Abstract
We investigated the anti-inflammatory effects of 3α-hydroxy-lup-20(29)-en-23, 28-dioic acid (HLEDA)—a lupane-type triterpene isolated from leaves of Acanthopanax gracilistylus W. W.Smith (AGS), as well as the underlying molecular mechanisms in lipopolysaccharide (LPS)-induced RAW264.7 cells. Our results demonstrated that HLEDA concentration-dependently reduced the production of nitric oxide (NO), significantly suppressed LPS-induced expression of TNF-α and IL-1β at the mRNA and protein levels in RAW264.7 cells. Further analysis revealed that HLEDA could reduce the secretion of High Mobility Group Box 1 (HMGB1). Additionally, the results showed that HLEDA efficiently decreased nuclear factor-kappaB (NF-κB) activation by inhibiting the degradation and phosphorylation of IκBα. These results suggest that HLEDA exerts anti-inflammatory properties in LPS-induced macrophages, possibly through inhibition of the NF-κB signaling pathway, which mediates the expression of pro-inflammatory cytokines. These results warrant further studies that would concern candidate therapy for diseases, such as fulminant hepatitis and rheumatology of triterpenoids in AGS.
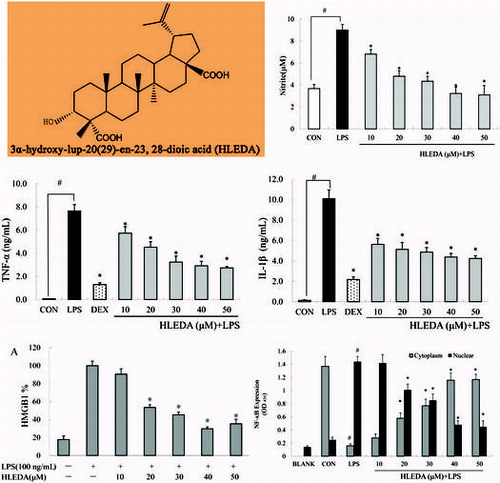
Inflammation is the defensive response of living tissue to infection or injury.Citation1) Stimulated inflammatory cells produce pro-inflammatory mediators, such as nitric oxide (NO), tumor necrosis factor-α (TNF-α), and interleukin-1β (IL-1β) and trigger the cascades responsible for the inflammatory response. However, overproduction of proinflammatory mediators induces systemic inflammatory diseases, such as allergy, arthritis, asthma, multiple sclerosis, atherosclerosis, and Alzheimer’s disease (AD).Citation2–6) For example, the overproduction of TNF-α will cause metabolic disorder, tissue injury, and septic shock by the induced body fever or inflammatory reactions.Citation7,8) Accordingly, it has been suggested that the inhibition of these inflammatory mediators might provide a useful therapeutic strategy for the treatment of inflammatory diseases.Citation9)
High mobility group box 1 (HMGB1) is a protein that has been shown to be released by activated monocytes or macrophages, as well damaged and necrotic cells.Citation10) Within the nucleus, HMGB1 plays an important role in the regulation of gene transcription.Citation11) Upon release by phagocytes and damaged/necrotic cells,Citation12–14) extracellular HMGB1 plays a critical role in the initiation of inflammation, can activate macrophages and upregulate the expression of cytokines, such as TNF-α and IL-1β,Citation15) and contributes to the pathogenesis of various inflammatory diseases.Citation16,17) As a late mediator of inflammation, HMGB1 is released days after endotoxin exposure.Citation18) HMGB1 amplifies the inflammatory response by stimulating the release of various proinflammatory cytokines in several kinds of cells, such as macrophages and monocytes.Citation19) Given the critical role of HMGB1 in the process of inflammation, HMGB1 represents an attractive drug target for the clinical treatment of inflammatory diseases.Citation20)
Macrophages are widely distributed in the body and play a critical role in the immune system by providing an immediate defense against foreign agents.Citation21) Activated macrophages produce inflammatory mediator nitric oxide (NO), prostanoids, as well as proinflammatory cytokines, such as tumor necrosis factor-α (TNF-α), interleukin 1-β (IL1-β)Citation22), and “late-phase” pro-inflammatory cytokine High-mobility group 1 (HMGB-1).Citation23) Lipopolysaccharide (LPS) is the principal component of the outer membrane of Gram-negative bacteria, and is one of the most powerful known activators of macrophages. Stimulation of toll-like receptor 4 (TLR4) on macrophages by LPS activates a variety of transcription factors that include nuclear factor-κB (NF-κB), which in turn coordinate the induction of many genes encoding inflammatory mediators.Citation24) Furthermore, transcription factor NF-κB plays a key role in LPS-stimulated inflammatory cascades,Citation25) which is normally inactive in the cytoplasm of resting cells. Exposure of cells to LPS causes the translocation of NF-κB from cytoplasm to nucleus and regulates the expression of the inflammation-associated genes.Citation26) Therefore, possible inhibitors of NF-κB signaling pathway would be a good approach for the development of anti-inflammatory drugs.
Acanthopanax gracilistylus (AGS) belongs to the Araliaceae family, which is widely distributed in China, and its dried roots and stem barks have been commonly used to treat paralysis, arthritis, rheumatism, sinew, bone pains, lameness, and liver disease for many centuries in China.Citation27,28) However, the mechanism by which the biologically active components of AGS elicit these anti-inflammatory effects remains to be elucidated. Wu et al., reported anti-inflammatory effect of diterpenoids from the root bark of AGS.Citation29) No other reports were found about anti-inflammatory effects of active components in AGS. Our previous studies have isolated a number of chemical constituents from AGS,Citation30–33) and found that 3α-hydroxy-lup-20(29)-en-23, 28-dioic acid (HLEDA) is very abundant in AGS.
In the present, we investigated the anti-inflammatory effects of HLEDA and the possible molecular mechanisms involved using RAW 264.7 macrophages, which can be stimulated with LPS to mimic the conditions of the infection and inflammation. NO, TNF-α, IL-1β, and HMGB1were used as indicators in this in vitro study. We further investigated its molecular mechanisms of action by studying effects of the compound on nuclear translocation of NF-κB.
