Abstract
Bacillus cereus and Bacillus megaterium both accumulate polyhydroxyalkanoate (PHA) but their PHA biosynthetic gene (pha) clusters that code for proteins involved in PHA biosynthesis are different. Namely, a gene encoding MaoC-like protein exists in the B. cereus-type pha cluster but not in the B. megaterium-type pha cluster. MaoC-like protein has an R-specific enoyl-CoA hydratase (R-hydratase) activity and is referred to as PhaJ when involved in PHA metabolism. In this study, the pha cluster of B. cereus YB-4 was characterized in terms of PhaJ’s function. In an in vitro assay, PhaJ from B. cereus YB-4 (PhaJYB4) exhibited hydration activity toward crotonyl-CoA. In an in vivo assay using Escherichia coli as a host for PHA accumulation, the recombinant strain expressing PhaJYB4 and PHA synthase led to increased PHA accumulation, suggesting that PhaJYB4 functioned as a monomer supplier. The monomer composition of the accumulated PHA reflected the substrate specificity of PhaJYB4, which appeared to prefer short chain-length substrates. The pha cluster from B. cereus YB-4 functioned to accumulate PHA in E. coli; however, it did not function when the phaJYB4 gene was deleted. The B. cereus-type pha cluster represents a new example of a pha cluster that contains the gene encoding PhaJ.
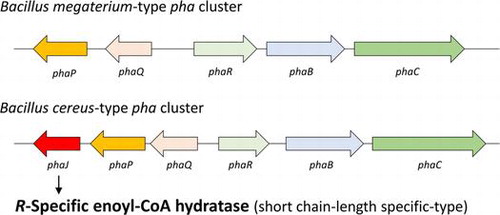
Polyhydroxyalkanoates (PHAs) are reserve polymeric materials that are accumulated as intracellular granules by a wide variety of bacteria.Citation1) Recently, PHAs have attracted industrial attention for use as biodegradable and biocompatible thermoplastics, which can be produced by bacterial fermentation from certain types of renewable biomass, such as sugars and plant oils. PHAs are thus expected to solve some environmental problems associated with the use of petroleum.
The biosynthetic pathway of PHA is shown in Fig. .Citation2) PHA is polymerized by PHA synthase (PhaRC or PhaC) from (R)-3-hydroxyacyl-CoA (R-3HA-CoA) as a monomeric substrate with releasing free CoA. The most typical monomeric substrate for PhaRC is (R)-3-hydroxybutyryl-CoA (R-3HB-CoA), which is usually generated from two acetyl-CoAs via the dimerization pathway that involves 3-ketothiolase (PhaA) and NADPH-dependent acetoacetyl-CoA reductase (PhaB). Alternatively, the β-oxidation cycle is also a source of a monomeric substrate for PHA biosynthesis. The intermediate of β-oxidation, trans-2-enoyl-CoA, is converted to R-3HA-CoA by the action of R-specific enoyl-CoA hydratase (R-hydratase, PhaJ). Thus, PhaJs channel β-oxidation products toward PHA biosynthesis. To date, different types of PhaJs have been found, namely, a short chain-length (C4-C6) enoyl-CoA-specific type and a medium chain-length (C8-C12) enoyl-CoA-specific type. Pseudomonas aeruginosa is known to have both types of R-hydratase, which functioned as monomer suppliers for PHA biosynthesis in recombinant Escherichia coli.Citation3,4)
Fig. 1. Proposed metabolic pathway for polyhydroxyalkanoate (PHA) metabolism in B. cereus YB-4.Citation2)
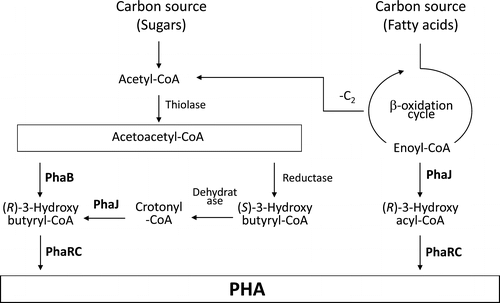
Among PHA-producing bacteria, Aeromonas caviae is the first bacterium that was identified to have a PHA biosynthesis gene (pha) locus containing a phaJ gene.Citation5) A. caviae has a pha operon consisting of phaP-phaC-phaJ, where phaP encodes the gene for the PHA granule associated protein phasin (PhaPAc). The A. caviae PhaJAc is an R-hydratase that preferably shows activity toward short chain-length enoyl-CoA substrates.Citation6) Additionally, Rhodospirillum rubrum,Citation7 Methylobacterium rhodesianum,Citation8) Haloferax mediterranei,Citation9) P. aeruginosa,Citation3,4) Pseudomonas oleovorans,Citation10) Pseudomonas putida,Citation11,12) Ralstonia eutropha,Citation13) and othersCitation14–16) have been reported to possess R-hydratases. However, phaJ is not located in the pha operon or pha cluster in species other than A. caviae, H. mediterranei, and their relatives.Citation5,9,16)
