Abstract
6-Tuliposide B (PosB) is a glucose ester accumulated in tulip (Tulipa gesneriana) as a major secondary metabolite. PosB serves as the precursor of the antimicrobial lactone tulipalin B (PaB), which is formed by PosB-converting enzyme (TCEB). The gene TgTCEB1, encoding a TCEB, is transcribed in tulip pollen but scarcely transcribed in other tissues (e.g. roots) even though those tissues show high TCEB activity. This led to the prediction of the presence of a TCEB isozyme with distinct tissue specificity. Herein, we describe the identification of the TgTCEB-R gene from roots via native enzyme purification; this gene is a paralog of TgTCEB1. Recombinant enzyme characterization verified that TgTCEB-R encodes a TCEB. Moreover, TgTCEB-R was localized in tulip plastids, as found for pollen TgTCEB1. TgTCEB-R is transcribed almost exclusively in roots, indicating a tissue preference for the transcription of TCEB isozyme genes.
Graphical Abstract
Tissue-specific isozymes are involved in the conversion of 6-tuliposide B, a major secondary metabolite in tulip, to antibacterial tulipalin B.
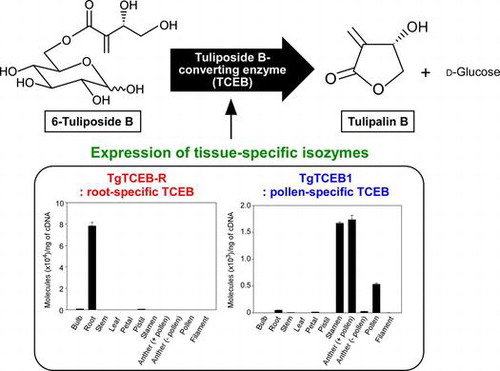
6-Tuliposides A (PosA) and B (PosB), the glucose esters of 4-hydroxy-2-methylenebutanoate and (3S)-3,4-dihydroxy-2-methylenebutanoate, respectively, are major secondary metabolites in tulip (Tulipa gesneriana) (Fig. ).Citation1–3) PosA and PosB serve as precursors of the corresponding lactonized aglycons, tulipalins A (PaA) and B (PaB), respectively (Fig. ).Citation2,4,5) Intact tulip tissues constitutively accumulate large amounts of Pos, whereas the biologically active PaA and PaB are far less abundant than Pos and are sometimes barely detectable.Citation3,4) PaA functions as an antifungal agent and PaB as an antibacterial agent;Citation1,2,4,6–14) thus, they are considered to be key secondary metabolites contributing to the chemical defense of tulip, while PosA and PosB function as storage forms of Pa.
Fig. 1. Reactions catalyzed by tuliposide-converting enzymes (TCEs).
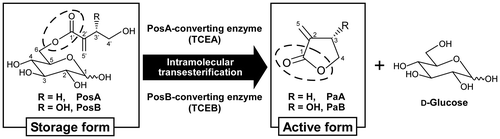
Although there is very little information about the biosynthetic pathway of Pos, the biochemical basis for the formation of Pa from Pos has been researched in our laboratory, and we discovered a novel Pos-converting enzyme (TCE) that specifically catalyzes this conversion reaction (Fig. ).Citation15–21) Although all parts of the tulip show TCE activity, the activity ratios toward PosA and PosB in crude extracts differ from tissue to tissue and correlate well with the ratios of PosA to PosB in each tissue. This was demonstrated to be attributable to the presence of two distinct types of TCE, a PosA-converting enzyme (TCEA) and a PosB-converting enzyme (TCEB), with the former preferentially catalyzing PosA conversion to PaA and the latter PosB conversion to PaB (Fig. ). Both types of TCE belong to the plant class I carboxylesterase family in the α/β-hydrolase fold superfamily.Citation21) Carboxylesterases are defined as catalyzing the hydrolysis of a carboxylic ester to form a carboxylic acid and an alcohol.Citation22) However, TCEs catalyze only intramolecular transesterification of Pos, which are glucose esters, to form Pa, which are lactones, and never catalyze hydrolysis. Hence, the TCEs were identified as unique non-ester-hydrolyzing carboxylesterases,Citation21) and, for this reason, TCE has been classified as a “lyase” (EC 4.2.99.22 for TCEA and EC 4.2.99.23 for TCEB).
The TgTCEA1 gene, encoding a TCEA, was isolated from the petals of tulip, and it is highly transcribed in petals.Citation17) However, the gene was not transcribed in bulbs, even though bulb tissue had the highest PosA-converting activity among the crude tissue extracts tested. It was therefore envisaged that a TCEA isozyme distinct from TgTCEA1 is predominantly expressed in bulbs. We subsequently succeeded in the purification of a TCEA isozyme from bulbs, the molecular mass and activity of which differed obviously from the petal enzyme. We also identified the corresponding TgTCEA-b1 gene from bulbs, which was functionally transcribed in bulbs but scarcely in petals, showing that there is a clear tissue preference for the transcription of the isozyme genes of TCEA.Citation18)
More recently, we identified the TgTCEB1 gene that encodes a TCEB from pollen grains of tulip via native enzyme purification.Citation20) TgTCEB1 was functionally transcribed in pollen, but only trace levels of transcription were observed in roots,Citation20) in which the second highest PosB-converting activity among crude extracts of tulip tissues has been detected.Citation17) This fact strongly suggests that the root TCEB is encoded by an isozyme gene different from TgTCEB1. Herein, to test this hypothesis, as well as to investigate the functional diversity of TCEB in tulip, we purified the native TCEB from roots and subsequently identified the corresponding gene, TgTCEB-R, which was highly homologous to TgTCEB1. TgTCEB-R shared some features with TgTCEB1, such as catalytic properties and subcellular localization, but a survey of the expression profiles clearly indicated tissue preferences for the transcription of the isozyme genes.