Materials and methods
Plant material
The leaves of AGS were collected in Changsha, Hunan province of China, in September 2012, and were botanically identified by Prof. Chang-Soo Yook, Kyung Hee University, Korea.
Chemicals and regents
RAW264.7 cell line was purchased from Shanghai Institute of Biochemistry and Cell Biology, Chinese Academy of Sciences (Shanghai, China). Lipopolysaccharide (LPS) and dimethyl sulfoxide (DMSO) were purchased from Sigma Chemicals (St. Louis, MO, USA). EZ4U cell proliferation and cytotoxicity assay kit was obtained from Biomedica (Vienna, Austria). Anti-HMGB1 antibody was ordered from Abcam (Cambridge, UK), secondary antibody conjugated with horseradish peroxidase-conjugated goat anti-rabbit IgG was purchased from Beyotime Institute of Biotechnology (Jiangsu, China). Commercially available sandwich ELISA kits TNF-α and IL-1β were obtained from Invitrogen Corporation (Camarillo, CA, USA). Anti-IκBα, anti-phosphorylated IκBα (anti-p-IκBα) mouse monoclonal antibodies were purchased from Cell signaling Technology Inc. (Danvers, USA). RPMI 1640 medium and fetal bovine serum (FBS) were purchased from Gibco-BRL (Grand Island, NY, USA). The other materials under study were endotoxin free.
Extraction and isolation
The isolation of HLEDA was performed as described previously.Citation30) Briefly, the dried leaves of AGS (1000 g) were extracted three times by hot methanol(3 × 10 L) through heating reflux at 65 °C. The combined methanol extract was evaporated under reduced pressure to obtain a residue (140 g), which was dissolved in water and partitioned with petroleum ether, ethyl acetate, and n-butyl alcohol successively, resulting in petroleum ether (6 g), ethyl acetate (42 g), and n-butyl alcohol (58 g) layers after removal of the solvent in a vacuum. The ethyl acetate soluble fraction (25 g) was chromatographed on silica gel (2.5 kg), eluted with chloroform, with the polarity gradually increased with methanol concentrations (25:1,20:1,15:1,10:1, and 5 L for each gradient) to give four fractions (Fr.1–Fr.4). Fr.4 (10:1) was chromatographed on silica gel which eluted with chloroform-methanol (10:1, v/v) to obtain HLEDA (3.6 g). The chemical structures of HLEDA was identified by analyzing the signals of spectral data (MS, 1H- and 13C-NMR, HMQC, HMBC, ROESY, and H–H COSY) and are shown in Fig. . HLEDA used in this study were checked by HPLC and were > 98% pure.
Cell culture and stimulation
RAW264.7 cell line was purchased from Shanghai Institute of Biochemistry and Cell Biology, Chinese Academy of Sciences. Macrophages were cultured in Dulbecco Modified Eagle’s medium (DMEM) supplemented with 10% FBS, 100 units/mL penicillin, and 100 units/mL streptomycin in tissue culture dishes at 37 °C, in a humidified atmosphere of 5% CO2 in air. For most experiments, cells were plated at a density of 1 × 106 cells/well on 12-well plates in 200 μL DMEM. Adherent RAW264.7 cells in 12-well culture plates were gently washed, and cultured in serum-reduced OPTI-MEM I medium 8 h before treating with vehicle or various concentrations of HLEDA (5–100 μM, dissolved with 0.1% DMSO). One hour after treatment with various concentrations of compounds or vehicle alone, LPS was added to a final concentration of 100 ng/mL at varying time intervals at 37 °C until further analysis.
Cytotoxicity assay
Cytotoxicity of HLEDA was assessed in RAW264.7 cells using an MTT-based test (EZ4U cell proliferation and cytotoxicity assay kit) following the manufacturer’s instructions. Briefly, 1 × 103cells/well were seeded in triplicate in 96-well microplates and cultured with various concentrations of HLEDA (5–100 μM) for 48 h. About 20 μL of EZ4U reagent was then added to each well and incubated for 4 h at 37 °C. Absorbance was recorded by a Spectrafluor Fluorometer (Tecan) at 450 nm with 620 nm as reference wave length. The spectrophotometer was calibrated to zero absorbance using culture medium without cells. The relative cell viability (%) relative to control wells was calculated by [A] test/[A] control × 100.
Measurement of nitrite in culture media
RAW264.7 cells were plated at 2.5 × 105 cells/mL in 96 well-plates and then incubated with or without LPS (100 ng/mL) in the absence or presence of various concentrations (10, 20, 30, 40, and 50 μM) of HLEDA for 24 h. The nitrite accumulated in culture medium was measured as an indication of NO production based on the Griess reaction. Briefly, 100 μL of cell culture medium was mixed up with 100 μL of Griess reagent [equal volumes of 1% (w/v) sulfanilamide in 5% (v/v) phosphoric acid and 0.1% (w/v) naphtylethylenediamine-HCl] incubated at room temperature for 10 min, and then the absorbance at 540 nm was measured in a microplate reader (Perkin Elmer Cetus, Foster City, CA, USA). Fresh culture medium was used as the blank in all experiments. The amount of nitrite in the samples was measured with the serial dilution standard curve of sodium nitrite.
Detection of TNF-α and IL-1β production
Macrophages were pretreated with various concentrations (10, 20, 30, 40, and 50 μM) of HLEDA or Dexamethasone (positive control, 10 μM) for 1 h then exposed to LPS (100 ng/mL) for 6 h. Culture media was collected and assayed using commercially available sandwich ELISA kits according to the manufacturer’s instructions (Invitrogen Corporation, Camarillo, CA, USA). Briefly, 50 μL of the incubation buffer, 50 μL standard diluent buffer, 50 μL standards, controls, or samples were added in triplicate to anti-TNF-α or anti-IL-1β coated ELISA microplates, and 50 μL biotin conjugate solution to the appropriate anti-mouse monoclonal antibody coated microtiter wells except the chromogen blank successively. Plates were covered and incubated at room temperature for 90 min. Solutions in the wells were aspirated and washed for four times with wash buffer. About 100 μL of streptavidin-HRP working solution was added to each well and incubated for 30 min at room temperature. Solutions were aspirated and wells were washed again four times. About 100 μL of Stabilized Chromogen was then added to each well, and incubated for 30 min at room temperature in the dark, after which 100 μL stop solution was added to each well, and absorbance was read at 450 nm using a plate reader (Perkin Elmer Cetus, Foster City, CA, USA). Absorbance values were normalized using a standard curve.
