Abstract
We screened for factors involved in the cellulose-responsive induction of cellulose biomass-degrading enzyme genes from approximately 12,000 Aspergillus aculeatus T-DNA insertion mutants harboring a transcriptional fusion between the FIII-avicelase gene (cbhI) promoter and the orotidine 5′-monophosphate decarboxylase gene. Analysis of 5-fluoroorodic acid (5-FOA) sensitivity, cellulose utilization, and cbhI expression of the mutants revealed that a mutant harboring T-DNA at the dipeptidyl peptidase IV (dppIV) locus had acquired 5-FOA resistance and was deficient in cellulose utilization and cbhI expression. The deletion of dppIV resulted in a significant reduction in the cellulose-responsive expression of both cbhI as well as genes controlled by XlnR-independent and XlnR-dependent signaling pathways at an early phase in A. aculeatus. In contrast, the dppIV deletion did not affect the xylose-responsive expression of genes under the control of XlnR. These results demonstrate that DppIV participates in cellulose-responsive induction in A. aculeatus.
Graphical abstract
Dashed lines with arrows indicate putative signaling pathways.
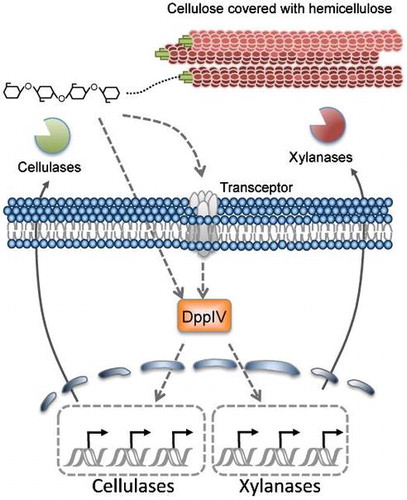
Aspergillus aculeatus no. F-50 [NBRC 108796], originally isolated from soil, produces enzymes that effectively hydrolyze pulp in combination with enzymes from Trichoderma reesei.Citation1) This functional synergism implies that A. aculeatus possesses different components of cellulolytic and hemicellulolytic enzymes than those in T. reesei. For example, A. aculeatus secretes a unique β-glucosidase 1 that hydrolyzes not only cello-oligosaccharides (up to cellopentaose), but also various disaccharides such as cellobiose and transglycosylation products.Citation2) Despite this capability, the quantities of enzymes secreted from A. aculeatus are too low to apply to enzymatically hydrolyse cellulosic biomass in industrial use. A comprehensive understanding of cellulase production mechanisms in A. aculeatus would contribute to the development of improved cellulolytic and hemicellulolytic enzyme production.
In filamentous fungi, cellulolytic enzyme production is mainly regulated at the transcriptional level in response to the presence of inducible carbon sources. The currently accepted view of cellulase and hemicellulase induction mechanisms is that cellulose and hemicellulose must first be hydrolyzed to oligosaccharides or monosaccharides by slightly and constitutively secreted enzymes.Citation3) These liberated saccharides trigger the expression of genes encoding hydrolases. Various saccharides are recognized as inducers of cellulase and hemicellulase production: d-xylose in A. niger,Citation4) cellobiose in A. oryzae and A. aculeatus,Citation5,6) and gentiobiose in Penicillium purpurogenum,Citation7) among others.Citation8) Several factors involved in this inducer recognition and induction of gene expression have been identified. In Neurospora crassa, two cellodextrin transporters, CDT-1 and CDT-2, contribute to inducer recognition and subsequent induction of gene expression.Citation9) CDT-1- and CDT-2-dependent cellulose signaling are not required for transportation of cellobiose into the cell; indicating the two proteins function as transceptors. Several pathway-specific transcription factors possessing the Zn(II)2Cys6 binuclear cluster domain have been identified in Aspergillus. XlnR participates in cellulose and xylose-responsive signaling pathways,Citation5,6,10) while ManR is involved in cellulose and mannose-responsive signaling pathways, ClbR and McmA function in the cellulose signaling pathway,Citation11,12) and AraR is associated with the arabinose signaling pathway.Citation13) Transcription factors induce genes in response to multiple physiological inducers. Despite all these findings, the factors involved in the signal transduction pathway remain unknown.
To aid elucidation of the cellulose-responsive signaling pathway, we established a positive screening system using the promoter of the FIII-avicelase gene (cbhI) fused to the orotidine 5′-monophosphate decarboxylase gene (pyrG) in A. aculeatus. The A. aculeatus strain harboring the reporter cassette was used to construct an A. aculeatus random T-DNA insertion mutant library by Agrobacterium tumefaciens-mediated transformation (AMT).Citation14) Screening for a cellulose-utilization deficient mutant from the library resulted in the identification of a novel cellobiose response regulator, ClbR.Citation11) We further screened for other factors involved in cellulose induction, which revealed that A. aculeatus dipeptidyl peptidase IV (AaDppIV) is involved—directly or indirectly—in the cellulose induction pathway.