McCool and CannonCitation17) reported that the pha cluster in Bacillus megaterium is composed of a phaR-phaB-phaC operon and a phaQ-phaP operon in the opposite direction, in which phaR and phaQ encode genes for the PHA synthase subunit and transcriptional regulator, respectively (Fig. ). Recently, whole-genome sequencing of some PHA-producing Bacillus strains has been performed. The sequencing analysis provides a new insight into the pha cluster of Bacillus, that is, Bacillus cereus and its relatives such as Bacillus thuringiensis and Bacillus anthracis may have a pha cluster containing a phaJ gene. Namely, the pha cluster may be composed of a phaR-phaB-phaC operon and a phaQ-phaP-phaJ operon in the opposite direction. The difference between these pha clusters is the presence or absence of the possible phaJ as the downstream gene of phaP. In the gene database, the possible phaJ is currently annotated as the gene for MaoC-like protein. However, if the protein exhibits R-hydratase activity and is involved in PHA metabolism, it should be referred to as PhaJ. It is of great interest to determine whether the gene product supplies monomers for PHA biosynthesis, because B. cereus and its relatives may be new examples of pha clusters containing a phaJ gene.
Fig. 2. B. megaterium-type and B. cereus-type pha clusters.
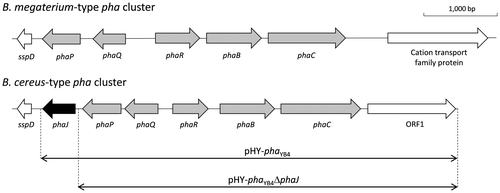
B. cereus YB-4 was isolated as a PHA-producing bacterium from ammunition-polluted soil in Kitakyushu City, Japan.Citation18) In our previous studies,Citation19–24) we demonstrated that the PHA synthase from B. cereus YB-4 (PhaRCYB4) catalyzes not only PHA polymerization but also the alcoholytic cleavage of PHA chains. The alcoholysis activity is observed when a PHA monomer is absent but an alcohol compound such as ethanol or propanol is present. This unique property of PhaRCYB4 enables the synthesis of low-molecular-weight and end-structure-regulated PHA.Citation2,24) On the other hand, by performing a sequencing analysis of pha cluster in B. cereus YB-4, it was revealed that strain YB-4 also has a putative phaJ, as do other strains of B. cereus and B. thuringiensis.
In this study, the pha cluster of B. cereus YB-4 was characterized in terms of PhaJ’s function. PhaJ from B. cereus YB-4 (PhaJYB4) was assayed in vitro and in vivo to determine whether PhaJYB4 functions as an R-hydratase and monomer supplier. This study reveals that the B. cereus-type pha cluster is a new example of a pha cluster that contains the gene encoding PhaJ.
Materials and methods
Bacterial strains and plasmid construction
The bacterial strains and plasmids used in this study are listed in Table . The phaJYB4 gene was amplified by polymerase chain reaction (PCR) using the B. cereus YB-4 genome as a template. Oligonucleotide primers for the PCR amplification were as follows: 5′-tag cat atg aat cca ttt caa gaa gca c-3′ and 5′- tag gga tcc cta ctc ttt ttt tag tat tgt cgc -3′ (the underlined sequences denote NdeI and BamHI sites). The resultant fragment was cloned into pET-3a (Apr, Novagen, WI, USA) at NdeI and BamHI sites, yielding pET-3a-phaJYB4. This plasmid was digested with XbaI and BamHI, and the resultant fragment containing an open reading frame (ORF) and pET-3a’s ribosome binding site was cloned into the same restriction sites of pUC19 to yield pUC19-phaJBY4.