Reverse transcription polymerase chain reaction (RT-PCR) analysis
Macrophages were pretreated with HLEDA (10, 20, 30, 40, or 50 μM) for 1 h then exposed to LPS (100 ng/mL) for 2 h. After washing twice with PBS, total RNA was extracted from treated RAW264.7 cells by adding TRIzol reagent (Invitrogen, Carlsbad, CA) according to the manufacturer’s protocol. RNA isolation was carried out in an RNase-free environment. 4 μg of RNA was reverse-transcribed (RT) using MuLV reverse transcriptase (Promega, WI, USA), oligo (dT) 16 primer, dNTP (0.5 μM), and 1U RNase inhibitor. PCR analyses were then performed on the cDNA preparations to detect TNF-α, IL-1β, and β-actin (as an internal standard) gene expression using a DNA gene cycler (BIO-RAD, HC, USA). PCR primers used in this study are listed below (Takara biotechnology, Dalian, China):
sense strand TNF-α, 5′- GAATGGGTGTTCATCCATTCT -3′,
antisense strand TNF-α, 5′- GCTTAAGTGACCTCGGAGCTTACA -3′,
sense strand IL-1β, 5′- TTGACGGACCCCAAAGAGTG -3′,
antisense strand IL-1β, 5′- ACTCCTGTACTCGTGGAAGA -3′,
sense strand β-actin, 5′- ATGGTGGGAATGGGTCAGAAG -3′,
antisense strand β-actin, 5′- GGAAGATGTTACTCGACGAGC -3′.
After amplification, portions of the PCR reactions were electrophoresed on a 1.2% agarose gel and visualized by ethidium bromide staining and UV irradiation.
Western blot analysis
Macrophages were pretreated with HLEDA for 1 h then exposed to LPS (100 ng/mL) for 24 h. RAW264.7-conditioned medium was harvested, filtered, and concentrated through a Centricon YM-10 ultrafilter (Millipore, MS, USA) following the manufacturer’s instructions. Concentrated samples were frozen in aliquots at −80 °C.
Cells were also harvested and washed three times with cold PBS, and cellular extracts for total protein of RAW264.7 cells were prepared as follows. Briefly, the cell pellet was resuspended in 100 μL cell lysate buffer (50 mmol/L Tris, 150 mM NaCl, 1 mM PMSF, 0.1% SDS, 0.02% Sodium azide, and 1% Nonidet P-40). After mixing at 4 °C for 30 min on a shaking platform and ultrasonicating for 30 s, cellular extracts were centrifuged for 30 min in a microfuge at 4 °C, and supernatants were frozen in aliquots at -80 °C. Protein content was determined using the Enhanced BCA Protein Assay Kit (Beyotime, Jiangsu, China).
Approximately 15 μg total proteins from cellular extracts or 25 μL supernatant per lane were loaded onto a 12% SDS-PAGE gel. After electrophoretic separation, proteins were transferred to a polyvinylidene fluoride membrane. The membrane was blocked with 5% fat-free skim milk in Tris-buffered saline with tween-20 at room temperature for 1 h, and was incubated with anti-HMGB1 (Abcam, Cambridge, UK) or anti-β-actin primary antibody (1:1,000; Santa Cruz Technology, Santa Cruz, CA) at 4 °C overnight. After washing, the membrane was incubated with a secondary antibody conjugated with horseradish peroxidase-conjugated goat anti-rabbit IgG (1:5000 dilution) at room temperature for 1 h, and was followed by an extensive wash. The signal was visualized using ECL detection reagent (Thermo Fisher Scientific, CA, USA) and quantified by densitometry using Quantity One software. The expression level of HMGB1 protein in cellular extracts was normalized by β-actin levels.
Determination of nuclear translocation of NF-κB p65
Macrophages were pretreated with HLEDA (10, 20, 30, 40, or 50 μM) for 1 h then exposed to LPS (100 ng/mL) for 2 h. After the stimulation period, the RAW264.7 cells were harvested and washed 3 times with cold PBS. The cytoplasmic and nuclear extracts were prepared using a nuclear/cytosol fractionation kit (Biovision, Inc., Mountain View, CA, USA) according to manufacturer’s instructions. Cytoplasmic and nuclear fractions were measured for levels of NF-kB using a commercially available NF-κB p65 ELISA kit (Cell Signaling Technology, Inc.), according to the manufacturer’s instructions.
Immunoblot for IκB
Macrophages were pretreated with HLEDA (10, 20, 30, 40, or 50 μM) for 1 h then exposed to LPS (100 ng/mL) for 2 h. LPS-stimulation was stopped by the addition of an ice-cold phosphate-buffered saline. The cells were then lysed for immunoblotting. The cytoplasmic or nuclear extracts were prepared from LPS-treated cells by nuclear/cytosol fractionation kit (Biovision, Inc., Mountain View, CA, USA) and then resolved on 10% SDS-polyacrylamide gels. After electrophoresis, the proteins were transferred onto a polyvinylidene difluoride membrane, and the membrane was blocked in Tris-buffered saline containing 0.05% Tween-20 (TBS-T) supplemented with 5% skim milk (Sigma) at room temperature for 2 h. Phosphorylation-specific antibodies against IκBα were used as the primary antibody. They were diluted 1:1000 in TBS-T and incubated with the membrane at 4 °C overnight. Subsequently, the membrane was incubated with peroxidase-conjugated secondary antibody at room temperature for 1 h. The immunoreactive proteins were detected using an enhanced chemiluminescence Western blotting detection kit. The β-actin protein served as an internal control.
