Abstract
Flavan-3-ol derivatives are common plant-derived bioactive compounds. In particular, (–)-epigallocatechin-3-O-gallate shows various moderate biological activities without severe toxicity, and its health-promoting effects have been widely studied because it is a main ingredient in green tea and is commercially available at low cost. Although various biologically active flavan-3-ol derivatives are present as minor constituents in plants as well as in green tea, their biological activities have yet to be revealed, mainly due to their relative unavailability. Here, I outline the major factors contributing to the complexity of functionality studies of flavan-3-ol derivatives, including proanthocyanidins and oligomeric flavan-3-ols. I emphasize the importance of conducting structure-activity relationship studies using synthesized flavan-3-ol derivatives that are difficult to obtain from plant extracts in pure form to overcome this challenge. Further discovery of these minor constituents showing strong biological activities is expected to produce useful information for the development of functional health foods.
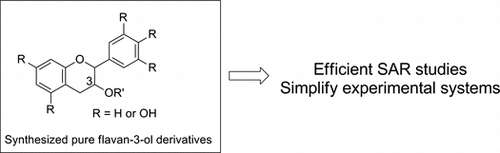
There is currently great interest in the investigation of compounds from food sources with biological activities, as these compounds are generally considered highly safe because they are consumed as part of the general daily diet. In particular, polyphenols are thought to have various health benefits and are found in many health foods, as well as in vegetables and fruits.Citation1,2) However, the structure-activity relationships (SARs) of polyphenols are not well understood, because they are typically obtained as a mixture of various analogs, which makes purification difficult. The flavan-3-ol series is one of the most well-known groups of biologically active polyphenol compounds, including (–)-epigallocatechin-3-O-gallate (EGCG) (1), (+)-catechin (2), and (–)-epicatechin (3) (Fig. ), which are often referred to simply as catechins. (–)-EGCG (1) tends to have higher activity than (+)-catechin (2) and (–)-epicatechin (3) owing to the greater number of phenolic hydroxyl groups and the presence of a galloy moiety. Although other various flavan-3-ols are also found naturally as minor components in plants, it has thus far been difficult to conduct detailed investigations of the wide variety of these compounds with standard biological assays because they are only available in limited quantities.
Proanthocyanidins, generally referred to as condensed tannins, are compounds that are particularly difficult to isolate in pure form but are known to have various strong biological activities (Fig. ).Citation1,2) Since they are built of flavan-3-ols, i.e., (–)-EGCG (1), (+)-catechin (2), and (–)-epicatechin (3), or their derivatives found as various analogs in plants, there is a large variety of structural isomers of proanthocyanidins. Thus, the SARs of proanthocyanidins remain unclear, because of the challenges associated with the purification and identification of each compound.
In general, bioavailability estimations of plant polyphenols, including flavan-3-ols and proanthocyanidins, are considered to be difficult owing to non-specific interactions with various proteins.Citation3) Therefore, in many cases, plant polyphenols are excluded from clinical trials for drug development. However, many recent analytical studies on the interactions between polyphenols and proteins have revealed that such interactions are not as non-specific as previously thought. Consequently, there is a need to reveal the SARs and protein-binding abilities of flavan-3-ols, proanthocyanidins, and their derivatives to establish their most efficient use with respect to health maintenance and medical treatment in the near future.
In this review, I describe the difficulties associated with studies to reveal the functions of flavan-3-ol derivatives, and the importance of conducting SAR studies using pure flavan-3-ol derivatives that are particularly difficult to obtain from plant extracts.