Materials and methods
Plant materials and growth conditions
All experiments were performed using tulip (T. gesneriana) cultivar “Murasakizuisho.” The bulbs were purchased from the Toyama Bulb Growers Association (Toyama, Japan). The bulbs were left in the dark at 15 °C for 2 weeks, and then at 4 °C for 8 weeks for vernalization prior to cultivation. The plants were cultivated hydroponically in a greenhouse at 20/15 °C (day/night) with a 12-h photoperiod, and roots were collected every 4 days from day-3 to day-51 (the day of flowering), and stored at −30 °C until use. For enzyme purification and cDNA cloning, roots collected from 17-d-old plants were used.
Chemicals
PosA, PosB, and PaB were prepared as described previously.Citation17,19) PaA (α-methylene-γ-butyrolactone) was purchased from Tokyo Chemical Industry.
Crude enzyme extraction
Lyophilized roots were ground to a fine powder with a mortar and pestle. The powders were then extracted with 100 mM potassium phosphate (KPi) buffer (pH 7.0), and the resulting extract was centrifuged at 15,000×g for 20 min at 4 °C. The supernatant was collected and passed through a PD-10 column (GE Healthcare), which had been equilibrated with the same buffer, and the eluent was used to measure the PosA- and PosB-converting activities according to the procedures described below in “Enzyme assay.”
Extraction and analysis of Pos/Pa content
Lyophilized roots were ground to a fine powder with a mortar and pestle. The powders were then extracted with 50% (v/v) methanol. After centrifugation (21,500×g, 10 min, 4 °C), the supernatant was appropriately diluted with water and subjected to reversed-phase HPLC analysis (column, TSKgel ODS-100 V, 5 μm, 4.6 × 150 mm, Tosoh [two columns were tandemly connected]; solvent, 5% [v/v] methanol/10 mM phosphoric acid; flow rate, 0.65 mL/min; detection, 208 nm).
Time course analysis of TCE activities and Pos/Pa content during root development
Hydroponic cultivation was started on November 19 2014, and the plants were grown until January 9 2015 (51 days after planting; DAP) in the conditions described in “Plant materials and growth conditions” (Fig. S1). Roots collected from three plants after each time period from 3 DAP to 51 DAP were pooled to average out biological errors, and stored at −30 °C until use. The stored roots were lyophilized for a day, and used for the measurement of Pos-converting enzyme activities and Pos/Pa content.
Purification of TCEB from roots
The PosB-converting activity of the crude extracts prepared from the frozen and lyophilized root samples was compared prior to enzyme purification. Given that the lyophilization did not affect the activity yield, the root samples were lyophilized prior to extraction, which made it much easier to implement the extraction step. All purification procedures were performed at 4 °C using KPi buffer (pH 7.0) unless otherwise noted. PosB- and PosA-converting activities were monitored throughout the purification steps. Approximately 250 g of 17-d-old roots were lyophilized for 2 d. The resulting lyophilized roots (16 g) were ground to a fine powder and extracted with a mortar and pestle in the presence of sea sand with 400 mL of 100 mM buffer. The resulting suspension was stirred for 1 h, filtered through cheesecloth, and centrifuged (9260×g, 30 min). The supernatant was fractionated with 30–60% saturated ammonium sulfate, and the resulting precipitate was dissolved in 100 mL of 100 mM buffer. Following dialysis against 100 mM buffer, the solution was brought to 30% ammonium sulfate saturation and applied to a Butyl-Toyopearl column (1.5 × 9 cm, Tosoh) that had been equilibrated with 100 mM buffer containing 30% saturated ammonium sulfate. After washing the column with 75 mL of the same buffer, the column was subsequently eluted with a linear gradient of ammonium sulfate (30–0% saturation) in 100 mM buffer (75 mL for each buffer; 3-mL fractions collected). The active fractions were combined (six fractions, 18 mL), dialyzed against 20 mM buffer, and loaded onto a DEAE-Toyopearl column (1.0 × 6.8 cm, Tosoh) equilibrated with the same buffer. After washing the column with 25 mL of the same buffer, the column was eluted with a linear gradient of NaCl (0–500 mM) in 20 mM buffer (25 mL for each buffer; 1.7 mL fractions). The active fractions were combined (two fractions, 3.4 mL) and the buffer was exchanged to 5 mM (pH 6.0) by passing through a PD-10 column equilibrated with the same buffer. The solution was loaded onto a CFT Ceramic Fluoroapatite column (0.8 × 4.7 cm, Bio-Rad) equilibrated with the same buffer. After washing the column with 10 mL of the same buffer, the column was eluted with a linear gradient of buffer (5–500 mM, pH 6.0; 10 mL for each buffer; 0.4 mL fractions). The active fractions were combined (five fractions, 2 mL), concentrated by ultrafiltration with an Amicon Ultra-2 (10 kDa) (Merck Millipore), and subjected to gel filtration chromatography on a Superdex 200 column (GE Healthcare) equilibrated with 50 mM buffer containing 150 mM NaCl at 0.5 mL/min. The active fractions were combined to give purified TCEB and stored at 4 °C.