Dipeptidyl peptidase (DPP), which is categorized into the serine peptidase SC clan S9B subfamily, is phylogenetically broadly distributed.Citation15) Human DPPIV specifically liberates dipeptides from the N-terminus of substrates comprising an average of 30 residues with a proline at the second position; it modulates biological activity by hydrolyzing peptide hormones, chemokines, and neuropeptides.Citation16) Ste13 in Saccharomyces cerevisiae, dipeptidyl aminopeptidase, is involved in α-factor maturation in yeast.Citation17) A. oryzae secretes three types of DPPs, namely DppB, DppE, and DppF with different substrate specificities.Citation18) The physiological function of DPPs in filamentous fungi remains unknown.
In this study, we found that deletion of the A. aculeatus dppIV gene (AadppIV) led to a reduction in gene expression in response to cellulose at the early phase in A. aculeatus. In A. aculeatus, two cellulose-induction pathways exist, namely, XlnR-dependent and XlnR-independent signaling pathways.Citation6) FIb-xylanase (xynIb) and FI-carboxymethyl cellulase (cmc1) genes are regulated via the XlnR-dependent signaling pathway, while cbhI, FII-carboxymethyl cellulase (cmc2), and FIa-xylanase (xynIa) are controlled by the XlnR-independent signaling pathway. The deletion of AadppIV resulted in reduced expression of the above-mentioned genes regulated via XlnR-dependent and XlnR-independent signaling pathways. This is the first report on the physiological function of DPPIV in filamentous fungi.
Materials and methods
Strains, transformation, and T-DNA insertion
All A. aculeatus strains used in this study were derived from wild-type A. aculeatus no. F-50. Unless otherwise stated, all strains were propagated at 30 °C in appropriately supplemented minimal medium (MM).Citation19)
A. aculeatus NCP2 (niaD1::niaD::PCBH1-pyrG; pyrG1) was used as a host to breed A. aculeatus T-DNA insertion strains by Agrobacterium tumefaciens-mediated transformation.Citation11) A. aculeatus MR12 (pyrG1; Δku80)Citation20) was used as a host for disruption and complementation of the dppIV gene. Escherichia coli DH5αF′ was used for plasmid construction.
Screening strategy for novel regulators
We screened for new factors controlling cellulose-induced gene expression using an A. aculeatus T-DNA insertion mutant library that was constructed as described previously.Citation11) Candidate cellulose-signaling deficient mutants were first selected by counter selection against 5-fluoroorodic acid (5-FOA), as A. aculeatus strain NCP2 harbors a reporter gene composed of the orotidine 5′-monophosphate decarboxylase gene (pyrG) under the control of the cbhI promoter.Citation11) Growth of the isolated 5-FOA-resistant strains was investigated on 1% (w/v) Avicel, 1% (w/v) alkali-swollen cellulose (ASC) medium, 1% (w/v) glucose, 1% (w/v) beechwood xylan, or 1% (w/v) d-xylose medium. The T-DNA insertion locus was determined by inverse PCR. Inverse PCR was performed using genomic DNA digested with EcoRI or XbaI-SpeI to amplify the right or left flanking regions of the T-DNA, respectively.Citation14)
Gene expression analyses
To obtain RNA for gene expression analyses, A. aculeatus strains were grown as described in Kunitake et al.Citation11) In this study, 0.1% polypeptone (Nihon Pharmaceutical, Tokyo, Japan) was used as an uninduced carbon source. The expression of tested genes was investigated by adding the indicated carbon source to media supplemented with 0.1% polypeptone. To assess the direct effect of cellobiose on gene expression, 50 μg/ml 1-deoxynojirimycin (DNJ) was added to media to avoid hydrolysis of cellobiose by β-glucosidase in A. aculeatus. Total RNA was isolated using TRIzol reagent as recommended by the manufacturer (Invitrogen/Life Technologies, Carlsbad, CA, USA). Gene expression levels were quantified by Northern blot hybridizationCitation21) and quantitative real-time PCR (RT-qPCR). Data from the Northern blot analyses were representative of biologic replicates performed in three independent experiments. For qPCR, total RNA (500 ng) was used to amplify cDNA using ReverTra Ace qPCR Master Mix with gDNA Remover (Toyobo, Osaka, Japan). The qPCR was performed in a Thermal Cycler Dice Real-Time system (Takara, Kyoto, Japan). For the amplification reactions, a SYBR Green I assay using THUNDERBIRD qPCR Mix (Toyobo) was performed in a reaction volume of 20 μl. The primers used for qPCR are listed in Table . Expression of the glyceraldehyde-3-phosphate dehydrogenase A gene (gpdA) was used as an internal control.
Table 1. Primers used in this study.
Disruption and complementation of dppIV
The A. aculeatus B7 mutant was transformed with pPTRII (Takara) harboring the dppIV fragment. The dppIV fragment, containing KpnI recognition sites, was amplified with the primer pair DPP4kpnF/DPP4kpnR to include 837 nt before the translation start site and 427 nt after the stop codon. The amplified DNA fragment was digested with KpnI and subcloned into the pPTRII kpnI site to yield pPTRII-dppIV.