Statistical analyses
All continuous data were expressed as the mean ± SD. Comparison between groups was performed by one way-ANOVA analysis, Student’s t-test or Dunnet’s test. Statistical significance was defined as a p < 0.05. All statistical analyses were performed through SPSS 16.0 for Windows.
Results
Cell viability
To investigate the cytotoxicity of HLEDA on RAW264.7 macrophages, we analyzed the viability of RAW264.7 cells after treatment with HLEDA using an MTT-based EZ4U assay. The relative cytotoxicity of HLEDA at different concentrations is shown in Fig. . Cytotoxicity was only observed in RAW264.7 cells treated with concentrations of HLEDA higher than 50 μM. Therefore, subsequent experiments were performed at concentrations of 50 μM or less for HLEDA.
Fig. 2. Cell viability in RAW264.7 macrophages treated with HLEDA. RAW264.7 cells were exposed to HLEDA at the indicated concentrations for 48 h and evaluated by MTT-based EZ4U test. Cell viability of treated samples was expressed as a percentage of surviving cells compared with that in the vehicle control. Values are expressed as mean ± S.D. of three individual experiments performed in triplicate. CON stands for vehicle control. *p < 0.05 vs. the control group.
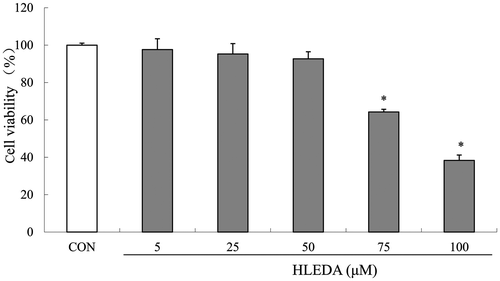
HLEDA inhibit NO production in RAW264.7 macrophages
The effects of HLEDA on NO production in LPS-induced RAW 264.7 macrophages were tested by NO assay. The Griess reagent was used to measure the accumulated nitrite in the culture media. Cells were stimulated with LPS (100 ng/mL) in the absence or presence of HLEDA at various concentrations (10, 20, 30, 40, and 50 μM) for 24 h. Following 24 h incubation, RAW264.7 macrophages produced 3.68 ± 0.37 μM nitrite in the resting state. However, NO production in the culture medium of the LPS (100 ng/mL) stimulated cells was increased dramatically to 9.01 ± 0.51 μM after 24 h. As shown in Fig. , when the concentration of HLEDA increased, the effects of antagonizing NO releasing significantly elevated, which showed a tendency of concentration-dependent manner. HLEDA gave good inhibitory effect on nitrite production.
Fig. 3. Inhibitory effects of HLEDA on LPS-induced NO production in RAW264.7 macrophages. RAW264.7 macrophages were pretreated with different concentrations (10, 20, 30, 40, and 50 μM) of HLEDA for 1 h, then with LPS (100 ng/mL), and being incubated for 24 h. Control (CON) values were obtained in the absence of LPS and HLEDA. LPS values were obtained in the presence of LPS(100 ng/mL) and absence of HLEDA. The values shown are means ± SD of three independent experiments. #p < 0.05 vs. the negative controls; *p < 0.05 vs. 100 ng/ml LPS-treated cells; the significances of differences between treated groups were determined through ANOVA and Dunnett’s post hoc test.
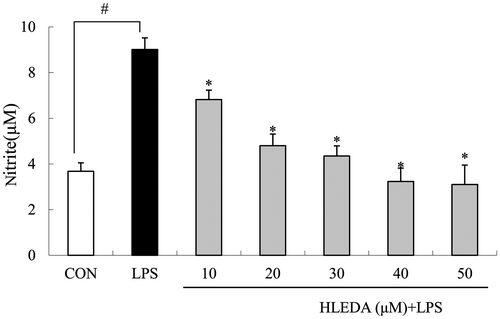
HLEDA inhibit TNF-α and IL-1β production in RAW264.7 macrophages
TNF-α and IL-1β are multifunctional proinflammatory cytokines involved in the regulation of the immune response, hematopoiesis, and inflammation. To examine the effect of HLEDA on the release of TNF-α and IL-1β, RAW264.7 cells were incubated with HLEDA for 1 h then exposed to LPS (100 ng/mL) for 6 h to induce inflammation. The quantities of TNF-α and IL-1β secreted from RAW264.7 cells was determined using an ELISA assay on the supernatants. Dexamethasone (10 μM), which is a widely used anti-inflammatory agent, was used as a positive control.
In LPS-stimulated RAW264.7 cells pretreated with HLEDA, the secretion of TNF-α significantly decreased in a concentration-dependent manner as compared to control treated cells (p < 0.05, Fig. (A)), IL-1β secretion was also significantly decreased in a concentration-dependent manner (p < 0.05, Fig.(B)).
Fig. 4. Effect of HLEDA on LPS-induced TNF-α and IL-1β production and mRNA expression in RAW264.7 cells. A-B: Effect of HLEDA on LPS-induced TNF-α (A) and IL-1β (B) production. The concentration of TNF-α and IL-1β secreted by RAW264.7 was measured by commercially available sandwich ELISA kits. Control (CON) values were obtained in the absence of LPS and HLEDA. LPS values were obtained in the presence of LPS (100 ng/mL) and absence of HLEDA. The positive control (DEX) was obtained in the presence of LPS (100 ng/mL) and Dexamethasone (10 μM). Three independent experiments were performed with similar results. Data is expressed as mean ± S.D. Student’s t-test was used to determine significant differences between two groups. #p < 0.05 vs. negative controls (CON); *p < 0.05 vs. 100 ng/mL LPS-treated cells. C-D: Effects of HLEDA on mRNA expression of TNF-α and IL-1β in LPS-stimulated macrophages. RAW 264.7 cells were preincubated with various concentrations of HLEDA for 1 h and were then treated with 100 ng/mL LPS for an additional 2 h. mRNA expression of TNF-α (C) and IL-1β (D) was analyzed by real-time RT-PCR and β-actin was used as the internal control.