Enzyme assay
A standard enzyme assay was performed using 4 mM PosA or PosB as substrate and the reaction products were detected by HPLC analysis as described previously.Citation17) The effects of pH and temperature on the activity and stability of the enzyme and the effects of various compounds on the enzyme activity were examined according to the methods described by Nomura et al.Citation20) Enzyme reactions for the determination of the kinetic parameters for PosA and PosB were performed as described previously.Citation17) Kinetic parameters were calculated by nonlinear fitting of the data to the Michaelis-Menten equation using SigmaPlot 12.5 (Systat Software).
Protein analysis
Protein concentrations were determined with a Protein Assay Kit (Bio-Rad) using a bovine serum albumin standard. The molecular masses of the denatured and native forms of the enzyme were estimated by SDS-PAGE and gel filtration analyses, respectively. The gel filtration analysis on a Superdex 200 column was performed in the same conditions as described above in “Purification of TCEB from roots” using Gel Filtration Calibration Kit HMW (GE Healthcare) as molecular size markers. The presence or absence of sugar chains was analyzed in SDS-PAGE gels with a Glycoprotein Detection Kit (Sigma) using horseradish peroxidase (Sigma) as a positive control. For N-terminal amino acid sequencing, the TCEB purified from roots was subjected to SDS-PAGE, and then transferred electrophoretically onto a polyvinylidene difluoride membrane (0.22 μm, ATTO). After Coomassie Brilliant Blue (CBB) staining, a 45-kDa band was cut out from the blot and subjected to automatic Edman degradation on a peptide sequencer, which was performed by a professional service (APRO Life Science Institute). For the prediction of internal peptide sequences, the CBB-stained 45-kDa band was subjected to in-gel digestion with trypsin, and the digests were analyzed by LC-MS/MS by APRO Life Science Institute.
Cloning of TgTCEB-R gene
Total RNA was isolated from 17-d-old roots using a NucleoSpin RNA Plant Kit (Macherey-Nagel). Poly(A) RNA was purified from DNase I-treated total RNA using a MicroPoly(A) Purist Kit (Ambion). cDNA was synthesized with a SMARTer RACE cDNA Amplification Kit (Clontech) and subsequently used as a PCR template with degenerate primers. The primers were designed based on two internal peptide sequences of the purified TCEB: the forward (rTCEB-deg-F) and reverse (rTCEB-deg-R) primers were designed from peptide sequences ANILIVSVNYR and NIEGIALVHPYFFGK, respectively (Table S1). The PCR reaction was performed as follows: 2 min at 94 °C, followed by 35 cycles of amplification (30 s at 94 °C, 1 min at 50 °C, and 1 min at 72 °C) in a 20-μL reaction mixture containing 4 ng of cDNA, 0.5 μM primers, 0.2 mM dNTPs, 1 × reaction buffer and 0.5 U of Blend Taq DNA polymerase (Toyobo). The PCR products (around 300 bp) were cloned into vector pCR2.1-TOPO-TA (Invitrogen) and sequenced, resulting in the isolation of a 275-bp fragment. To isolate the missing parts from the 5′- and 3′- ends of the gene, 5′- and 3′-RACE-PCR experiments were performed using the cDNAs synthesized above as templates. The 5′-RACE was performed with the gene-specific primer rTCEB-5RACE-R1 (Table S1) and an adaptor primer as follows: 30 s at 98 °C, followed by 30 cycles of amplification (10 s at 98 °C, 30 s at 68 °C, and 30 s at 72 °C) in a 20-μL reaction mixture containing 4 ng of cDNA, 1 × Universal Primer A Mix, 0.5 μM rTCEB-5RACE-R1 primer, 0.2 mM dNTPs, 1 × reaction buffer and 0.4 U of Phusion Hot Start II High-Fidelity DNA Polymerase (Thermo Scientific). The second 5′-RACE-PCR was performed with the gene-specific primer rTCEB-5RACE-R2 (Table S1) and a nested adaptor primer as follows: 30 s at 98 °C, followed by 30 cycles of amplification (10 s at 98 °C and 1 min at 72 °C) in a 50-μL reaction mixture containing 1 μL of the first PCR reaction mixture, 0.5 μM Nested Universal Primer A, 0.5 μM rTCEB-5RACE-R2 primer, 0.2 mM dNTPs, 1 × reaction buffer and 1 U of Phusion Hot Start II High-Fidelity DNA Polymerase. The first PCR for the 3′-RACE was performed in the same conditions as those used for the first 5′-RACE-PCR, except for the use of the gene-specific primer rTCEB-3RACE-F1 (Table S1). The second 3′-RACE-PCR was performed in the same conditions as those used for the second 5′-RACE-PCR, except for the use of the gene-specific primer rTCEB-3RACE-F2 (Table S1). The 5′- and 3′-RACE products were then cloned into vector pCR-Blunt II-TOPO (Invitrogen) and sequenced.
Finally, the entire coding region of the TgTCEB-R cDNA was obtained by PCR using root cDNA (synthesized above for 5′-RACE) as the template with rTCEB-full-F and rTCEB-full-R primers (Table S1), which were designed from the untranslated 5′- and 3′- regions, respectively. The reaction was performed as follows: 30 s at 98 °C, followed by 35 cycles of amplification (10 s at 98 °C, 30 s at 55 °C, and 1 min at 72 °C) in a 50-μL reaction mixture containing 4 ng of cDNA, 0.5 μM primers, 0.2 mM dNTPs, 1 × reaction buffer, and 1 U of Phusion Hot Start II High-Fidelity DNA Polymerase. The PCR products were cloned into pCR-Blunt II-TOPO and sequenced.