The A. aculeatus dppIV-deficient mutant (pyrG1; Δku80; ΔdppIV::pyrG) was created by replacing the dppIV gene with the A. nidulans pyrG gene. To construct the dppIV disruption cassette, the 5′ and 3′ regions of the dppIV gene, which were required for homologous recombination, were amplified from A. aculeatus genomic DNA using primer pairs DPP4F/DPP4R and oDPP4F/oDPP4R, respectively. The A. nidulans pyrG gene (AnpyrG) was amplified from A. nidulans genomic DNA using the primer pair pyrG-dppF/pyrG-dppR. To eliminate AnpyrG by intramolecular homologous recombination at the dppIV locus, the flanking region on the 3′ side of dppIV was amplified using the primer pair mDPP4F/mDPP4R. The 5′ region, the region for marker recycling, AnpyrG, and the 3′ region were fused by PCR using the primer pair DPP4F/oDPP4R. The amplified fragment was introduced into MR12 (pyrG1; Δku80) by the protoplast-PEG method to yield the A. aculeatus ΔdppIV plus pyrG strain (pyrG1; Δku80; ΔdppIV::pyrG).Citation19,20) Marker recycling was performed by spreading 1 × 104 spores of transformants onto MM supplemented with 0.01% (w/v) uridine and 1 mM 5-FOA. After monospore isolation, A. aculeatus ΔdppIV (pyrG1; Δku80; ΔdppIV) was obtained. To complement A. aculeatus ΔdppIV, amplification of the dppIV promoter, open reading frame, and 3′-untranslated region was first carried out using the primer pair DPP4F/mDPP4R. AnpyrG and the 3′ region required for homologous recombination at the dppIV locus were then amplified with primer pairs pyrG-dppF/pyrG-dppR and cDPP4F/cDPP4R, respectively. The three DNA fragments were fused by PCR using the primer pair DPP4F/cDPP4R. Finally, A. aculeatus ΔdppIV was transformed with the amplified DNA fragments to yield the dppIV-complemented strain (pyrG1; Δku80; ΔdppIV::dppIV::pyrG).
DppIV activities
DppIV activities in MR12 and ΔdppIV were determined as described before with minor modification.Citation22) Conidia (1 × 106) of both strains were inoculated to 200 ml of minimal media supplemented with 0.2% (w/v) uridine and grown for 30 h at 30 °C. Mycelia were harvested and ground to a powder with a mortar and pestle in liquid nitrogen. Proteins were extracted by extraction buffer (50 mM Tris-HCl, pH 7.5, 0.1% Triton X-100). After a centrifugation at 140,000 x g, the supernatant was applied for Dpp assay. Final concentration of 0.4 mM Gly-Pro-p-nitroanilide was used as a substrate. The change in absorbance at 405 nm was kinetically monitored every 10 min for 60 min at 37 °C. The protein concentration was measured using the Bio-Rad Assay reagent (Bio-Rad Co., Tokyo, Japan).
Additional methods
Genomic DNA preparation and Southern blot analysis were performed as described previously.Citation11) The genomic sequence of the dppIV gene was obtained from our in-house A. aculeatus draft genome database. The dppIV cDNA sequence was determined by analyzing two independently amplified cDNA fragments.
Results
Characterization of a cellulose-utilization deficient mutant
We screened the A. aculeatus T-DNA insertion mutant library, consisting of approximately 12,000 transformants, for 5-FOA resistant strains. We identified five 5-FOA-resistant strains that exhibited slightly reduced growth on 1% (w/v) Avicel or 1% (w/v) ASC medium but normal growth on 1% (w/v) glucose, 1% (w/v) beechwood xylan, and 1% (w/v) d-xylose medium (data not shown). We initially focused on the phenotype of strain B7 after confirming the correlation between the function of the disrupted gene with the T-DNA insertion and the cellulose-utilization deficient phenotype.
To characterize the phenotype of the B7 strain, the expression of cbhI, whose promoter was used as the reporter, was quantified in B7 and control strain NCP2 by Northern blot analysis. The expression of cbhI was induced in response to 1% (w/v) Avicel in NCP2, whereas transcripts of cbhI were not detected in B7 (Fig. , lanes 1 and 2). These results indicated a good correlation with the cellulose-utilization deficient phenotype. We selected B7 as a mutant deficient for cellulose-responsive induction.
Fig. 1. Northern blot analysis of cbhI expression in NCP2 and B7.