I. Structural complexity of flavan-3-ols and their derivatives
The basic structure of flavan-3-ol is shown in Fig. . Flavan-3-ols are defined as the analogs of 2-phenyl-3,4-dihydro-2H-chromen-3-ol, and have a characteristic three-ring structure including two aromatic rings that are substituted by several hydroxyl groups. Various structurally related compounds such as structural isomers, stereoisomers, and modified derivatives have been isolated as bioactive components from a wide variety of plants.Citation1,2)
In addition, proanthocyanidins, oligomeric secondary metabolites of flavan-3-ols or condensed tannins, are known as extremely strong agents with multiple biological activities. The basic structure of proanthocyanidins is shown in Fig. Citation4,5); however, the structures of proanthocyanidins are complicated by the specific structure of the flavan-3-ol unit, including the number of hydroxyl groups, substituted positions of hydroxyl groups, and the absolute stereochemistry of the chiral carbons. In addition, there are different condensation positions, 6 and 8, on the A ring, resulting in branched structural isomers, as shown in Fig. . Furthermore, “A-type” proanthocyanidins have been identified as crucially strong biologically active proanthocyanidins (Fig. ). Oomori and colleagues recently reported an excellent stereoselective synthesis method of a 4–6 condensed catechin dimerCitation6,7) and A-type proanthocyanidins.Citation8) Moreover, several reviews of recent studies for the synthesis of various proanthocyanidins have also been published.Citation9–11) Continued progress in synthetic methodology will contribute to gaining a greater understanding of the individual functions and SARs to best exploit the biological activities of proanthocyanidins.
II. Biological activities of pure flavan-3-ol derivatives
Various biological activities of polyphenolic compounds have been reported to date. Among them, flavan-3-ol derivatives have received substantial attention for their potential health-promoting benefits, which are believed to arise mainly from their antioxidant activity.Citation12) In addition, there are many reports related to other biological activities of flavan-3-ol derivatives, such as antifungal.Citation13,14) antiviral,Citation13) anti-inflammatory,Citation15) anticancer,Citation16) and anti-angiogenic activities,Citation17) and roles in the protection against neurologicalCitation18) and heart diseases,Citation19) among others. Proanthocyanidins generally show strong activities even though the individual activities of their monomeric flavan-3-ols are weak. Our previous SAR studies also indicated that a longer oligomeric structure is necessary for proanthocyanidins to exert strong activity against inhibition of DNA polymerases.Citation20,21) Furthermore, the oligomeric structure was also shown to be required for cancer cell proliferation inhibitory activityCitation22) and antiviral activities.Citation23) However, long oligomers over the pentamer range are often toxic to normal cells as well as to cancer cells in in vitro assays,Citation22) suggesting that a long flavan-3-ol oligomer may be unsuitable for use in health maintenance. In addition to the oligomer length, the presence of gallo-moieties plays a major role in the various biological activities of flavan-3-ols. Multiple gallo-moieties in one molecule of flavan-3-ol have a huge effect on exerting biological activities.Citation24) Interestingly, the effect of gallo-moieties is potentially limited depending on the introduced position and its stereochemistry. We synthesized di-galloyl-modified flavan-3-ols 4 and 5, which were derived from (–)-epicatechin and (+)-catechin, respectively (Fig. ). The inhibitory activities of compounds 1, 4, and 5 against HeLa S3 cell proliferation are shown in Fig. . Compound 4 showed significantly higher activity compared to compounds 1 and 5.Citation24) These results indicated that the stereochemistry of the 3-position is important for inhibition of HeLa S3 cells proliferation.
Fig. 7. Inhibitory activity of flavan-3-ols derivatives against HeLa S3 cells. HeLa S3 cells were incubated with 1 μL of each compound in dimethyl sulfoxide (DMSO) solution at final concentration 10, 50, and 100 μM (n = 12) for 48 h. DMSO solution alone was used as a control. Error bars represent standard deviations of the mean (n = 12).