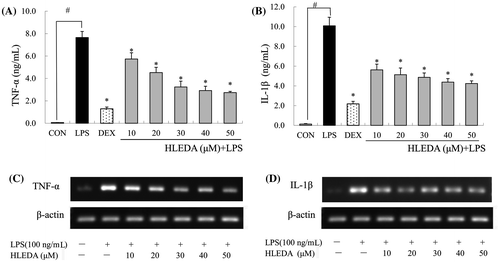
HLEDA suppress TNF-α and IL-1β mRNA expression in LPS-stimulated RAW264.7 macrophages
As HLEDA was found to inhibit the secretion of pro-inflammatory mediators TNF-α and IL-1β, we further investigated the effects of HLEDA on LPS-induced TNF-α and IL-1β gene expression using RT-PCR. HLEDA significantly reduced LPS-induced mRNA expression of TNF-α (Fig. (C)) and IL-1β (Fig. (D)), which is in accordance with ELISA data on the effects of HLEDA on TNF-α and IL-1β secretion.
Effect of HLEDA on HMGB1 secretion in LPS-induced RAW264.7 macrophages
To examine the effects of HLEDA on the expression of HMGB1, RAW264.7 cells were incubated with HLEDA then exposed to LPS (100 ng/mL). HMGB1 protein levels from the cell supernatant or whole cell lysate were analyzed by Western blot analysis.
HMGB1 protein levels were not detected in the RAW264.7 cell supernatant without LPS stimulation. And HMGB1 protein levels in the RAW264.7 cell supernatant were significantly increased after LPS stimulation. However, preincubation with HLEDA reversed this effect. The relative increase in expression of secreted HMGB1 in the supernatant after LPS stimulation was significantly reduced by treatment with HLEDA (Fig. (A)).
Fig. 5. Effect of HLEDA on the expression of HMGB1 protein in LPS-induced RAW264.7 cells. A: Effect of different concentrations of HLEDA on the expression of HMGB1 protein in the supernatant and quantitative densitometric analysis of HMGB1 protein in LPS-treated RAW264.7 cells. Mean values ± SD (n = 3). *p < 0.05 compared with the LPS-treatment group. B: Effect of HLEDA on total HMGB1 protein content in RAW264.7 cells. The expression level of HMGB1 protein in cellular extracts was normalized by β-actin levels.
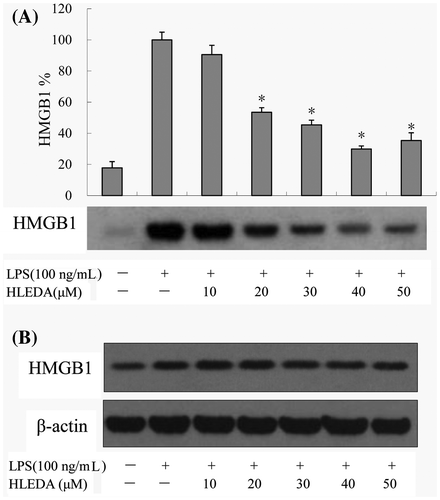
By Western blot analysis of whole cell lysates, we found that in LPS-treated RAW264.7 pretreated with HLEDA at 10, 20, 30, 40, or 50 μM, the expression of HMGB1 protein did not change significantly. Thus, the addition of HLEDA caused a significant decrease in the secretion of HMGB1; however, it did not change the steady-state level of HMGB1 protein in RAW 264.7 cells. (Fig. (B))
HLEDA inhibits nuclear translocation of NF-κB in LPS-stimulated RAW264.7 macrophages
LPS is known to produce pro-inflammatory-associated gene expression through the activation of NF-κB. Based on the inhibition of inflammatory mediators and pro-inflammatory cytokines in RAW264.7 macrophages by HLEDA, we used ELISA to test the effects of HLEDA on LPS-induced translocation of the NF-κB p65 subunit in RAW264.7 macrophages. Our experiments showed that treatment with LPS-induced translocation of the p65 subunit from the cytosol to the nucleus. This process was significantly inhibited by all five concentrations of HLEDA tested in a concentration-dependent manner (Fig. ).
Fig. 6. Effect of HLEDA on nuclear translocation of NF-κB in LPS-induced RAW264.7 cells. HLEDA inhibits LPS-induced translocation of the p65 subunit from the cytosol to the nucleus in a concentration-dependent manner. RAW264.7 cells were stimulated with or without LPS (100 ng/mL) in the absence or presence of HLEDA (10, 20, 30, 40, or 50 μM) for 2 h. The cytoplasmic and nuclear extracts were prepared using a nuclear/cytosol fractionation kit, and were measured for levels of NF-κB using a commercially available NF-κB p65 ELISA kit (Cell Signaling Technology, Inc.). Control (CON) values were obtained in the absence of LPS and HLEDA. LPS values were obtained in the presence of LPS (100 ng/mL) and absence of HLEDA. Mean values ± SD (n = 3). #p < 0.05 compared with the control group. *p < 0.05 compared with the LPS-treatment group.
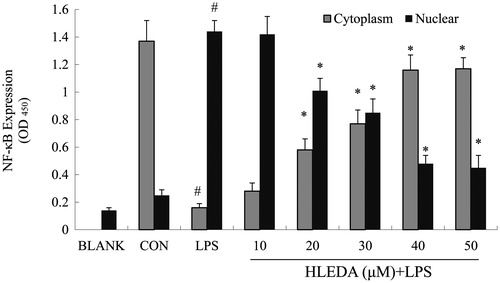
Inhibitory effects of HLEDA on the LPS-induced phosphorylation and degradation of IκB
In unstimulated macrophage cells, NF-κB is sequestered in the cytosol by its inhibitor IκB-α, and when cells are stimulated with LPS, IκB is phosphorylated by its inhibitor IκB kinases, ubiquitinated, and rapidly degraded via 26S proteasome, which results in the release of NF-κB.Citation34) Here, we examined the effects of HLEDA on the LPS-induced phosphorylation and degradation of IκB-α by Western blotting. In resting cells, the phosphorylated forms of IκB-α were hardly detectable, but after treatment with LPS (100 ng/mL) alone for 2 h, phosphorylated IκB-α levels were elevated (Fig. ). Furthermore, HLEDA significantly and concentration-dependently reduced the LPS-induced phosphorylation and degradation of IκB-α.