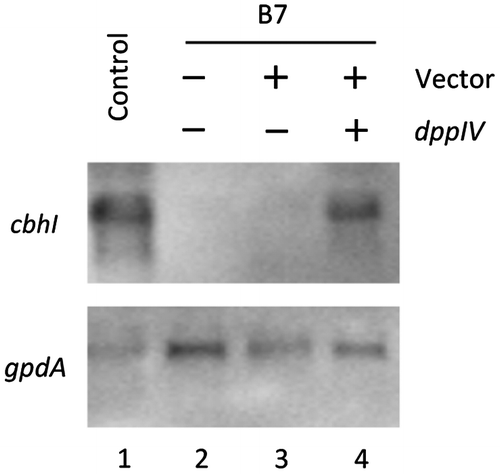
Because the approach needed to recover T-DNA flanking sequences by inverse PCR would differ depending on their integration patterns, we investigated the T-DNA integration pattern in B7 by Southern blot analysis. Genomic DNA isolated from B7 was digested separately with EcoRI, XbaI/SpeI, PstI, and HindIII. Single digestion by EcoRI (unique site located in the T-DNA left border), PstI (unique site located in the vector backbone), and HindIII (absent from the vector) yielded a single band (Fig. S1). Double digestion by XbaI and SpeI, with a unique site located in the T-DNA right border and absent, respectively, yielded two bands (Fig. S1a). In the case of T-DNA random insertion, T-DNA tends to be inserted into the recipient genome in various patterns, namely, tandem insertion of T-DNA, truncation of T-DNA, or insertion of T-DNA with the vector backbone.Citation14) Taking these points into consideration, we predicted that two copies of T-DNA with the vector backbone could be integrated into a single locus in the B7 genome (Fig. S1b). We tried to amplify the T-DNA flanking sequence by inverse PCR using B7 genomic DNA digested with only EcoRI or both XbaI and SpeI. Sequencing of the DNA fragment amplified by inverse PCR using B7 genomic DNA digested with EcoRI yielded the sequence beginning at the EcoRI site located 467 nt upstream of the translation start site of the putative dppIV gene (DDBJ Acc. No. LC186945). Nevertheless, the vector backbone hindered the recovery of the T-DNA flanking sequence, thereby preventing determination of the precise locus of the inserted T-DNA (Fig. S1b). These results demonstrate that the T-DNA was inserted into the dppIV locus even though PCR amplification of the other side of the T-DNA flanking region unfortunately failed.
To identify the genes conferring the cellulose-utilization deficient phenotype to B7, we introduced dppIV into B7 because the T-DNA is sometimes inserted into the recipient genome with a large deletion of the recipient genome sequence at the T-DNA inserted locus.Citation14) The reduced expression of cbhI in B7 was recovered by the introduction of pPTRII harboring the dppIV gene, but not by pPTRII alone (Fig. , lanes 3 and 4). Furthermore, introduction of the gene located downstream of dppIV did not restore the expression of cbhI (data not shown). These results strongly suggest that the cellulose-utilization deficient phenotype and the reduced expression of cbhI in B7 was caused by disruption of dppIV.
DppIV participates in the early phase of cellulose induction
To gain deeper insight into the function of dppIV, we deleted dppIV by homologous recombination (ΔdppIV pyrG+) followed by marker recycling to yield the A. aculeatus dppIV deletion mutant, namely ΔdppIV. ΔdppIV was also transformed with the dppIV DNA fragment to yield the complemented strain dppIV+ (Fig. S2a). We used Southern blot analysis to confirm that gene replacement and complementation occurred as expected (Fig. S2b). The ΔdppIV strain grows normally as well as the control strain does on 1% (w/v) Avicel, 1% (w/v) glucose, and 0.1% (w/v) polypeptone media. NCP2, the host strain of B7, shows slightly poorer growth than MR12 does, the host strain of ΔdppIV, in all tested condition; suggesting that slight reduction of cellulase production was critical on cellulose-utilization in B7 but not in ΔdppIV (data not shown). Furthermore, intracellular dipeptidyl peptidase activities in ΔdppIV were in the same level with those in the control strain (Fig. S3), while extracellular dipeptidyl peptidase activities were not detected in both strains.
To assess the functional role of dppIV in cellulose induction, transcripts of cbhI and cmc2 were quantified at 3 h postinduction in control, ΔdppIV, and dppIV+ strains. The expressions of cbhI and cmc2 in response to 1% (w/v) cellobiose supplemented with 50 μg/ml DNJ were reduced only in ΔdppIV, whereas no significant difference was observed under uninduced conditions in three strains. Consequently, the fold induction in ΔdppIV, i.e. the expression level under induced conditions divided by that under uninduced conditions, was reduced to approximately 40% of that in the host strain. The reduced expression of cbhI and cmc2 in ΔdppIV was restored in dppIV+ (Fig. ). Because cellobiose induced the expression of a limited number of genes, transcripts of cbhI, cmc2, xynIa, cmc1, and xynIb in the presence of ASC were quantified at 4, 6, and 8 h postinduction in control, ΔdppIV, and dppIV + strains. In the ΔdppIV strain, induction levels of cbhI, cmc2, and xynIa in response to 0.5% (w/v) ASC, regulated via the XlnR-independent signaling pathway, were significantly reduced, to 30–40% of that in the host strain, at 4 and 6 h postinduction, whereas no significant differences were observed at 8 h postinduction. The delayed induction of these genes was restored in dppIV+(Fig. a). Transcripts of cmc1 and xynIb, under the control of XlnR, were also decreased in the presence of ASC (Fig. b). Interestingly, however, the expressions of cmc1 and xynIb in response to 1% (w/v) beechwood xylan and 1% (w/v) xylose were not affected at all by the dppIV deletion (Fig. a and b). These results indicate that DppIV participates, directly or indirectly, in cellulose-responsive induction at an early phase in A. aculeatus. Because the effect of the dppIV deletion was limited to the early induction period, the deletion of dppIV affected neither the production of extracellular endoglucanase nor β-1,4-xylanase in the presence of wheat bran used as a cellulosic inducible carbon source (data not shown).
