and 3 in this order (Fig. ). The inhibition rates by treating 1–4 at 300 μg/mL were 14.1, 53.5, 16.5, and 86.7%, respectively.
Induced accumulation of aromatic amines in rice and foxtail millet in response to pathogen infection
To examine the generality of the accumulation of these aromatic amines in the Poaceae, we inoculated conidia of B. oryzae and B. maydis to the leaves of rice and foxtail millet, respectively. These species have been shown to accumulate 3.Citation2,6) We extracted the leaves 60 h after inoculation, and analyzed the amounts of 1–4 by HPLC (Table ). The leaves of rice and foxtail millet accumulated 3 and 4 in response to pathogen infection in common. Rice leaves also showed induced accumulation of 2 but not 1. The B. maydis-infected leaves of foxtail millet did not accumulate either 1 or 2.
Table 4. Accumulation of aromatic amines in rice and foxtail millet in response to pathogen infection.
Discussion
In the present study, we found that barley leaves accumulated tyramine (1), 3-(2-aminoethyl)-3-hydroxyindolin-2-one (2), serotonin (3), and 5,5′-dihydroxy-2,4′-bitryptamine (4) in response to attack by B. sorokiniana. In barley, metabolic changes in response to JACitation7–9) and pathogen infection have been reported.Citation6,10,23) The inducible metabolites previously identified were p-coumaroylagmatine and p-coumaroylhydroxyagmatine, tryptamine, serotonin, and hydroxycinnamoylsaponarins. The present findings of new inducible metabolites indicate that diverse metabolic pathways are activated in the defense responses in barley, as in other Poaceae species.Citation13,24)
Tyramine (1) is present in various plants including vegetables,Citation25) and serves as precursor for secondary metabolites such as benzylisoquinoline alkaloids. The seedling of barley also contains the simple amine hordenine derived from tyramine.Citation26) The hydroxycinnamic acid conjugates of tyramine were found to accumulate as a part of defense responses in various plants, and they are indicated to be incorporated into cell walls.Citation27–29) Tyramine may also act as the precursor for defensive hydroxycinnamic acid amides in barley. The enzyme activity that catalyzes the formation of hydroxycinnamic acid amides of tyramine was detected in roots of barley and wheat.Citation30) Furthermore, we detected the accumulation of serotonin. The inducible accumulation of serotonin has also been reported in rice.Citation2) Serotonin has been shown to be incorporated into cell walls to form brown deposits. The induction of hydroxycinnamic acid amides of serotonin has been demonstrated in the pathogen-infected leaves of rice.Citation2) Tyramine as well as serotonin may function in the defense response as free forms or as hydroxycinnamic acid amides in the reinforcement of cell walls.
This study is the first report that shows the presence of 3-(2-aminoethyl)-3-hydroxyindolin-2-one (2) as a free form. Its hydroxycinnamic acid amides have been isolated from Cinnamomum triplinervis.Citation31) We detected the antifungal activity of 2 in a germination inhibition assay for B. sorokiniana. Similarly, we also detected antifungal activity for 4. Compound 4 has been demonstrated to be formed by electrochemical oxidationCitation21) and by the peroxidase reaction,Citation20) but has not been reported as a natural product. On the basis of their antifungal activity, accumulation of these metabolites is considered to function as phytoalexins as a part of the chemical barrier against pathogens.
Compound 2 is deduced to be biosynthesized by oxidation of tryptamine at positions 2 and 3. Oxidation of the indole ring is part of the biosynthetic pathway for benzoxazinones in wheat, rye, and maize. In the biosynthesis of benzoxazinones, the oxidation reactions were catalyzed by specific cytochrome P450 enzymes classified into the CYP71C group.Citation32,33) The hydroxylation of tryptamine to serotonin was also catalyzed by the cytochrome P450 enzyme CYP71P1 in rice.Citation3) The oxidation of tryptamine to 2 maybe catalyzed by similar cytochrome P450 enzymes. In barley, the genes encoding CYP71C group enzymes responsible for the biosynthesis of benzoxazinones were absent, but some cytochrome P450 genes in this family may be involved in the generation of 2. In addition, 2,3-dioxy-indole-3-acetic acid (DiOxIAA), which is a metabolite of indole-3-acetic acid (IAA), has the same oxidized indole ring system.Citation34,35) The enzyme activity that catalyzes the conversion of IAA to DiOxIAA was detected in a crude extract of rice husk.Citation36) The rice sl mutant has been shown to accumulate a high concentration of DiOxIAA and its asparagine conjugate together with IAA when the leaves were infected with B. oryzae.Citation37) It is of interest to explore the relationship between the enzymes involved in the biosynthesis of 2 and DiOxIAA.