Table 1. Bacterial strains and plasmids used in this study.
The pBBR1-phaRCYB4 was constructed by deleting phaABRe genes from pBBR1-phaRCYB4ABReCitation19) as follows: The pBBR1-phaRCYB4ABRe was digested with NruI and SalI and the 8.9 kb DNA fragment containing phaRCYB4 was blunted and self-ligated.
The pha cluster of B. cereus YB-4 containing a phaR-phaB-phaC-ORF1 operon and a phaQ-phaP-phaJ operon was amplified by PCR using the B. cereus YB-4 genome as a template. Oligonucleotide primers for the PCR amplification were as follows: 5′- atg gga tcc gat gat aca cat cct tat gag tat -3′ and 5′- atg gaa ttc acg gta aac taa gca ata tca ccg -3′ (the underlined sequences denote BamHI and EcoRI sites). Subsequently, the nucleotide fragment was inserted into pHY300PLK (Takara Bio, Otsu, Japan) at the BamHI and EcoRI sites, yielding pHY-phaYB4. In addition, pHY-phaYB4 was digested with PstI and self-ligated after the phaJYB4 fragment was eliminated, yielding pHY-phaYB4ΔphaJ.
For complementation of the deleted phaJYB4 gene, pBBR1-phaJYB4, which is a pBBR1-MCS2-derived plasmidCitation25) carrying phaJYB4 with native ribosome binding sites at the HindIII and BamHI sites, was constructed. Oligonucleotide primers for the PCR amplification of the native ribosome binding site and phaJYB4 were as follows: 5′- cat aag ctt gaa aaa tgt ggg agc taa ctg ca -3′ and 5′- tag gga tcc atg aat cca ttt caa gaa gca c -3′ (the underlined sequences denote HindIII and BamHI sites).
Hydration activity assay
In vitro hydratase assays were performed using the soluble fraction of a recombinant E. coli DH5α strain harboring phaJYB4. The recombinant harboring phaJYB4 in pUC19 was cultured in Luria–Bertani (LB) medium (10 g/L tryptone, 5 g/L yeast extract, and 10 g/L NaCl) at 37 °C. Isopropyl-β-ᴅ-thiogalactopyranoside (IPTG, 1 mM) was added at the beginning of the cultivation for E. coli DH5α strain. After 24 h, cells were harvested by centrifugation and resuspended in 50 mM Tris–HCl buffer (pH 8.0). The cells were lysed by sonication, and the supernatants were used for hydratase activity measurements.
Hydratase activity assays were performed at 30 °C in 50 mM Tris–HCl (pH 8.0) containing 25 μM crotonyl-CoA (Sigma-Aldrich, St. Louis, MO, USA) or octenoyl-CoA.Citation4) A 5-μl aliquot of protein solution was added to quartz cells with a 1.0-cm light path containing enoyl-CoA solution to initiate the hydration reaction. The reaction was monitored using a UV-vis spectrophotometer at 263 nm to measure absorption derived from the enoyl-thioester bond. The ε263 of the enoyl-thioester bond is 6.7 × 103 M−1 cm−1. One unit of hydratase activity was defined as the amount of enzyme required to catalyze a decrease of 1 μmol of substrate in 1 min. Protein concentrations were determined using a Quant-iT™ protein assay kit (Invitrogen, Carlsbad, CA, USA) according to the manufacturer’s instructions.