For the isolation of the genomic TgTCEB-R sequence, PCR was performed in the same conditions as those used for the RT-PCR to obtain full-length TgTCEB-R cDNA, except for the use of 50 ng of genomic DNA as the template. The PCR products were cloned into pCR-Blunt II-TOPO and sequenced.
Expression and purification of recombinant enzyme
Full-length TgTCEB-R cDNA was used as the template for PCR to prepare a DNA fragment corresponding to the mature TgTCEB-R enzyme, whose N-terminus started from residue Leu-63 (Fig. S2). The DNA fragment amplified with primers rTCEB-fus-F and rTCEB-fus-R (Table S1) was inserted into the NdeI site of vector pET28a (Novagen) with an In-Fusion HD Cloning Kit (Clontech). The resulting plasmid was introduced into Escherichia coli strain Rosetta 2 (DE3) (Novagen) for expression of the N-terminally His-tagged enzyme.
Culturing of the recombinant E. coli, induction of enzyme expression, and the extraction and purification of the His-tagged recombinant enzyme, were performed as described previously.Citation17) For removal of 17 vector-derived amino acid residues, including the His-tag, the metal affinity-purified enzyme was treated with thrombin (Sigma) (1.5 U/mg recombinant enzyme) overnight at 4 °C. After exchanging the buffer to 10 mM KPi (pH 7.0) by passing through a PD-10 column equilibrated with the same buffer, the enzyme solution was applied to a RESOURCE Q column (1 mL, GE Healthcare) equilibrated with 10 mM KPi buffer (pH 7.0), and the column was eluted in gradient mode at 2 mL/min as follows: buffer A, 10 mM KPi (pH 7.0); buffer B, 50 mM KPi (pH 7.0) containing 1 M NaCl; 0% B (0–3 column volumes; CV), and 0–15% B (3–33 CV). The active fractions were collected, concentrated by ultrafiltration with an Amicon Ultra-15 (10 kDa) (Merck Millipore), and subjected to gel-filtration chromatography on a Superdex 200 column equilibrated with 50 mM HEPES buffer (pH 7.5) containing 150 mM NaCl at 0.5 mL/min. The active fractions were combined and stored at 4 °C.
Quantification of transcript levels
Total RNA samples were isolated as described by Nomura et al.Citation20) from each part of plants at the flowering stage. After treatment with DNase I and repurification of the RNA using a NucleoSpin RNA Plant Kit, cDNA was synthesized with oligo(dT)20 primer using SuperScript III reverse transcriptase (Invitrogen), for which 1 μg of RNA was used as the template in 20 μL of reaction mixture. Quantitative PCR (qPCR) for the TgTCEB-R gene was performed with primers rTCEB-qRT-F and rTCEB-qRT-R (Table S1), which were designed to specifically amplify a 153-bp amplicon from the TgTCEB-R gene but not TgTCEB1. The reaction was performed in triplicate in a 7500 Real-Time PCR System (Applied Biosystems) in the following conditions: 30 s at 95 °C followed by 40 cycles of amplification (5 s at 95 °C and 34 s at 64 °C) in a 20-μL reaction mixture containing 10 ng of template cDNA, 0.2 μM primers, 0.4 μL of ROX Reference Dye II (Takara Bio) and 10 μL of SYBR Premix Ex Taq (Takara Bio). A standard curve was made using a dilution series of known quantities of plasmid containing TgTCEB-R cDNA as the template. The specificity of the amplification was verified by a melt-curve analysis and agarose gel electrophoresis.
Functional analysis of the transit peptide of TgTCEB-R
The coding sequence of the putative transit peptide (TP) at the N-terminus of TgTCEB-R (residues 1–62; TP62) was amplified by PCR using plasmid containing TgTCEB-R cDNA as the template with primers rTCEB-rep-F and rTCEB-rep-R (Table S1). The resulting fragment was inserted into the NcoI site of the GFP expression plasmid pCaMV35Somega-sGFP(S65T)-nos3′Citation23) with an In-Fusion HD Cloning Kit to give a plasmid capable of expressing the TgTCEB-R(TP62)-GFP fusion protein. Transient expression of the fusion protein in onion (Allium cepa) epidermal cells and microscopic analysis were performed as described previously.Citation17)
Results and discussion
Time-dependent changes in TCE activity and Pos/Pa content during root development
Prior to the purification of the TCEB from roots, the PosA- and PosB-converting activities and the Pos/Pa content were measured every 4 days from 3 DAP to 51 DAP, for which hydroponic cultivation, rather than soil cultivation, was adopted to keep the roots as intact as possible. As Fig. (A) shows, PosB-converting activity was significantly higher than PosA-converting activity throughout the cultivation period. The highest activity was detected at 3 DAP (approximately 770 U/g dry weight; DW), after which the activity declined to approximately 270 U/g DW over a week; a similar level was maintained until 51 DAP. At 51 DAP, the PosB-converting activity (320 U/g DW) was approximately eightfold higher than the PosA-converting activity (41 U/g DW), in good agreement with our previous data.Citation17) The time course profiles of Pos and Pa content (Fig. (B)) were similar to those of the Pos-converting activities, and PosB was found to be the major type of Pos in roots, except that the PosB content at 3 DAP was similar to the PosA content. These results confirm our previous observation that the ratios of the PosA- and PosB-converting activities correlate well with the ratios of PosA and PosB content in each tissue.Citation15–17,20)
Fig. 2. Changes in the TCE activities and Pos/Pa content in roots during cultivation.