The antifungal activity of serotonin (3) at high concentrations was reported.Citation38) We found that the activity was enhanced by dimerization to 4. Although oligomers of serotonin are easily prepared using a peroxidase reaction, their biological activities have not been investigated. We found that the dimer of serotonin (4) showed 86.7% inhibition of spore germination at 300 μg/mL. The activity of serotonin itself was weaker than the dimer, with the inhibition rate being 16.5% at the same concentration. Thus, the dimerization is the mechanism that increases biological activity in this case. Similar examples are seen with the generation of ε-viniferin from resveratrol. ε-Viniferin has been characterized as a phytoalexin in grape.Citation39) Resveratrol did not inhibit fungal pathogens, whereas ε-veniferin has been shown to be effective to inhibit the growth of pathogens in concentration range of 12–100 μg/mL.Citation39) Similarly, the precursor p-coumaroylagmatine showed no antifungal activity at concentrations lower than 80 ppm, but its dimer hordatine A exerted activity at 5 ppm, inhibiting spore germination by 80%.Citation40) Furthermore, we found the generation of various dehydrodimers of avenanthramide phytoalexins in oats.Citation41,42) Thus, dimerization and oligomerization are considered as simple but effective mechanisms that increase the diversity of secondary metabolites and potentially enhance their biological activity.
Compounds 2–4 are all biosynthesized via the tryptophan biosynthetic pathway. In the grass family, the tryptophan pathway has been implicated in defense responses in various ways. For example, serotonin accumulation in response to pathogen attack has been found in barley, rice, wheat, foxtail millet, and Japanese barnyard millet.Citation6) In the present study, we also found that 4 was present in barley, rice, and foxtail millet. The grass family was classified into BOP and PACMAD clades by a large-scale phylogenic analysis.Citation43,44) Rice, barley, and wheat are classified into the BOP clade, whereas foxtail millet and Japanese barnyard millet are into the PACMAD clade. Thus, the induced accumulation of serotonin and its dehydrodimer 4 was likely acquired by an early ancestor of grass family species. Benzoxazinones also occur in species in both clades—wheat and rye in BOP clade and maize in PACMAD clade.Citation45–47) Because serotonin and benzoxazinones are derived from the tryptophan biosynthetic pathway, both the inducible and constitutive defense systems of the grass family largely depend on this pathway.
Supplemental materials
The supplemental material for this paper is available at http://dx.doi.org/10.1080/09168451.2017.1290520
Notes on contributors
Rie Kumeda, Noriko Hayashi, Yukari Yagi, Nanase Sakaguchi, Yu Kokubo, Naoki Ube, and Shin-ichi Tebayashi (S. T.) performed experiments. Kotomi Ueno and Atsushi Ishihara (A. I.) analyzed data, and A. I. wrote the paper.
Funding
This work was supported by JSPS KAKENHI [grant number 16K07718] for A. I. and [16K15069] for S. T.
Disclosure statement
No potential conflict of interest was reported by the authors.
TBBB_1290520_Supplementary_materials.pdf
Download PDF (239.1 KB)References
- Kodama O, Miyakawa J, Akatsuka T, et al. Sakuranetin, a flavanone phytoalexin from ultraviolet-irradiated rice leaves. Phytochemistry. 1992;31:3807–3809.10.1016/S0031-9422(00)97532-0
- Ishihara A, Hashimoto Y, Tanaka C, et al. The tryptophan pathway is involved in the defense responses of rice against pathogenic infection via serotonin production. Plant J. 2008;54:481–495.10.1111/j.1365-313X.2008.03441.x
- Fujiwara T, Maisonneuve S, Isshiki M, et al. Sekiguchi lesion gene encodes a cytochrome P450 monooxygenase that catalyzes conversion of tryptamine to serotonin in rice. J Biol Chem. 2010;285:11308–11313.10.1074/jbc.M109.091371
- Ishihara A, Nakao T, Mashimo Y, et al. Probing the role of tryptophan-derived secondary metabolism in defense responses against Bipolaris oryzae infection in rice leaves by a suicide substrate of tryptophan decarboxylase. Phytochemistry. 2011;72:7–13.10.1016/j.phytochem.2010.11.001
- Hayashi K, Fujita Y, Ashizawa T, et al. Serotonin attenuates biotic stress and leads to lesion browning caused by a hypersensitive response to Magnaporthe oryzae penetration in rice. Plant J. 2016;85:46–56.10.1111/tpj.13083
- Ishihara A, Matsukawa T, Nomura T, et al. Involvement of tryptophan-pathway-derived secondary metabolism in the defence responses of grasses. In: Amino acids in higher plants; 2016. p. 362–389.