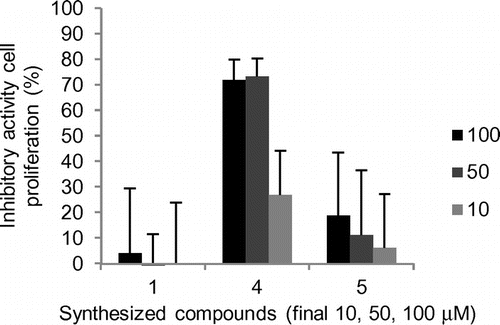
Moreover, partial acetylation of flavan-3-ol derivatives, which increased the lipophilic property, was reported to enhance the biological activities of flavan-3-ols as effective inhibitors of the proteasome and inducers of cancer cell apoptosis.Citation25) We have also reported that acetylation of the DEF moiety of procyanidin B1 (6), a dimeric flavan-3-ol, (–)-epicatechin-(4–8)-(+)-catechin dimer, enhanced the inhibitory activity against HeLa S3 cell proliferation (Fig. ).Citation26) By contrast, acetylation of the ABC moiety of procyanidin B1 could not effectively enhance the activity, and the DEF moiety acetylated derivative (7), an analog of the (+)-catechin-(4–8)-(+)-catechin dimer named procyanidin B3, showed weak cell proliferation inhibitory activity.Citation27) These results suggest that the antioxidant activity caused by the phenolic hydroxyl groups is not essential for HeLa S3 cell proliferation inhibition. These findings further indicated that the (–)-epicatechin unit of 6 is important for its activity.
The wide variety of biological activities of flavan-3-ol derivatives adds additional complexity to their study and development for application. Of particular interest, short-term treatment of cells with proanthocyanidins was shown to accelerate the proliferation of 3T3-L1 mouse fibroblast cells.Citation28) We have also consistently observed this phenomenon in that some treatments of flavan-3-ol derivatives accelerated cell proliferation when applied at low concentrations using our cell culture assay systems. This suggests the possibility that the specific assay method and conditions (e.g., concentration) can influence the observed biological activities of flavan-3-ol derivatives.
Biological activity measurements are commonly performed using a mixture of plant extracts, and the results are expressed as the sum of activities exerted by various compounds. As described above, there is a large variety of flavan-3-ol derivatives, and their metabolic pathways are overly complicated and thus poorly understood. It is therefore necessary to confirm the activity and functionality of each compound and conduct related SAR studies to understand their potential applications. Toward this end, organic synthesis, by which various isomers and analogs are provided in pure form simultaneously, would be the best methodology to fill this knowledge gap.
III. Interactions between flavan-3-ol derivatives and proteins
Tannins are cocktails extracted from vegetables that are used in the vegetable “tanning” process of converting animal skin to leather, which relies on the polyphenol–collagen protein interaction in the skin. In general, polyphenol compounds are considered to interact with various proteins in a non-specific manner, and such non-specific interactions are commonly considered as a large contributor to the poor bioavailability of polyphenols.Citation3,29) However, considerable recent research has suggested that these interactions between polyphenols and proteins might actually be more specific than previously thought. EGCG (1) binds to the 67-kDa laminin receptor, which is widely expressed at high levels in cancer cell membranes, resulting in the selective cytotoxic activity of EGCG (1) against cancer cells.Citation30) By contrast, binding studies between and EGCG (1) and various proteins such as fibrinogen,Citation31) serum albumin,Citation32) and ovalbuminCitation33) have revealed a further level of complexity in the interactions between flavan-3-ol derivatives and proteins. Furthermore, proanthocyanidins interact strongly with various proteins in a non-specific manner. In particular, the non-specific binding property of proanthocyanidins with bovine serum albumin (BSA) is commonly used for the quantification of total proanthocyanidin.Citation34)
Nevertheless, it is not clear whether these types of binding interactions with proteins are related to their degree of effectiveness in various in vitro and in vivo assays. In our previous study,Citation24) the interactions between BSA, a protein present in large amounts in the cell culture medium, and flavan-3-ol derivatives immobilized on beads by the photo-affinity methodCitation35) were investigated. However, if BSA competes with a target of flavan-3-ol, the activities of the cell-based assay would be underestimated. With respect to digalloyl derivatives, there was little difference detected between the effects of the strong cell proliferation inhibitor 4 and the non-active compound 5 (chemical structures are shown in Fig. ); thus, in this case, non-specific interaction with BSA did not affect the difference of activity between compounds 4 and 5.Citation24)
To evaluate the functionality of flavan-3-ol derivatives, it is essential to analyze their non-specific properties and binding modes. This will require synthesizing various flavan-3-ol derivatives and determining the differences of their individual non-specific binding properties.