Fig. 2. Quantitative real-time PCR analysis of cbhI and cmc2 expression in the presence of 1% (w/v) cellobiose supplemented with 50 μg/ml 1-deoxynojirimycin in the MR12 parental strain (white bars), ΔdppIV (black bars), and dppIV+ (gray bars).
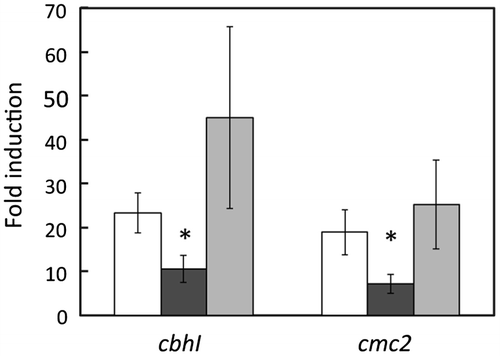
Fig. 3. Cellulase and xylanase gene expression in response to 0.5% (w/v) alkali-swollen cellulose (ASC) in MR12 (white bars), ΔdppIV (black bars), and dppIV+ (gray bars).
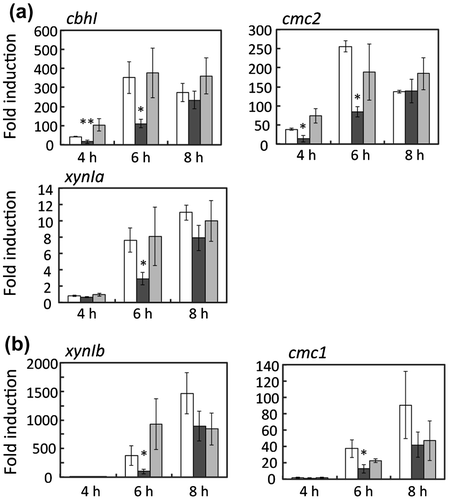
Fig. 4. Expression of xynIb and cmc1 in response to 1% (w/v) beechwood xylan (a) and 1% (w/v) xylose (b) in MR12 (white bars), ΔdppIV (black bars), and dppIV+ (gray bars).
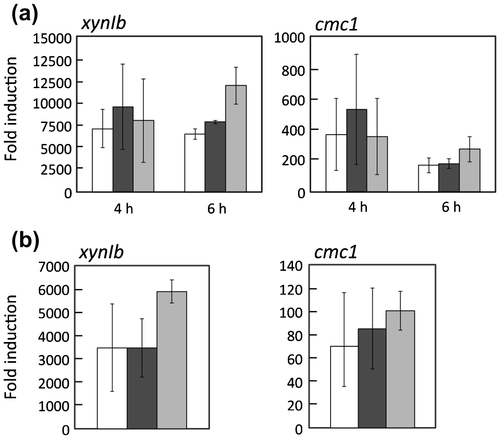
DppIV does not control transcription factor expression in A. aculeatus
ManR,Citation23) ClbR,Citation11) and XlnRCitation5,6) are known transcription factors controlling the expression of cellulase and xylanase genes in response to cellulosic carbon sources in Aspergillus. We thus next investigated the effect of the dppIV deletion on the expression of these genes in the presence of 1% (w/v) Avicel. No significant difference was observed in the expressions of the three tested transcription factor genes between ΔdppIV and MR12 at 4, 6, and 8 h postinduction (Fig. ), the same conditions under which cbhI expression was reduced.
Fig. 5. Expression of manR, clbR, and XlnR in response to 1% (w/v) Avicel in MR12 (white bars) and ΔdppIV (black bars).
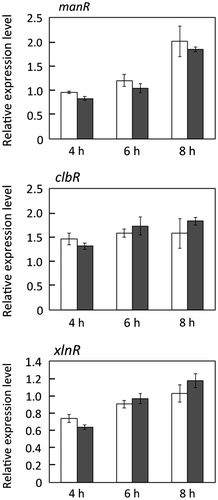
dppIV expresses under the carbon starvation condition
The expression profile of dppIV was quantified under the 1% (w/v) glucose, 1% (w/v) Avicel, and no carbon conditions at 8 h postinduction and the 1% (w/v) xylose condition at 4 h postinduction in MR12 (Fig. ). When the levels of dppIV expression under the glucose condition were assigned a value of 1, the expression levels of dppIV were 1.4, 9.6, and 15.1 under the xylose, Avicel, and no carbon conditions, respectively (Fig. ). The Avicel condition is similar to carbon starvation condition for A. aculeatus because A. aculeatus poorly grows on 1% (w/v) Avicel media. These data suggest that the presence of cellulosic carbon source under the carbon starvation condition stimulates the initial expression of the genes in response to cellulosic inducers via DppIV-dependent signaling pathway.