- Lee J, Vogt T, Schmidt J, et al. Methyljasmonate-induced accumulation of coumaroyl conjugates in barley leaf segments. Phytochemistry. 1997;44:589–592.10.1016/S0031-9422(96)00562-6
- Ogura Y, Ishihara A, Iwamura H. Induction of hydroxycinnamic acid amides and tryptophan by jasmonic acid, abscisic acid and osmotic stress in barley leaves. Z Naturforsch C. 2001;56:193–202.
- Ishihara A, Ogura Y, Tebayashi S, et al. Jasmonate-induced changes in flavonoid metabolism in barley (Hordeum vulgare) leaves. Biosci Biotechnol Biochem. 2002;66:2176–2182.10.1271/bbb.66.2176
- Miyagawa H, Toda H, Tsurushima T, et al. Accumulation of tryptamine in barley leaves irradiated with UV light. Biosci Biotechnol Biochem. 1994;58:1723–1724.10.1271/bbb.58.1723
- Stoessl A, Unwin CH. The antifungal factors in barley. V. Antifungal activity of the hordatines. Can J Bot. 1970;48:465–470.10.1139/b70-066
- Zúñiga GE, Corcuera LJ. Effect of gramine in the resistance of barley seedlings to the aphid Rhopalosiphum padi. Entomol Exp Appl. 1986;40:259–262.
- Yamane H. Biosynthesis of phytoalexins and regulatory mechanisms of it in rice. Biosci Biotechnol Biochem. 2013;77:1141–1148.10.1271/bbb.130109
- Park HL, Lee SW, Jung KH, et al. Transcriptomic analysis of UV-treated rice leaves reveals UV-induced phytoalexin biosynthetic pathways and their regulatory networks in rice. Phytochemistry. 2013;96:57–71.10.1016/j.phytochem.2013.08.012
- Oikawa A, Ishihara A, Tanaka C, et al. Accumulation of HDMBOA-Glc is induced by biotic stresses prior to the release of MBOA in maize leaves. Phytochemistry. 2004;65:2995–3001.10.1016/j.phytochem.2004.09.006
- Schmelz EA, Kaplan F, Huffaker A, et al. Identity, regulation, and activity of inducible diterpenoid phytoalexins in maize. Proc Nat Acad Sci USA. 2011;108:5455–5460.10.1073/pnas.1014714108
- Huffaker A, Kaplan F, Vaughan MM, et al. Novel acidic sesquiterpenoids constitute a dominant class of pathogen-induced phytoalexins in maize. Plant Physiol. 2011;156:2082–2097.10.1104/pp.111.179457
- Horng AJ, Yang SF. Aerobic oxidation of indole-3-acetic acid with bisulfite. Phytochemistry. 1975;14:1425–1428.10.1016/S0031-9422(00)98643-6
- Chen WB, Du XL, Cun LF, et al. Highly enantioselective aldol reaction of acetaldehyde and isatins only with 4-hydroxydiarylprolinol as catalyst: concise stereoselective synthesis of (R)-convolutamydines B and E, (-)-donaxaridine and (R)-chimonamidine. Tetrahedron. 2010;66:1441–1446.10.1016/j.tet.2009.12.041
- Wrona MZ, Dryhurst G. Interactions of 5-hydroxytryptamine with oxidative enzymes. Biochem Pharmacol. 1991;41:1145–1162.10.1016/0006-2952(91)90653-M
- Wrona MZ, Dryhurst G. Electrochemical oxidation of 5-hydroxytryptamine in acidic aqueous solution. J Org Chem. 1989;54:2718–2721.10.1021/jo00272a048
- Kanjanaphachoat P, Wei BY, Lo SF, et al. Serotonin accumulation in transgenic rice by over-expressing tryptophan decarboxylase results in a dark brown phenotype and stunted growth. Plant Mol Biol. 2012;78:525–543.10.1007/s11103-012-9882-5
- von Röpenack E, Parr A, Schulze-Lefert P. Structural analyses and dynamics of soluble and cell wall-bound phenolics in a broad spectrum resistance to the powdery mildew fungus in barley. J Biol Chem. 1998;273:9013–9022.10.1074/jbc.273.15.9013
- Ejike CECC, Gong M, Udenigwe CC. Phytoalexins from the Poaceae: biosynthesis, function and prospects in food preservation. Food Res Int. 2013;52:167–177.10.1016/j.foodres.2013.03.012
- Ly D, Kang K, Choi JY, et al. HPLC analysis of serotonin, tryptamine, tyramine, and the hydroxycinnamic acid amides of serotonin and tyramine in food vegetables. J Med Food. 2008;11:385–389.10.1089/jmf.2007.514
- Leete E, Marion L. The biogenesis of alkaloids: vii. the formation of hordenine and n-methyltyramine from tyrosine in barley. Can J Chem. 1953;31:126–128.10.1139/v53-017