Fig. 7. Inhibitory effect of HLEDA on IκB-α phosphorylation and degradation. RAW 264.7 macrophage cells were treated with LPS (100 ng/mL) in the absence or presence of the indicated concentrations of HLEDA for 2 h. Total cellular proteins were prepared and Western blotted for IκB-α and pIκB-α using specific antibodies. β-actin was used as an internal loading control.
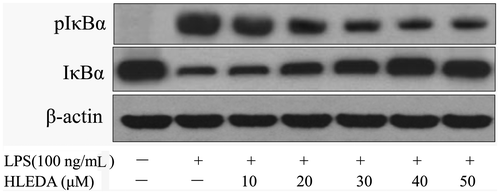
Discussion
Many previous studies on natural herbal medicines have been conducted to find the potential natural anti-inflammatory products through in vitro and in vivo systems. AGS is one of important oriental herbs and has been used to treat various diseases such as rheumatism, paralysis, arthritis, sinew, bone pains, and tonic since ancient times. Although several pharmacological and biochemical pharmacokinetic studies of AGS-derived compounds have been conducted previously, the existence of potential anti-inflammatory properties of lupane-type triterpenoids had not been explored. On the other hand, Pentacyclic triterpenes have a wide distribution in plants, many of which have been used in empirical anti-inflammatory therapies. Especially oleanolic acid is commonly used in the therapy of hepatitis in clinic. Previous studies showed that lots of lupane-type triterpenes exert anti-inflammatory effects by inhibiting NF-κB activation, such as betulin, 28-acetoxy-betulin, epibetulin, epibetulinic acid, acankoreoside R etc.Citation35–37) Kim et al. reported impressic acid, which just has a bit different with HLEDA, can inhibit tumor necrosis factor (TNF)-α induced NF-κB activity and to upregulate transcriptional activity of PPARγ in HepG2.Citation38) For the first time we investigated the anti-inflammatory activity of HLEDA extracted from AGS in LPS-stimulated RAW 264.7 murine macrophages. Since LPS plays a key role in inflammation by stimulating macrophages to produce excessive inflammatory mediators such as NO, PGE2, and proinflammatory cytokines,Citation1) those may cause various inflammatory diseases.Citation39,40) So firstly, our results showed that lupane-type triterpene HLEDA from AGS exhibited potent anti-inflammatory activity against LPS-stimulated production of NO, and cytokines (TNF-α, IL-1β). HLEDA showed concentration-dependent inhibitory effects on NO production in LPS-stimulated RAW 264.7 murine macrophages. HLEDA markedly down-regulated the LPS-induced TNF-α and IL-1β production and mRNA expression of TNF-α and IL-1β in concentration-dependent manner. The results presented here demonstrate that HLEDA is an effective anti-inflammatory component.
Considered a late inflammatory cytokine, HMGB1 appears relatively late in the inflammatory response and has a long duration. Serum concentrations of HMGB1 increased late (16~24 h) in patients with sepsis.Citation10,41) HMGB1 protein inhibitors or antagonists have been shown to significantly reduce the incidence of lethal endotoxemia and resulting acute tissue damage, even when given 24 h after the occurrence of endotoxemia and sepsis in mice.Citation42) HMGB1 may also enhance the inflammatory response by stimulating a variety of cells to synthesize other proinflammatory cytokines. Therefore, inhibition of HMGB1 is considered to be a good target for reducing mortality and complications in patients with sepsis. In the present in vitro study, the effects of HLEDA on HMGB1 secretion in LPS-induced RAW264.7 macrophages were investigated for the first time. The results also show that HLEDA significantly reversed elevated HMGB1 protein levels after LPS stimulation in the RAW264.7 cell supernatant. Since HLEDA didn’t change the protein levels of HMGB1, so the downregulation caused by HLEDA may through the inhibition of HMGB1 secretion. So HLEDA may also be potential drug for reducing HMGB1-inducing inflammation.
LPS and other inflammatory stimuli have been reported to activate the NF-κB signaling pathways in macrophages.Citation43) In particular, NF-κB is an important upstream regulator of cytokine, COX-2, and iNOS expressions and blockade of NF-κB transcriptional activity in macrophages can suppress expression of iNOS, COX-2, and the proinflammatory cytokines, such as TNF-α, IL-1β, IL-6, and HMGB1.Citation44) NF-κB is present in the cytosol as either a homodimer or heterodimer and is linked to IκB. After the LPS-induced phosphorylation and degradation of IκB-α in the cytosol, NF-κB subunits are free to translocate to the nucleus.Citation45,46) After nuclear translocation, the NF-κB subunits bind to the promoters of target genes of pro-inflammatory factors.Citation45) Therefore, blocking the transcriptional activity of NF-κB in the macrophage nucleus can suppress the expression of inflammatory mediators. We found that HLEDA inhibited the LPS-induced phosphorylation and degradation of IκB-α and nuclear translocation of NF-κB p65 subunits. Therefore, these results suggest that HLEDA inhibits the expression of NO, TNF-α, IL-1β, and HMGB1 through the inactivation of NF-κB by reducing IκB-α degradation and phosphorylation.