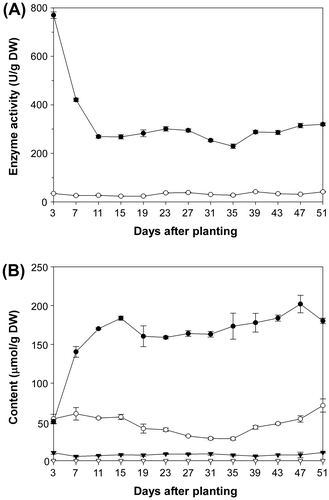
Purification of TCEB from roots
Although the highest PosB-converting activity was detected at 3 DAP, the quantity of roots was limited at such an early growth stage (Fig. S1) and it was laborious to collect enough root samples for enzyme purification. Thus, the roots collected from 17-d-old plants were used for enzyme purification. The crude extract prepared from 16 g DW (equivalent to 250-g fresh weight) of roots was first fractionated by ammonium sulfate precipitation, and, subsequently, through four steps of column chromatography, the TCEB was purified 119-fold to homogeneity with an overall yield of 1.42% (Table ; Fig. ). On purification of TCEB from the pollen grains of tulip, three activity peaks appeared separately on column chromatography, which were individually purified as isoforms 1, 2, and 3Citation20); however, only a single activity peak was detected throughout the purification process of root TCEB.
Table 1. Purification of the TCEB from tulip roots.
Fig. 3. Purification of the TCEB from roots.
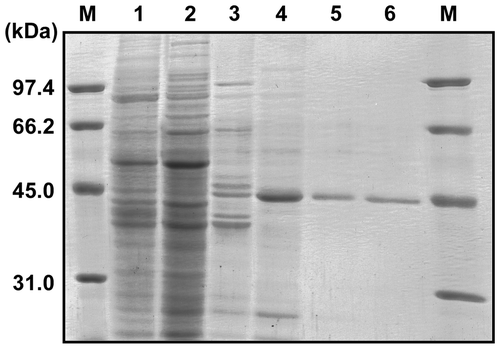
Enzymatic properties of root TCEB
The purified root TCEB appeared as a 45-kDa band by SDS-PAGE analysis (Fig. ), and the native molecular mass was estimated to be 100 kDa by gel filtration analysis, indicating that the enzyme exists as a dimer in its native form, as found for other TCEs.Citation17,18,20) The SDS-PAGE gel of the enzyme was subjected to sugar chain staining using the periodate–fuchsin method, but no staining was observed, which indicated that the root TCEB is not glycoprotein. The native and subunit molecular masses of the pollen TCEBs were 47 kDa and 92–99 kDa, respectively; the subunit masses were clearly different between the pollen and the root enzymes, which strongly suggests that the root TCEB is a distinct isozyme from the pollen TCEBs.
The purified root TCEB catalyzed the conversion of PosB to PaB with a specific activity of 272 U/mg at 4 mM PosB, whereas its activity toward 4 mM PosA was 4.62 U/mg, 1.7% of that toward PosB. These values were comparable with the activities of the TCEBs purified from pollen grains, indicating that PosB is the natural substrate for the root enzyme.
The temperature and pH optima of the root TCEB were determined to be 35–40 °C and pH 6.5–7.5. The enzyme was stable at temperatures up to 35 °C for 30 min at pH 7.0, and at pH 5.5–10.0 for 30 min at room temperature. The effects of 51 compounds on enzyme activity were tested (Table S2). The addition of the serine enzyme inhibitor phenylmethanesulfonyl fluoride (PMSF; 2 mM) inhibited the activity by 89%. Among the metal ions tested, Ag+, Cu2+, Fe2+, Fe3+, Hg2+, Sn2+, and V3+ notably lowered the activity, of which Cu2+ and Hg2+ exhibited the strongest inhibitory effects. These enzymatic properties were similar to those of the pollen TCEBs reported previously.Citation20)
The kinetic parameters of the root TCEB were determined for PosB and PosA (Table ). The Km and Vmax values for PosB were 3.3 mM and 680 U/mg, respectively, which gave a catalytic efficiency (Vmax/Km) of 210 U/mg/mM. The Km and Vmax values for PosA were 6.3 mM and 12 U/mg, from which the Vmax/Km value was calculated to be 1.9 U/mg/mM. Thus, the catalytic efficiency ratio for PosB and PosA (B/A) was 110, which was similar to those of the pollen TCEBs (ratios 130–150) (Table ; representative data for pollen isoform 1 is listed),Citation20) verifying again that PosB is the preferred substrate for the root TCEB.
Table 2. Kinetic parameters of the TCEBs purified from roots and pollen grains.
Cloning of TgTCEB-R cDNA from roots
N-Terminal sequencing of the root TCEB resulted in the determination of the first 10 amino acids to be LSPSPTPLDV, which was identical to the N-terminal sequence of the pollen TCEB isoform 1.Citation20) In addition, LC-MS/MS analysis of in-gel digests of the enzyme allowed the prediction of five peptide sequences, including TDYVEASLDPATEVTSR (sequence 1; seq-1), DAITDTGVPVR (seq-2), VDDSPPNSLR (seq-3), ANILIVSVNYR (seq-4), and NIEGIALVHPYFFGK (seq-5).
To amplify a fragment of cDNA encoding root TCEB, degenerate primers were designed from seq-4 and seq-5. Reverse transcription (RT)-PCR from root mRNA resulted in the amplification of a 275-bp cDNA fragment. Its missing 5′- and 3′-parts were amplified by 5′- and 3′-RACE-PCR, and, subsequently, RT-PCR was performed with primers in the 5′- and 3′-untranslated regions, which resulted in the isolation of the full-length cDNA, TgTCEB-R (“R” for root; GenBank accession No. LC205737).