IV. The additional complexity of flavan-3-ol metabolism for research
Flavan-3-ol derivatives are also converted to various structurally complex secondary polyphenols through chemical reactions that occur during food processing such as drying,Citation36) frozen storage,Citation37) and acidic treatment.Citation38) These complex and diverse forms make the isolation, structural determination, and evaluation of the biological activities of each compound much more difficult, which has limited applications and developments of flavan-3-ol derivatives as pharmaceutical agents despite their high potential.
Therefore, the development of new methods to elucidate the biosynthesis scheme, polymerization mechanisms, and transport of flavan-3-ol derivatives has become an important and active research theme in the field.Citation39,40) In particular, development of a method to control the biosynthesis of flavan-3-ol derivatives with strong biological activities in plants would be an incredibly valuable tool to produce highly functional plants and foods. The production of polyphenol compounds is affected by light irradiation, which can be used to increase the amount of polyphenolic secondary metabolites, including flavan-3-ols. For example, short-term ultraviolet C irradiation was shown to stimulate biosynthetic pathways, leading to enhancement of the accumulation of polyphenol compounds.Citation41) Similarly, long-term irradiation of visible blue light also showed an effect on the amount of flavan-3-ols produced.Citation42) These reports demonstrate the possibility of controlling the amounts of specific polyphenol compounds, including flavan-3-ol derivatives, in plants, and also indicate that environmental conditions have a large influence on polyphenol production, which causes difficulty in the functional evaluations of plant extracts.
Furthermore, the metabolism and biotransformation processes of flavan-3-ol derivatives in vivo are also substantially complex and remain poorly understood, although such knowledge is essential to elucidate the mechanisms of the functionality of flavan-3-ols in vivo. Several reports have shown that a small amount of intact procyanidin, a proanthocyanidin with two hydroxyl groups on the B ring, was detected in the blood after oral intake in mammals. Procyanidin B1, a dimeric flavan-3-ol, (–)-epicatechin-(4,8)-(+)-catechin, was detected in human serumCitation43); procyanidin B4, (+)-catechin-(4,8)-(–)-epicatechin, was detected in the urine and plasma of pigs in conjunction with monomeric flavan-3-ols and other metabolitesCitation44); and some metabolites of monomeric flavan-3-ols were recently identified in the rat microbiota.Citation45–50) Moreover, the biological activities of flavan-3-ols have been reported to change or become stronger through metabolic reactions and interactions. A mixture of metabolites of an A-type epicatechin dimer in the rat intestinal microbiota showed an increase in antioxidant capacity, whereas the antioxidant capacity of B-type epicatechin dimer metabolites decreased in this same mixture.Citation51
Collectively, these examples demonstrate that reproducible and accurate SAR studies of flavan-3-ol derivatives are complicated by the diversity of the structures of flavan-3-ols themselves, as well as by the various factors that act to modify, oligomerize, and decompose the molecules involved in their metabolic pathways. These problems in the study and applications of flavan-3-ol derivatives introduce attractive challenging research themes, garnering the interest of many scientists in this field.
V. Conclusion
Numerous biologically active flavan-3-ol derivatives are ingested every day, and are generally considered to be compounds with health benefits. However, their biological activities and functions have yet to be revealed because of their general unavailability and complexity. To resolve this problem, I propose that SAR studies using synthesized pure flavan-3-ol derivatives would be effective to simplify the experimental systems. Although there are still many difficulties to overcome in research to evaluate the functionality of flavan-3-ols, SAR studies would certainly provide useful information for their exploitation in health maintenance and for the development of health-promoting, functional foods. Thus, although research on flavan-3-ol derivatives is complex, it remains a challenging theme and great opportunity to advance the field.
Disclosure statement
No potential conflict of interest was reported by the author.
Funding
The related work on flavan-3-ol derivatives described herein was supported by BRAIN: Program for Promotion of Basic and Applied Research for Innovations in Bio-oriented Industry; Technology Research Promotion Program for Agriculture; Forestry, Fisheries, Food Industry; the Tojuro Iijima Foundation for Food Science and Technology; and the Science Research Promotion Fund 2016 from the Japan Private School Promotion Foundation.