Fig. 6. Expression of dppIV under the 1% (w/v) glucose (Glc), 1% (w/v) xylose (Xyl), 1% (w/v) Avicel (Avi), and no carbon (None) conditions in MR12.
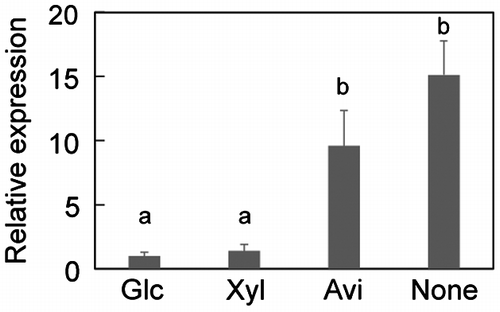
Discussion
In this study, we identified DppIV as a factor involved in cellulose-responsive induction in A. aculeatus by screening A. aculeatus T-DNA insertion mutants. We found that the deletion of dppIV in A. aculeatus caused delayed expressions of cbhI, cmc2, xynIa, cmc1, and xynIb in response to cellulose at early time points (4 and 6 h). According to the currently accepted gene regulation model for the expression of cellulase genes, cellulose needs to be hydrolyzed to oligosaccharides or monosaccharides by constitutively secreted enzymes.Citation3) Our data revealed that the expressions of cbhI and cmc2 in response to cellobiose, a physiological inducer, were also reduced in ΔdppIV; this suggests that AaDppIV is involved in the cellulose-responsive signaling pathway in A. aculeatus rather than constitutive cellulase secretion. In addition, the expressions of transcription factor genes manR, clbR, and xlnR were unaffected by the disruption of dppIV. The expression of manR in the presence of 1% (w/v) Avicel was reduced by the deletion of clbR (unpublished data). These results demonstrate that AaDppIV directly or indirectly participates in cellulose signaling pathways independent of ClbR- and ManR-dependent signaling pathways. To our knowledge, this is the first report on the physiological function of DppIV in filamentous fungi.
The deletion of A. aculeatus dppIV reduced gene expression in response to cellulosic inducers to approximately one-third of that of the control strain, but did not abolish it. Mining of our in-house A. aculeatus genome database revealed that four putative DPPs are encoded in the A. aculeatus genome: dipeptidyl peptidase III (DppIII), DppIV, dipeptidyl peptidase V (DppV), and dipeptidyl aminopeptidase B (DapB). The moderate effect of the dppIV deletion on expression of the tested genes could be due to partial complementation by these other DPPs. The same logic is also applicable to explain the reason why there was no significant difference on intracellular dipeptidyl peptidase activities in between the control and ΔdppIV strains (Fig. S3).
We note that substrate specificities and localizations of DPPs in filamentous fungi seem to differ from one another—even though the primary structure of DPPs is conserved. On the basis of DPP primary structure, filamentous fungal DppIV, DppV, and DapB peptidases are classified into peptidase family S9 and DppIII is assigned to peptidase family M49.Citation15) A. oryzae DppB and DppE, homologous to A. fumigatus and A. aculeatus DPPIV and DPPV, respectively, have different substrate specificities. A. oryzae DppB and A. fumigatus DPPIV are classified as Xaa-prolyl DPPs, whereas A. oryzae DppE and A. fumigatus DPPV are alanyl DPPs.Citation18,24,25) DppIV and DapB have the most similar primary structures among DPPs in filamentous fungi, but A. oryzae DppB and A. fumigatus DPPIV are extracellular peptidases, whereas A. niger DapB, a prolyl DPP, is an intracellular peptidase.Citation26) A. aculeatus DppIV is highly similar to A. fumigatus DPPIV, hydrolyzes Gly-Pro p-nitroanilide as a substrate, and possesses a signal peptide predicted by the SignalP 4.1 Server (http://www.cbs.dtu.dk/services/SignalP/). We questioned whether putative extracellular AaDppIV can participate in the cellulose responsive induction presumed to be an intracellular event. Histoplasma capsulatum DPPIVA (HcDPPIVA) possesses a signal peptide similar to Aspergillus DppIV, while H. capsulatum DppIVB (HcDPPIVB) possesses a putative transmembrane domain similar to Aspergillus DapB. In contrast to the function predicted on the basis of HcDppIVA and HcDppIVB primary structures, silencing of HcDPPIVA by RNA interference had no effect on secreted DppIV activity, whereas RNAi silencing of HcDPPIVB significantly reduced secreted DppIV activity.Citation27) These findings imply that AaDppIV can participate in the intracellular cellulose-signaling pathway, although comprehensive analyses of DPPIV in A. aculeatus are required.