Genomic PCR with the primers used in the cloning of the full-length TgTCEB-R cDNA resulted in the isolation of a genomic sequence corresponding to the TgTCEB-R cDNA, and a comparison of the cDNA and genomic sequences revealed that TgTCEB-R is an intronless gene, like other TgTCEA and TgTCEB genes.Citation17,18,20)
TgTCEB-R encoded a polypeptide of 437 amino acids (Fig. S2) that contains partial sequences identified by the analysis of the TCEB purified from roots, suggesting that TgTCEB-R cDNA encodes root TCEB. The TgTCEB-R polypeptide was different (80% identity) from the 440 amino acid-long TgTCEB1 polypeptide identified from pollen grains (Fig. S2).Citation20) A comparison of the full-length TgTCEB-R polypeptide with the N-terminus of the TCEB purified from roots confirmed that TgTCEB-R had been translated as a precursor polypeptide before being processed to a mature polypeptide by cleavage of a 62 amino acid-long N-terminal TP, like other TgTCEA and TgTCEB polypeptides identified so far.Citation17,18,20) The TgTCEB-R polypeptide possessed typical sequence motifs for class I carboxylesterases of plants, including the HGG-motif (residues 148–150), the two Gly residues of which are involved in the formation of an oxyanion hole structure during the formation of the acyl–enzyme complex, and catalytic triad residues Ser, Asp, and His (residues 232, 325, and 357, respectively), of which the catalytic Ser is present within the conserved sequence Gly–X–Ser–X–Gly (Fig. S2).Citation22,24) Phylogenetic analysis of TgTCEB-R together with selected members of plant carboxylesterase family, including TgTCEA1, TgTCEA-b1, and TgTCEB1, and related proteins in α/β-hydrolase fold superfamily showed a close relationship of TgTCEB-R to TgTCEB1 rather than to TgTCEAs, indicating the paralogous relationships between TgTCEA1 and TgTCEA-b1 and between TgTCEB1 and TgTCEB-R (Fig. S3).
Enzymatic properties of recombinant TgTCEB-R
Mature TgTCEB-R polypeptide (residues 63–437) was efficiently expressed as an N-terminally His-tagged protein in a soluble protein fraction in E. coli strain Rosetta 2 (DE3). The His-tagged enzyme was purified by metal-affinity chromatography, and, following the removal of the His-tag-containing 17 amino acid residues by thrombin digestion, the His-tag-free enzyme was purified to homogeneity by strong anion-exchange and gel-filtration chromatographies in a yield of approximately 6 mg from 500 mL of E. coli culture. Enzyme homogeneity and removal of the His-tag-containing fragment were confirmed by SDS-PAGE (Fig. S4). A dimeric nature of the enzyme, like the native TCEB purified from tulip roots, was also confirmed by SDS-PAGE and gel filtration analyses.
The specific activities of the purified His-tag-truncated recombinant TgTCEB-R toward PosB and PosA at 4 mM were 541 U/mg and 6.14 U/mg, respectively, which were approximately double of those of the native TCEB purified from roots, implying that the native root enzyme might be partially inactivated during the purification process. The kinetic parameters of the His-tag-truncated enzyme determined for PosB and PosA are listed in Table with those of the recombinant TgTCEB1 identified previously from pollen grains.Citation20) The catalytic efficiency (kcat/Km) of TgTCEB-R for PosB (230 /s/mM) was 88-fold higher than that for PosA (2.6 /s/mM), which was comparable with the catalytic efficiency ratio (for PosB/PosA) of 110 of the native TCEB purified from roots. No significant differences in the kinetic parameters were observed between the recombinant TgTCEB-R and TgTCEB1 enzymes derived from roots and pollen, respectively (Table ).
Table 3. Kinetic parameters of recombinant TgTCEB enzymes.
Transcript profile of TgTCEB-R in tulip tissues
The transcript levels of the TgTCEB-R gene were examined in different tulip tissues by RT-qPCR analysis with primers specific to TgTCEB-R. As Fig. shows, the TgTCEB-R gene was transcribed almost exclusively in roots. The profiles were totally different from those of the TgTCEB1 gene that was isolated from pollen grainsCitation20); transcription of TgTCEB1 was observed in stamens, anthers (with pollen), and pollen grains, but not in filaments and anthers stripped of pollen, demonstrating that the transcription of TgTCEB1 occurs specifically in pollen grains (Fig. , inset).
Fig. 4. Transcript levels of the TgTCEB-R gene in tulip tissues analyzed by RT-qPCR.
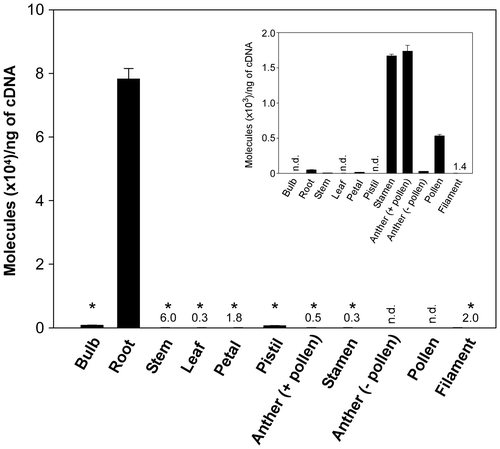
Given all the results in the present study, it was clearly demonstrated that TgTCEB-R is the gene responsible for encoding a root-specific TCEB isozyme, and there is a clear tissue preference in the transcription of the TgTCEB-R and TgTCEB1 isozyme-encoding genes, as was also found for the TgTCEA1 and TgTCEA-b1 isozyme genes that were isolated from petals and bulbs, respectively.Citation17,18) It will be of great interest to examine the molecular basis of the regulation of the tissue-specific transcription of those isozyme genes, for which analysis of the promoter region of each isozyme gene will give important clues.