Acknowledgments
I am deeply grateful to all of the scientists who have supported and encouraged this work. Without their help, this research would not have been possible. I would like to particularly express my greatest appreciation to Dr. T. Oritani, Dr. A. Tanaka, Dr. H. Kiyota, Dr. H. Osada, Dr. N. Kanoh, Dr. M. Ubukata, Dr. N. Nakajima, and Dr. N. Matsuura.
Notes
This review was written in response to the author’s receipt of the JSBBA Award for Young Scientists in 2012.
References
- Harborne JB. The flavonoids: advances in research from 1986. London: Chapman and Hall; 1993.
- Harborne JB, Baxter H. The handbook of natural flavonoids. New York (NY): Wiley; 1999.
- Haslam E. Natural polyphenols (vegetable tannins) as drugs: possible modes of action. J Nat Prod. 1996;59:205–215.10.1021/np960040+
- Fine AM. Oligomeric proanthocyanidin complexes: history, structure, and phytopharmaceutical applications. Altern Med Rev. 2000;5:144–151.
- Cos P, De Bruyne T, Hermans N, et al. Proanthocyanidins in health care: current and new trends. Curr Med Chem. 2013;10:1345–1359.
- Watanabe G, Ohmori K, Suzuki K. First regiocontrolled synthesis of procyanidin B6, a catechin dimer with rare connectivity: a halo-capping strategy for formation of 4,6-interflavan bonds. Chem Commun. 2013;49:5210–5212.10.1039/c3cc41993b
- Watanabe G, Ohmori K, Suzuki K. A seco-catechin cyclization approach to 4→6-linked catechin dimers. Chem Commun. 2014;50:14371–14373.10.1039/C4CC06390B
- Ito Y, Ohmori K, Suzuki K. Annulation approach to doubly linked (A-type) oligocatechins: synthesis of (+)-Procyanidin A2 and (+)-Cinnamtannin B1. Angew Chem Int Ed. 2014;53:10129–10133.10.1002/anie.201405600
- Ferreira D, Coleman CM. Towards the synthesis of proanthocyanidins: half a century of innovation. Planta Med. 2011;77:1071–1085.10.1055/s-0030-1270908
- Makabe H. Synthesis and anti-tumor activity of proanthocyanidins. Heterocycles. 2013;87:2225–2248.10.3987/REV-13-782
- Oyama K, Yoshida K, Kondo T. Recent progress in the synthesis of flavonoids: from monomers to supra-complex molecules. Curr Organic Chem. 2011;15:2567–2607.10.2174/138527211796367354
- Moon JK, Shibamoto T. Antioxidant assays for plant and food components. J Agric Food Chem. 2009;57:1655–1666.10.1021/jf803537k
- Cushnie TPT, Lamb AJ. Antimicrobial activity of flavonoids. Int J Antimicrob Agents. 2005;26:343–356.10.1016/j.ijantimicag.2005.09.002
- Dhanasekaran D, Thajuddin N, Panneerselvam A. Fungicides for plant and animal diseases. Croatia: InTech; 2012.10.5772/1130
- Mena P, Domínguez-Perles R, et al. Flavan-3-ols, anthocyanins, and inflammation. IUBMB Life. 2014;66:745–758.10.1002/iub.v66.11
- Du GJ, Zhang Z. Epigallocatechin gallate (EGCG) is the most effective cancer chemopreventive polyphenol in green tea. Nutrients. 2012;4:1679–1691.10.3390/nu4111679
- Pesca MS, Dal Piaz F, Sanogo R, et al. Bioassay-guided isolation of proanthocyanidins with antiangiogenetic activities. J Nat Prod. 2013;76:29–35.10.1021/np300614u