Use of a positive screening system involving the PcbhI-pyrG reporter enabled us to identify factors involved in the cellulose-responsive induction pathway, namely, ClbRCitation11) and DppIV. Given that the deletion of each gene resulted in delayed gene expression in response to cellulosic carbon sources, it is interesting that factors participating in cellulose-responsive induction at the early phase were identified by counter selection against 5-FOA. As the initial expression of pyrG should have been low enough to acquire the 5-FOA resistant phenotype, the screening may have selected factors involved in the initiation of the cellulose-responsive induction or transition from repressed, unrepressed, or carbon starvation conditions to the induced condition. In filamentous fungi, cellulose-responsive gene expression is under the control of various factors, such as transceptors,Citation9) transcription activators, Clr-1/ClrA,Citation28) Clr-2/ClrB/ManR,Citation23,28) ClbR,Citation11) XlnR,Citation5) and the transcriptional repressor CreA.Citation29) In addition, secretion of cellulolytic enzymes is under the control of MsbA, which influences the growth of A. nidulans under nutrient-poor conditions (including lignocellulose), even though the molecular mechanisms of MsbA remain unknown.Citation30) AaDppIV may control the stability of various factors, including putative regulators interacting with or controlling the activity of the above factors under nutrient poor conditions (Fig. ). DppIV in filamentous fungi is known to hydrolyze chromogenic substrates, while physiological substrates of DppIV have not been reported. Human DPPIV only hydrolyzes N-termini of peptides; however, the idea that fungal DppIV possesses the same enzymatic properties as human DPPIV is probably unrealistic. Fungal DppIV as well as human DP8 and DP9 may not have so narrow spectrum of substrate specificities as human DPPIV. Humans possess additional serine peptidase SC clan S9B members, namely DP8 and DP9, which are predicted to hydrolyze enzymes such as adenylate kinase.Citation31) A logical next step will be the identification of the specific substrate of DppIV involved in cellobiose- and cellulose-responsive induction in A. aculeatus. Uncovering the specific substrates of AaDppIV related to cellulose-responsive induction should greatly assist determination of the functions of DppIV in physiological processes.
Author contributions
All members conceived and designed the experiments, and discussed the results for the completion of the manuscript. ST, SY, and EK performed the experiments. The manuscript was written by ST, commented and revised by all of the authors.
Disclosure statement
No potential conflict of interest was reported by the authors.
Funding
This work was supported by Japan Society for the Promotion of Science (JSPS) KAKENHI [grant number JP25450110]; the New Energy and Industrial Technology Development Organization (NEDO) Project [grant number P07015].
Supplemental materials
The supplemental material for this paper is available at http://dx.doi.org/10.1080/09168451.2017.1295800
TBBB_1295800_Supplemental_information.pdf
Download PDF (850.5 KB)References
- Murao S, Kanamoto J, Arai M. Isolation and identification of a cellulolytic enzyme producing microorganism. J Ferment Technol. 1979;57:151–156.
- Baba Y, Sumitani J, Tani S, et al. Characterization of Aspergillus aculeatus beta-glucosidase 1 accelerating cellulose hydrolysis with Trichoderma cellulase system. AMB Express. 2015;5:3.
- Kubicek CP, Messner R, Gruber F, et al. The Trichoderma cellulase regulatory puzzle: from the interior life of a secretory fungus. Enzyme Microb Technol. 1993;15:90–99.10.1016/0141-0229(93)90030-6
- de Souza WR, de, Gouvea PF, Savoldi M, et al. Transcriptome analysis of Aspergillus niger grown on sugarcane bagasse. Biotechnol Biofuels. 2011;4:40.10.1186/1754-6834-4-40
- Marui J, Kitamoto N, Kato M, et al. Transcriptional activator, AoXlnR, mediates cellulose-inductive expression of the xylanolytic and cellulolytic genes in Aspergillus oryzae. FEBS Lett. 2002;528:279–282.10.1016/S0014-5793(02)03328-8
- Tani S, Kanamasa S, Sumitani J, et al. XlnR-independent signaling pathway regulates both cellulase and xylanase genes in response to cellobiose in Aspergillus aculeatus. Curr Genet. 2012;58:93–104.10.1007/s00294-012-0367-5
- Kurasawa T, Yachi M, Suto M, et al. Induction of cellulase by gentiobiose and its sulfur-containing analog in Penicillium purpurogenum. Appl Environ Microbiol. 1992;58:106–110.
- Tani S, Kawaguchi T, Kobayashi T. Complex regulation of hydrolytic enzyme genes for cellulosic biomass degradation in filamentous fungi. Appl Microbiol Biotechnol. 2014;98:4829–4837.10.1007/s00253-014-5707-6
- Znameroski EA, Li X, Tsai JC, et al. Evidence for transceptor function of cellodextrin transporters in Neurospora crassa. J Biol Chem. 2014;289:2610–2619.10.1074/jbc.M113.533273
- van Peij N, Gielkens M, de Vries R, et al. The transcriptional activator XlnR regulates both xylanolytic and endoglucanase gene expression in Aspergillus niger. Appl Environ Microbiol. 1998;64:3615–3619.