In conclusion, our results demonstrate that AGS-derived lupane-type triterpene HLEDA, efficiently decreased nuclear factor-kappaB (NF-κB) activation by inhibiting the degradation and phosphorylation of IκBα. These results suggest that HLEDA exerts anti-inflammatory properties in LPS-induced macrophages, possibly through inhibition of the NF-κB signaling pathway, which mediates the expression of pro-inflammatory cytokines NO, TNF-α, IL-1β, and HMGB1. Accordingly, our results suggest that HLEDA is a promising therapeutic agent for treating inflammatory diseases, such as rheumatic arthritis, rheumatoid arthritis, scapulohumeral periarthritis, and cervical spondylosis and slipped disk. Although this research indicates the potential of HLEDA as an anti-inflammatory compound, which may be a valuable therapeutic agent in the treatment of inflammation related diseases. There remains a great deal more to be studied and explored about HLEDA. We need to study whether the similar protective effect of HLEDA on LPS-induced systemic inflammatory response and subsequent vital organs injuries in septic animals. And we also need to investigate the bioavailability of HLEDA. Furthermore, discovery of the anti-inflammatory properties of HLEDA suggests that future studies should be conducted to identify other potentially beneficial pharmacological mechanisms of AGS-derived compounds.
Funding
This work was supported by Natural Science Foundation of Hunan Province, China [grant number 11JJ2042]; Key Projects of Changsha City Science and Technology Bureau [grant number k1403122-31].
Author contributions
Xiang-Qian Liu, Qin-Peng Zou, Chang-Soo Yook, and Hyeong-Kyu Lee designed the study. Xiang-Qian Liu and Qin-Peng Zou co-wrote the paper. Jian-Jun Huang assisted in writing the paper. Qin-Peng Zou, Jian-Jun Huang, Wan-Kyunn Whang, and Hyeong-Kyu Lee performed the experiments. Xiang-Qian Liu, Jian-Jun Huang, and Ok-Kyoung Kwon contributed to data collection and interpretation. All authors reviewed and approved the final manuscript.
Disclosure statement
No potential conflict of interest was reported by the authors.
References
- Morson BC. Pathology of inflammatory diseases. Proc R Soc Med. 1970;63(Suppl):63.
- Nanjundaiah SM, Stains JP, Moudgil KD. Kinetics and interplay of mediators of inflammation-induced bone damage in the course of adjuvant arthritis. Int J Immunopathol Pharmacol. 2013;26(1):37–48.10.1177/039463201302600104
- Moreira AP, Hogaboam CM. Macrophages in allergic asthma: fine-tuning their pro- and anti-inflammatory actions for disease resolution. J Interferon Cytokine Res. 2011;31:485–491.10.1089/jir.2011.0027
- Hanken K, Eling P, Hildebrandt H. The representation of inflammatory signals in the brain – a model for subjective fatigue in multiple sclerosis. Front Neurol. 2014;5:264. doi:10.3389/fneur.2014.00264
- Hansson GK, Hermansson A. The immune system in atherosclerosis. Nat Immunol. 2011;12:204–212.10.1038/ni.2001
- Wang X, Zhu M, Hjorth E, et al. Resolution of inflammation is altered in Alzheimer’s disease. Alzheimers Dement. 2015;11(1):40–50.10.1016/j.jalz.2013.12.024
- Ettinger R, Mebius R, Browning JL, et al. Effects of tumor necrosis factor and lymphotoxin on peripheral lymphoid tissue development. Int Immunol. 1998;10(6):727–741.10.1093/intimm/10.6.727
- Shohami E, Ginis I, Hallenbeck JM. Dual role of tumor necrosis factor alpha in brain injury. Cytokine Growth Factor Rev. 1999;10(2):119–130.10.1016/S1359-6101(99)00008-8
- Wang GJ, Chen SM, Chen WC, et al. Selective inducible nitric oxide synthase suppression by new bracteanolides from Murdannia bracteata. J Ethnopharmacol. 2007;112(2):221–227.
- Wang H, Bloom O, Zhang M, et al. HMG-1 as a late mediator of endotoxin lethality in mice. Science. 1999;285:248–251.10.1126/science.285.5425.248
- Bustin M. Regulation of DNA-dependent activities by the functional motifs of the high-mobility-group chromosomal proteins. Mol Cell Biol. 1999;19:5237–5246.10.1128/MCB.19.8.5237
- Abraham E, Arcaroli J, Carmody A, et al. HMG-1 as a mediator of acute lung inflammation. J Immunol. 2000;165:2950–2954.10.4049/jimmunol.165.6.2950
- Scaffidi P, Misteli T, Bianchi ME. Release of chromatin protein HMGB1 by necrotic cells triggers inflammation. Nature. 2002;418:191–195.10.1038/nature00858
- Lotze MT, Tracey kJ. High-mobility group box 1 protein (HMGB1): nuclear weapon in the immune arsenal. Nat Rev Immunol. 2005;5(4):331–342.10.1038/nri1594
- Andersson U, Tracey kJ. HMGB1 is a therapeutic target for sterile inflammation and infection. Annu Rev Immunol. 2011;29:139–162.10.1146/annurev-immunol-030409-101323
- Harris HE, Raucci A. Alarmin(g) news about danger: workshop on innate danger signals and HMGB1. EMBO Rep. 2009;7(8):774–778.
- Bianchi ME. DAMPs, PAMPs and alarmins: all we need to know about danger. J Leukoc Biol. 2007;81(1):1–5.
- Andersson U, Wang H, Palmblad K, et al. High mobility group 1 protein (Hmg-1) stimulates proinflammatory cytokine synthesis in human monocytes. J Exp Med. 2000;192(4):565–570.10.1084/jem.192.4.565
- Sha Y, Zmijewski J, Xu Z. HMGB1 develops enhanced proinflammatory activity by binding to cytokines. J Immunol. 2008;180(4):2531–2537.10.4049/jimmunol.180.4.2531
- Li X, Wang LK, Wang LW, et al. Cisplatin protects against acute liver failure by inhibiting nuclear HMGB1 release. Int J Mol Sci. 2013;14(6):11224–11237.10.3390/ijms140611224
- Lee WS, Shin JS, Jang DS, et al. Cnidilide, an alkylphthalide isolated from the roots of Cnidium officinale, suppresses LPS-induced NO, PGE2, IL-1β, IL-6 and TNF-α production by AP-1 and NF-κB inactivation in RAW 264.7 macrophages. Int Immunopharmacol. 2016;40:146–155.10.1016/j.intimp.2016.08.021
- Murray PJ, Wynn TA. Protective and pathogenic functions of macrophage subsets. Nat Rev Immunol. 2011;11(11):723–737.10.1038/nri3073
- Wang H, Yang H, Czura CJ, et al. HMGB1 as a late mediator of lethal systemic inflammation. Am J Respir Crit Care Med. 2001;164(10 Pt 1):1768–1773.10.1164/ajrccm.164.10.2106117
- Gholijani N, Gharagozloo M, Farjadian S, et al. Modulatory effects of thymol and carvacrol on inflammatory transcription factors in lipopolysaccharide-treated macrophages. J Immunotoxicol. 2015;13(2):157–164.