- Uttara B, Singh AV, Zamboni P, et al. Oxidative stress and neurodegenerative diseases: a review of upstream and downstream antioxidant therapeutic options. Curr Neuropharmacol. 2009;7:65–74.10.2174/157015909787602823
- Wang CZ, Mehendale S, Calway T, et al. Botanical flavonoids on coronary heart disease. Am J Chin Med. 2011;39:661–671.10.1142/S0192415X1100910X
- Saito A, Mizushina Y, Ikawa H, et al. Systematic synthesis of galloyl-substituted procyanidin B1 and B2, and their ability of DPPH radical scavenging activity and inhibitory activity of DNA polymerases. Bioorg Med Chem. 2005;13:2759–2771.10.1016/j.bmc.2005.02.023
- Saito A, Mizushina Y, Tanaka A, et al. Versatile synthesis of epicatechin series procyanidin oligomers, and their antioxidant and DNA polymerase inhibitory activity. Tetrahedron. 2009;65:7422–7428.10.1016/j.tet.2009.07.018
- Kozikowski AP, Tückmantel W, Böttcher G, et al. Studies in polyphenol chemistry and bioactivity. 4. (1) Synthesis of trimeric, tetrameric, pentameric, and higher oligomeric epicatechin-derived procyanidins having all-4beta,8-interflavan connectivity and their inhibition of cancer cell growth through cell cycle arrest. J Org Chem. 2003;68:1641–1658.10.1021/jo020393f
- Chandrasekaran L, Ichiyama K, Chen VF, et al. In silico study verifying antiviral activity of proanthocyanidins with special reference to dengue virus. Front Anti-infect Drug Discov. 2014;3:317–338.
- Mori K, Ayano Y, Hamada Y, et al. Role of 2,3-cis structure of (–)-epicatechin-3,5-O-digallate in inhibition of HeLa S3 cell proliferation. Nat Prod Chem Res. 2015;3:172.
- Osanai K, Landis-Piwowar KR, Dou QP, et al. A para-amino substituent on the D-ring of green tea polyphenol epigallocatechin-3-gallate as a novel proteasome inhibitor and cancer cell apoptosis inducer. Bioorg Med Chem. 2007;15:5076–5082.10.1016/j.bmc.2007.05.041
- Okamoto S, Ishihara S, Okamoto T, et al. Inhibitory activity of synthesized acetylated procyanidin B1 analogs against HeLa S3 cells proliferation. Molecules. 2014;19:1775–1785.10.3390/molecules19021775
- Saito A, et al. unpublished data.
- Tsuruya M, Niwano Y, Nakamura K, et al. Acceleration of proliferative response of mouse fibroblasts by short-time pretreatment with polyphenols. Appl Biochem Biotechnol. 2014;174:2223–2235.10.1007/s12010-014-1124-7
- D’Archivio M, Filesi C, Varì R, et al. Bioavailability of the polyphenols: status and controversies. Int J Mol Sci. 2010;11:1321–1342.10.3390/ijms11041321
- Tachibana H, Koga K, Fujimura Y, et al. A receptor for green tea polyphenol EGCG. Nat Struct Mol Biol. 2004;11:380–381.10.1038/nsmb743
- Suzuki Y, Isemura M. Binding interaction between (−)-epigallocatechin gallate causes impaired spreading of cancer cells on fibrinogen. Biomed Res. 2013;34:301–308.10.2220/biomedres.34.301
- Li M, Hagerman E. Role of the flavan-3-ol and galloyl moieties in the interaction of (–)-epigallocatechin gallate with serum albumin. J Agric Food Chem. 2015;62:3768–3775.