- Kunitake E, Tani S, Sumitani J, et al. A novel transcriptional regulator, ClbR, controls the cellobiose- and cellulose-responsive induction of cellulase and xylanase genes regulated by two distinct signaling pathways in Aspergillus aculeatus. Appl Microbiol Biotechnol. 2013;97:2017–2028.10.1007/s00253-012-4305-8
- Yamakawa Y, Endo Y, Li N, et al. Regulation of cellulolytic genes by McmA, the SRF-MADS box protein in Aspergillus nidulans. Biochem Biophys Res Commun. 2013;431:777–782.10.1016/j.bbrc.2013.01.031
- Battaglia E, Hansen SF, Leendertse A, et al. Regulation of pentose utilisation by AraR, but not XlnR, differs in Aspergillus nidulans and Aspergillus niger. Appl Microbiol Biotechnol. 2011;91:387–397.10.1007/s00253-011-3242-2
- Kunitake E, Tani S, Sumitani J, et al. Agrobacterium tumefaciens-mediated transformation of Aspergillus aculeatus for insertional mutagenesis. AMB Express. 2011;1:46.
- Rawlings ND, Barrett AJ, Finn R. Twenty years of the MEROPS database of proteolytic enzymes, their substrates and inhibitors. Nucleic Acids Res. 2016;44:D343–D350.10.1093/nar/gkv1118
- Mentlein R, Dipeptidyl-peptidase IV. Dipeptidyl-peptidase IV (CD26)-role in the inactivation of regulatory peptides. Regulatory Peptides. 1999;85:9–24.10.1016/S0167-0115(99)00089-0
- Julius D, Blair L, Brake A, et al. Yeast α factor is processed from a larger precursor polypeptide: the essential role of a membrane-bound dipeptidyl aminopeptidase. Cell. 1983;32:839–852.10.1016/0092-8674(83)90070-3
- Maeda H, Sakai D, Kobayashi T, et al. Three extracellular dipeptidyl peptidases found in Aspergillus oryzae show varying substrate specificities. Appl Microbiol Biotechnol. 2016;100:4947–4958.10.1007/s00253-016-7339-5
- Adachi H, Tani S, Kanamasa S, et al. Development of a homologous transformation system for Aspergillus aculeatus based on the sC gene encoding ATP-sulfurylase. Biosci Biotechnol Biochem. 2009;73:1197–1199.10.1271/bbb.80772
- Tani S, Tsuji A, Kunitake E, et al. Reversible impairment of the ku80 gene by a recyclable marker in Aspergillus aculeatus. AMB Express. 2013;3.
- Kunitake E, Kawamura A, Tani S, et al. Effects of clbR overexpression on enzyme production in Aspergillus aculeatus vary depending on the cellulosic biomass-degrading enzyme species. Biosci Biotechnol Biochem. 2015;79:488–495.10.1080/09168451.2014.982501
- Doumas A, van Den Broek P, Affolter M, et al. Characterization of the prolyl dipeptidyl peptidase gene (dppIV) from the Koji Mold Aspergillus oryzae. Appl Environ Microbiol. 1998;64:4809–4815.
- Ogawa M, Kobayashi T, Koyama Y. ManR, a transcriptional regulator of the β-mannan utilization system, controls the cellulose utilization system in Aspergillus oryzae. Biosci Biotechnol Biochem. 2013;77:426–429.10.1271/bbb.120795
- Beauvais A, Monod M, Debeaupuis JP, et al. Biochemical and antigenic characterization of a new dipeptidyl-peptidase isolated from Aspergillus fumigatus. J Biol Chem. 1997;272:6238–6244.10.1074/jbc.272.10.6238
- Beauvais A, Monod M, Wyniger J, et al. Dipeptidyl-peptidase IV secreted by Aspergillus fumigatus, a fungus pathogenic to humans. Infect Immun. 1997;65:3042–3047.
- Jalving R, Godefrooij J, Veen WJ, et al. Characterisation of the Aspergillus niger dapB gene, which encodes a novel fungal type IV dipeptidyl aminopeptidase. Mol Genet Genomics. 2005;273:319–325.10.1007/s00438-005-1134-9
- Cooper KG, Woods JP. Secreted dipeptidyl peptidase IV activity in the dimorphic fungal pathogen Histoplasma capsulatum. Infect Immun. 2009;77:2447–2454.10.1128/IAI.01345-08
- Coradetti S, Craig J, Xiong Y, et al. Conserved and essential transcription factors for cellulase gene expression in ascomycete fungi. Proc Nat Acad Sci USA. 2012;109:7397–7402.10.1073/pnas.1200785109
- Dowzer CE, Kelly JM. Cloning of the creA gene from Aspergillus nidulans: a gene involved in carbon catabolite repression. Curr Genet. 1989;15:457–459.10.1007/BF00376804
- Brown NA, Dos Reis TF, Goinski AB, et al. The Aspergillus nidulans signalling mucin MsbA regulates starvation responses, adhesion and affects cellulase secretion in response to environmental cues. Mol Microbiol. 2014;94:1103–1120.
- Wilson CH, Indarto D, Doucet A, et al. Identifying natural substrate for dipeptidyl peptidases 8 and 9 using terminal amine isotopic labeling of substrates (TAILS) reveals in vivo roles in cellular homeostasis and energy metabolism. J Biol Chem. 2013;288:13936–13949.10.1074/jbc.M112.445841