- Facchini PJ, Huber-Allanach KL, Tari LW. Plant aromatic L-amino acid decarboxylases: evolution, biochemistry, regulation, and metabolic engineering applications. Phytochemistry. 2000;54:121–138.10.1016/S0031-9422(00)00050-9
- Ishihara A, Kawata N, Matsukawa T, et al. Induction of N -hydroxycinnamoyltyramine synthesis and tyramine N -hydroxycinnamoyltransferase (THT) activity by wounding in maize leaves. Biosci Biotechnol Biochem. 2000;64:1025–1031.10.1271/bbb.64.1025
- Negrel J, Jeandet P. Metabolism of tyramine and feruloyltyramine in TMV inoculated leaves of Nicotiana tabacum. Phytochemistry. 1987;26:2185–2190.10.1016/S0031-9422(00)84681-6
- Louis V, Negrel J. Tyramine hydroxycinnamoyl transferase in the roots of wheat and barley seedlings. Phytochemistry. 1991;30:2519–2522.10.1016/0031-9422(91)85092-E
- Ripperger H, Diaz M, Schreiber K. Two cinnamoyl derivatives from Cinnamomum triplinervis. Phytochemistry. 1981;20:1453–1454.10.1016/0031-9422(81)80070-2
- Frey M, Chomet P, Glawischnig E, et al. Analysis of a chemical plant defense mechanism in grasses. Science. 1997;277:696–699.10.1126/science.277.5326.696
- Nomura T, Ishihara A, Imaishi H, et al. Molecular characterization and chromosomal localization of cytochrome P450 genes involved in the biosynthesis of cyclic hydroxamic acids in hexaploid wheat. Mol Genet Genom. 2002;267:210–217.
- Chamarro J, Östin A, Sandberg G. Metabolism of indole-3-acetic acid by orange (Citrus sinensis) flavedo tissue during fruit development. Phytochemistry. 2001;57:179–187.10.1016/S0031-9422(01)00023-1
- Tsurumi S, Wada S. Metabolism of indole-3-acetic acid and natural occurrence of dioxindole-3-acetic acid derivatives in Vicia roots. Plant Cell Physiol. 1980;21:1515–1525.10.1093/pcp/21.8.1515
- Kato T, Tomita N, Hoshikawa M, et al. In vitro oxidation of indoleacetic acid by crude enzyme from rice husk—an aspect of preharvest sprouting. Heterocycles. 1998;47:497–500.10.3987/COM-97-S(N)12
- Ishihara A, Fukami A, Matsuda Y, et al. Accumulation of indole-3-acetic acid in rice sl mutant leaves infected with Bipolaris oryzae. J Phytopathol. 2016;164:509–519.
- Lass-Flörl C, Wiedauer B, Mayr A, et al. Antifungal properties of 5-hydroxytryptamine (serotonin) against Aspergillus spp. in vitro. Int J Med Microbiol. 2002;291:655–657.10.1078/1438-4221-00174
- Langcake P, Pryce RJ. A new class of phytoalexins from grapevines. Experientia. 1977;33:151–152.10.1007/BF02124034
- Stoessl A. The antifungal factors in barley. IV. Isolation, structure, and synthesis of the hordatines. Can J Chem. 1976;45:1745–1760.
- Okazaki Y, Ishihara A, Nishioka T, et al. Identification of a dehydrodimer of avenanthramide phytoalexin in oats. Tetrahedron. 2004;60:4765–4771.10.1016/j.tet.2004.04.008
- Okazaki Y, Ishizuka A, Ishihara A, et al. New dimeric compounds of avenanthramide phytoalexin in oats. J Org Chem. 2007;72:3830–3839.10.1021/jo0701740
- Bouchenak-Khelladi Y, Salamin N, Savolainen V, et al. Large multi-gene phylogenetic trees of the grasses (Poaceae): progress towards complete tribal and generic level sampling. Mol Phylogenet Evol. 2008;47:488–505.10.1016/j.ympev.2008.01.035
- Soreng RJ, Peterson PM, Romaschenko K, et al. A worldwide phylogenetic classification of the Poaceae (Gramineae). J Syst Evol. 2015;53:117–137.10.1111/jse.12150
- Niemeyer HM. Hydroxamic acids (4-hydroxy-1,4-benzoxazin-3-ones), defence chemicals in the gramineae. Phytochemistry. 1988;27:3349–3358.10.1016/0031-9422(88)80731-3
- Niemeyer HM. Hydroxamic acids derived from 2-hydroxy-2H-1,4-benzoxazin-3(4H)-one: key defense chemicals of cereals. J Agric Food Chem. 2009;57:1677–1696.10.1021/jf8034034
- Kokubo Y, Nishizaka M, Ube N, et al. Distribution of the tryptophan-pathway-derived defensive secondary metabolites gramine and benzoxazinones in Poaceae. Biosci Biotechnol Biochem. 2017;81:431–440.