Furthermore, the results shown here raise another issue to be resolved. TgTCEB-R and TgTCEB1 were hardly transcribed in tulip leaves (Fig. ). However, crude extracts of leaf tissue exhibit high PosB-converting activity.Citation17) This allows us to predict the presence of another TCEB isozyme that is specifically expressed in leaves; the purification and molecular identification of this enzyme are in progress and will be published elsewhere.
Subcellular localization of TgTCEB-R enzyme
The function of the 62 amino acid-long N-terminal TP (TP62) of TgTCEB-R was examined by expressing it as a GFP-fused protein in onion epidermal cells. Expression of the TgTCEB-R(TP62)–GFP fusion protein produced a fluorescence signal localized to dotted organelles (Fig. ). The size and shape of the signal pattern matched well with those of WxTP-DsRed, expressing the plastid-targeted TP of Waxy protein fused to the red fluorescence protein DsRed,Citation25) which we have used as a positive control for plastidial localization,Citation17,18,20) and with the characteristic features of plastids.Citation26,27) These results indicated that the N-terminal TP of TgTCEB-R functions as a plastid-targeting signal, and the mature polypeptide of TgTCEB-R is localized in plastids, like TgTCEB1.Citation20) This conclusion is reasonable because there is high sequence similarity between the TPs of TgTCEB-R and TgTCEB1; only 13 amino acids (of 62) differ (Fig. S2). Given that all the TgTCEA and TgTCEB polypeptides identified so far are localized to plastids, plastidial localization must be a feature common to the TCEs in tulip and it allows for accumulation of subcellularly compartmentalized Pos at high levels in intact plants, which can immediately be converted to the biologically active Pa upon cell disruption caused by pathogen infection and herbivore predation, using the TCEs as ignition enzymes.Citation21)
Fig. 5. Subcellular localization of TgTCEB-R.
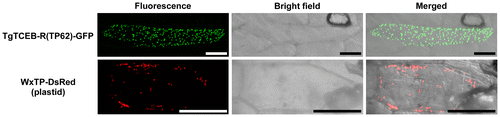
It has now been demonstrated that there are isozymes of both TCEA and TCEB (Table ). Although the reason for the presence of isozymes with different tissue preferences is yet to be elucidated, considering that plastids differentiate into several forms with distinct functions, such as chloroplasts and amyloplasts,Citation28) it is likely that expression of each isozyme is transcriptionally regulated so as to allocate the isozyme that is most suitable to the physiological environment of each type of plastid. Marked differences in enzyme characteristics have not been observed between the isozymes in conventional enzyme assay conditions, but such differences may exist in planta.
Table 4. TCEA and TCEB isozymes identified so far.
Conclusions
We identified the root-specific TCEB isozyme, which is responsible for the conversion of PosB, a major constitutive secondary metabolite in tulip, to biologically active PaB. Biochemical and molecular characterization of the enzyme clearly demonstrated that distinct TCEB isozymes are expressed almost exclusively to each other in roots and pollen, from which a TCEB was originally isolated, respectively. Furthermore, the presence of another TCEB isozyme specifically expressed in leaves is suggested by our data. Such isozyme tissue preference is also a feature of TCEAs, the enzyme catalyzing the conversion of PosA to PaA. The fact that all the TCEs identified so far, including the root TCEB discovered in the present study, are plastidial enzymes may provide some clues as to why the isozymes are tissue-specific. That is, each isozyme evolved to adapt to the distinct physiological conditions of the distinct forms of plastids in each tissue.
Author contribution
TN and YK designed research. TN and AU performed research. TN, AU, SO, and YK analyzed data. TN wrote the paper.
Disclosure statement
No potential conflict of interest was reported by the authors.
Funding
This work was supported by JSPS KAKENHI [grant number 23780120], [grant number 26850072].
Supplemental materials
The supplemental material for this paper is available at http://dx.doi.org/10.1080/09168451.2017.1295806
TBBB_1295806_Supplementary_information.doc
Download MS Word (4.6 MB)References
- Tschesche R, Kämmerer F-J, Wulff G, et al. Über die antibiotisch wirksamen der tulpe (Tulipa gesneriana) [On the antibiotically active substances of the tulip (Tulipa gesneriana)] Tetrahedron Lett. 1968;9:701–706.10.1016/S0040-4039(00)75615-2
- Tschesche R, Kämmerer F-J, Wulff G. Über die struktur der antibiotisch aktiven substanzen der tulpe (Tulipa gesneriana L.) [On the structure of antibiotically active substances of the tulip (Tulipa gesneriana L.)]. Chem Ber. 1969;102:2057–2071.10.1002/(ISSN)1099-0682
- Christensen LP, Kristiansen K. Isolation and quantification of tuliposides and tulipalins in tulips (Tulipa) by high-performance liquid chromatography. Contact Dermat. 1999;40:300–309.10.1111/cod.1999.40.issue-6
- Beijersbergen JCM, Lemmers CBG. Enzymic and non-enzymic liberation of tulipalin A (α-methylene butyrolactone) in extracts of tulip. Physiol Plant Pathol. 1972;2:265–270.