- Iwanowycz S, Wang J, Altomare D, et al. Emodin bi-directionally modulates macrophage polarization and epigenetically regulates macrophage memory. J Biol Chem. 2016;291(22):11491–11503.10.1074/jbc.M115.702092
- Gilmore TD. Introduction to NF-κB: players, pathways, perspectives. Oncogene. 2006;25:6680–6684.10.1038/sj.onc.1209954
- Shan BE, Yoshita Y, Sugiura T. Suppressive effect of Chinese medicinal herb, Acanthopanax gracilistylus, extract on human lymphocytes in vitro. Clin Exp Immunol. 1999;118:41–48.10.1046/j.1365-2249.1999.01031.x
- The state pharmacopoeia commission of P.R. China. Pharmacopoeia of People’s Republic of China. Vol. 1. Beijing: Chinese Medical Science and Technology Press; 2010. p. 61.
- Wu ZY, Zhang YB, Zhu KK, et al. Anti-inflammatory diterpenoids from the root bark of acanthopanax gracilistylus. J Nat Prod. 2014;77(11):2342–2351.10.1021/np500125x
- Zou QP, Liu XQ, Lee HK, et al. Lupane- triterpenoids from the methanol extracts of leaves of Acanthopanax gracilistylus W.W.Smith. J Lanzhou Univ (Natural Sciences). 2011;47(6):120–126.
- Liu XQ, Yook CS, Chang SY. Chemical constituents of Acanthopanax gracilistylus. Chin Tradit Herb Drugs. 2004;35(3):250–252.
- Liu XQ, Chang SY, Park SY, et al. A new lupane-triterpene glycoside from the leaves ofAcanthopanax gracilistylus. Arch Pharm Res. 2002;25(6):831–836.10.1007/BF02977000
- Yook CS, Liu XQ, Chang SY, et al. Lupane triterpene glycosides from the leaves of Acanthopanax gracilistylus. Chem Pharm Bull (Tokyo). 2002;50(10):1383–1385.
- Cho W, Nam JW, Kang HJ, et al. Zedoarondiol isolated from the rhizoma of Curcuma heyneana is involved in the inhibition of iNOS, COX-2 and pro-inflammatory cytokines via the downregulation of NF-kappaB pathway in LPS-stimulated murine macrophages. Int Immunopharmacol. 2009;9:1049–1057.10.1016/j.intimp.2009.04.012
- Kim JA, Son JH, Yang SY, et al. A new lupane-type triterpene from the seeds of Panax ginseng with its inhibition of NF-κB. Arch Pharm Res. 2012;35(4):647–651.10.1007/s12272-012-0408-0
- Reyes CP, Núñez MJ, Jiménez IA, et al. Activity of lupane triterpenoids from Maytenus species as inhibitors of nitric oxide and prostaglandin E2. Bioorg Med Chem. 2006;14(5):1573–1579.10.1016/j.bmc.2005.10.063
- Dat LD, Thao NP, Luyen BT, et al. A new saponin from Acanthopanax koreanum with anti-inflammatory activity. Arch Pharm Res. 2016. DOI:10.1007/s12272-016-0879-5
- Kim JA, Yang SY, Song SB, et al. Effects of impressic acid from Acanthopanax koreanum on NF-κB and PPARγ activities. Arch Pharm Res. 2011;34(8):1347–1351.10.1007/s12272-011-0815-7
- Roberts-Thomson IC, Fon J, Uylaki W, et al. Cells, cytokines and inflammatory bowel disease: a clinical perspective. Expert Rev Gastroenterol Hepatol. 2011;5(6):703–716.10.1586/egh.11.74
- Debnath T, Da Kim H, Lim BO. Natural products as a source of anti-inflammatory agents associated with inflammatory bowel disease. Molecules. 2013;18(6):7253–7270.10.3390/molecules18067253
- Gao H, Wu L, Kuroyanagi M, et al. Antitumor-promoting constituents from Chaenomeles sinensis KOEHNE and their activities in JB6 mouse epidermal cells. Chem Pharm Bull (Tokyo). 2003;51:1318–1321.10.1248/cpb.51.1318
- Fogo AS, Antonioli E, Calixto JB, et al. Tormentic acid reduces vascular smooth muscle cell proliferation and survival. Eur J Pharmacol. 2009;615:50–54.10.1016/j.ejphar.2009.05.009
- Chang Y, Huang X, Liu Z, et al. Dexmedetomidine inhibits the secretion of high mobility group box 1 from lipopolysaccharide-activated macrophages In vitro. J Surg Res. 2013;181:308–314.10.1016/j.jss.2012.07.017
- Moon DO, Park SY, Lee kJ, et al. Bee venom and melittin reduce proinflammatory mediators in lipopolysaccharide-stimulated BV2 microglia. Int Immunopharmacol. 2007;7(8):1092–1101.10.1016/j.intimp.2007.04.005
- Karin M, Ben-Neriah Y. Phosphorylation meets ubiquitination: the control of NF-[kappa] B activity. Annu Rev Immunol. 2000;18:621–663.10.1146/annurev.immunol.18.1.621
- Karin M, Delhase M. The IκB kinase (IKK) and NF-κB: key elements of proinflammatory signalling. Semin Immunol. 2000;12:85–98.10.1006/smim.2000.0210