- Ognjenović J, Stojadinović M, Milčić M, et al. Interactions of epigallo-catechin 3-gallate and ovalbumin, the major allergen of egg white. Food Chem. 2014;164:36–43.10.1016/j.foodchem.2014.05.005
- Cáceres-Mella A, Peña-Neira Á, Narváez-Bastias J, et al. Comparison of analytical methods for measuring proanthocyanidins in wines and their relationship with perceived astringency. Int J Food Sci Tech. 2013;48:2588–2594.10.1111/ijfs.2013.48.issue-12
- Kanoh N, Honda K, Simizu S, et al. Photo-cross-linked small-molecule affinity matrix for facilitating forward and reverse chemical genetics. Angew Chem Int Ed Engl. 2005;44:3559–3562.10.1002/(ISSN)1521-3773
- Tanaka T, Matsuo Y, Kouno I. Chemistry of secondary polyphenols produced during processing of tea and selected foods. Int J Mol Sci. 2009;11:14–40.10.3390/ijms11010014
- Johnson MC, Thomas AL, Greenlief CM. Impact of frozen storage on the anthocyanin and polyphenol content of American elderberry fruit juice. J Agric Food Chem. 2015;63:5653–5659.10.1021/acs.jafc.5b01702
- Delcour JA, Tuytens GM. Structure elucidation of three demeric proanthocyanidins isolated from a commercial Belgan pilsner beer. J Inst Brew. 1984;90:153–161.10.1002/jib.1984.90.issue-3
- Zhao J, Pang Y, Dixon RA. The mysteries of proanthocyanidin transport and polymerization. Plant Physiol. 2010;153:437–443.10.1104/pp.110.155432
- Liu Y, Shi Z, Maximova S, et al. Proanthocyanidin synthesis in Theobroma cacao: genes encoding anthocyanidin synthase, anthocyanidin reductase, and leucoanthocyanidin reductase. BMC Plant Biol. 2013;13:202.10.1186/1471-2229-13-202
- Wen PF, Ji W, Gao MY, et al. Accumulation of flavanols and expression of leucoanthocyanidin reductase induced by postharvest UV-C irradiation in grape berry. Genet Mol Res. 2015;14:7687–7695.10.4238/2015.July.13.14
- Kim YJ, Kim YB, Li X, et al. Accumulation of polyphenolpropanoids by white, blue, and red light irradiation and their organ-specific distribution in Chinese cabbage (Brassica rapa ssp. pekinensis). J Agric Food Chem. 2015;63:6772–6778.10.1021/acs.jafc.5b02086
- Sano A, Yamakoshi J, Tokutake S, et al. Procyanidin B1 is detected in human serum after intake of proanthocyanidin-rich grape seed extract. Biosci Biotech Biochem. 2003;67:1140–1143.10.1271/bbb.67.1140
- Bittner K, Kemme T, Peters K, et al. Systemic absorption and metabolism of dietary procyanidin B4 in pigs. Mol Nutr Food Res. 2014;58:2261–2273.10.1002/mnfr.201400435
- Kohri T, Matsumoto N, Tamakawa M, et al. Metabolic fate of (–)-[4-(3)H] epigallocatechin gallate in rats after oral administration. J Agric Food Chem. 2001;49:4202–4112.
- Kohri T, Suzuki M, Nanjo F. Identification of metabolites of (−)-epicatechin gallate and their metabolic fate in the rat. J Agric Food Chem. 2003;51:5561–5566.10.1021/jf034450x
- Takagaki A, Nanjo F. Metabolism of (−)-epigallocatechin gallate by rat intestinal flora. J Agric Food Chem. 2010;58:1313–1321.10.1021/jf903375s
- Takagaki A, Nanjo F. Catabolism of (+)-catechin and (−)-epicatechin by rat intestinal microbiota. J Agric Food Chem. 2013;61:4927–4935.10.1021/jf304431v
- Takagaki A, Nanjo F. Effects of metabolites produced from (−)-epigallocatechin gallate by rat intestinal bacteria on angiotensin I-converting enzyme activity and blood pressure in spontaneously hypertensive rats. J Agric Food Chem. 2015;63:8262–8266.10.1021/acs.jafc.5b03676
- Takagai A, Nanjo F. Biotransformation of (–)-epicatechin, (+)-epicatechin, (–)-catechin, and (+)-catechin by intestinal bacteria involved in isoflavone metabolism. Biosci Biotechnol Biochem. 2015;80:199–202.
- Ge Z, Dong X, Zhu W, et al. Metabolites and changes in antioxidant activity of A-type and B-type proanthocyanidin dimers after incubation with rat intestinal microbiota. J Agric Food Chem. 2015;63:8991–8998.10.1021/acs.jafc.5b03657