- van Rossum MWPC, Alberda M, van der Plas LHM. Tulipaline and tuliposide in cultured explants of tulip bulb scales. Phytochemistry. 1998;49:723–729.10.1016/S0031-9422(98)00199-X
- Bergman BHH, Beijersbergen JCM. A fungitoxic substance extracted from tulips and its possible role as a protectant against disease. Neth J Plant Pathol. 1968;74:157–162.10.1007/BF01977525
- Shoji K, Ubukata M, Momonoi K, et al. Anther-specific production of antimicrobial tuliposide B in tulips. J Jpn Soc Hort Sci. 2005;74:469–475.10.2503/jjshs.74.469
- Bergman BHH, Beijersbergen JCM, Overeem JC, et al. Isolation and identification of α-methylenebutyrolactone, a fungitoxic substance from tulips. Recl Trav Chim Pays-Bas. 1967;86:709–714.
- Beijersbergen JCM. A method for determination of tulipalin A and B concentrations in crude extracts of tulip tissues. Recl Trav Chim Pays-Bas. 1972;91:1193–1200.
- Schönbeck F, Schroeder C. Role of antimicrobial substances (tuliposides) in tulips attacked by Botrytis spp. Physiol Plant Pathol. 1972;2:91–99.10.1016/0048-4059(72)90015-X
- Mendgen T, Scholz T, Klein CD. Structure-activity relationships of tulipalines, tuliposides, and related compounds as inhibitors of MurA. Bioorg Med Chem Lett. 2010;20:5757–5762.10.1016/j.bmcl.2010.07.139
- Shigetomi K, Shoji K, Mitsuhashi S, et al. The antibacterial properties of 6-tuliposide B. Synthesis of 6-tuliposide B analogues and structure–activity relationship. Phytochemistry. 2010;71:312–324.10.1016/j.phytochem.2009.10.008
- Shigetomi K, Omoto S, Kato Y, et al. Asymmetric total synthesis of 6-tuliposide B and its biological activities against tulip pathogenic fungi. Biosci Biotechnol Biochem. 2011;75:718–722.10.1271/bbb.100845
- Shigetomi K, Olesen SH, Yang Y, et al. MurA as a primary target of tulipalin B and 6-tuliposide B. Biosci Biotechnol Biochem. 2013;77:2517–2519.10.1271/bbb.130663
- Kato Y, Shoji K, Ubukata M, et al. Purification and characterization of a tuliposide-converting enzyme from bulbs of Tulipa gesneriana. Biosci Biotechnol Biochem. 2009;73:1895–1897.10.1271/bbb.90226
- Kato Y, Yoshida H, Shoji K, et al. A facile method for the preparation of α-methylene-γ-butyrolactones from tulip tissues by enzyme-mediated conversion. Tetrahedron Lett. 2009;50:4751–4753.10.1016/j.tetlet.2009.06.018
- Nomura T, Ogita S, Kato Y. A novel lactone-forming carboxylesterase: molecular identification of a tuliposide A-converting enzyme in tulip. Plant Physiol. 2012;159:565–578.10.1104/pp.112.195388
- Nomura T, Tsuchigami A, Ogita S, et al. Molecular diversity of tuliposide A-converting enzyme in the tulip. Biosci Biotechnol Biochem. 2013;77:1042–1048.10.1271/bbb.130021
- Nomura T, Hayashi E, Kawakami S, et al. Environmentally benign process for the preparation of antimicrobial α-methylene-β-hydroxy-γ-butyrolactone (tulipalin B) from tulip biomass. Biosci Biotechnol Biochem. 2015;79:25–35.10.1080/09168451.2014.946395
- Nomura T, Murase T, Ogita S, et al. Molecular identification of tuliposide B-converting enzyme: a lactone-forming carboxylesterase from the pollen of tulip. Plant J. 2015;83:252–262.10.1111/tpj.12883
- Nomura T. Function and application of a non-ester-hydrolyzing carboxylesterase discovered in tulip. Biosci Biotechnol Biochem. 2017;81:81–94.10.1080/09168451.2016.1240608
- Gershater MC, Edwards R. Regulating biological activity in plants with carboxylesterases. Plant Sci. 2007;173:579–588.10.1016/j.plantsci.2007.08.008
- Niwa Y. A synthetic green fluorescent protein gene for plant biotechnology. Plant Biotechnol. 2003;20:1–11.10.5511/plantbiotechnology.20.1
- Ileperuma NR, Marshall SD, Squire CJ, et al. High-resolution crystal structure of plant carboxylesterase AeCXE1, from Actinidia eriantha, and its complex with a high-affinity inhibitor paraoxon. Biochemistry. 2007;46:1851–1859.10.1021/bi062046w
- Kitajima A, Asatsuma S, Okada H, et al. The rice α-amylase glycoprotein is targeted from the Golgi apparatus through the secretory pathway to the plastids. Plant Cell. 2009;21:2844–2858.10.1105/tpc.109.068288
- Natesan SKA, Sullivan JA, Gray JC. Stromules: a characteristic cell-specific feature of plastid morphology. J Exp Bot. 2005;56:787–797.10.1093/jxb/eri088
- Nelson BK, Cai X, Nebenführ A. A multicolored set of in vivo organelle markers for co-localization studies in Arabidopsis and other plants. Plant J. 2007;51:1126–1136.10.1111/j.1365-313X.2007.03212.x
- Wise RR. The diversity of plastid form and function. In: Wise RR, Hoober JK, editors. The structure and function of plastids. Dordrecht: Springer; 2006. p. 3–26.10.1007/978-1-4020-4061-0