Production and analysis of PHA
Recombinant strains of E. coli LS5218Citation27) were prepared to verify whether PhaJYB4 can supply monomers for PHA synthesis by modifying β-oxidation products. The plasmid pUC19-phaJYB4 was transformed into E. coli LS5218 together with the plasmid pBBR1-phaRCYB4, which can express the B. cereus YB4 PHA synthase gene (phaRCYB4), or the plasmid pPPAC,Citation27) which can express the Pseudomonas sp. 61-3 PHA synthase gene (phaC1Ps). The recombinant E. coli LS5218 strains were cultured in 100 mL of M9 medium (17.1 g/L Na2HPO4∙12H2O, 3 g/L KH2PO4, 0.5 g/L NH4Cl, 0.5 g/L NaCl, 2 mM MgSO4, and 1 mM CaCl2) containing sodium dodecanoate (2.5 g/L), Brij-35 (0.4% v/v, for the solubilization of dodecanoate), ampicillin (100 mg/L), kanamycin (50 mg/L), and IPTG (1 mM). The cells were cultivated in a 500-ml flask on a reciprocal shaker (130 strokes per min) at 37 °C for 72 h. The content and composition of PHAs in dry cells were determined by gas chromatography after the methanolysis of lyophilized cells in the presence of 15% sulfuric acid, as described previously.Citation28)
Alternatively, to verify PHA accumulation, PHA accumulating-cells were stained with Nile redCitation29) by culturing them on an LB agar plate containing Nile red (0.25 mg/L) and a carbon source (20 g/L glucose or 0.5 g/L octanoate). PHA-accumulating cells were viewed under visible light of 420–500 nm using a Dark Reader® transilluminator (MoBiTec, Göttingen, Germany) with an attached amber screen.
Heterologous expression of pha cluster
To heterologously express the pha cluster of B. cereus YB-4, pHY-phaYB4 or pHY-phaYB4ΔphaJ was introduced into E. coli LS5218. The strain was cultivated in M9 medium containing fatty acid (2.5 g/L), ampicillin (100 mg/L), and tetracycline (10 mg/L) for 72 h at 37 °C. Fatty acids with different chain lengths were added at 0.5 g/L every 12 h until a final concentration of 2.5 g/L was reached, in order to minimize the toxicity of the fatty acids, expect for sodium dodecanoate which was added once at the beginning of cultivation together with Brij-35 (0.4% v/v). The content and composition of PHA in dry cells were also determined by gas chromatography. Genetic complementation of phaJYB4 was conducted by cotransforming pHY-phaYB4ΔphaJ and pBBR-phaJYB4 into E. coli LS5218, and the transformed strain was grown on sodium butyrate or sodium dodecanoate.
Isolation of native PHA granules
Native PHA granules were isolated by the sucrose gradient ultracentrifugation method.Citation30) The recombinant E. coli cells grown in M9 medium containing sodium butyrate (0.5 g/L × 5 times, every 12 h) for 72 h at 37 °C were washed and resuspended in 100 mM Tris–HCl (pH 7.5). The cells were disrupted by sonication. The whole cell extract was loaded onto the top of a discontinuous sucrose gradient of 1.0–2.0 M. After centrifugation (256,000×g, 2.5 h, 4 °C), the layer containing PHA granules was isolated. Finally, the isolated PHA granules were washed twice with the Tris–HCl buffer (pH 7.5).
Sodium dodecyl sulfate-polyacrylamide gel electrophoresis (SDS-PAGE) analysis and N-terminal amino acid determination
SDS-PAGE was performed according to standard procedures using 12.5% gels. The gels were stained with Coomassie Brilliant Blue. To determine the N-terminal amino acid sequence, proteins blotted on polyvinylidene difluoride membranes were sequenced using an automated Edman degradation sequencer.
Results and discussion
B. cereus YB-4 pha cluster
The nucleotide sequence of the pha cluster of B. cereus YB-4 was determined by PCR-based genomic walking. The sequence data have been deposited in the DNA Data Bank of Japan database (accession number AB525763). The structure of the pha cluster of B. cereus YB-4 is shown in Fig. as the B. cereus-type pha cluster. Of seven ORFs found in the pha cluster of B. cereus YB-4, five were identified as phaRYB4, phaBYB4, phaCYB4, phaQYB4, and phaPYB4 as shown in Table , based on their homology with the corresponding genes of B. megaterium.Citation17,31,32) The other two genes are not found in the B. megaterium-type pha cluster.
Table 2. Homology of the gene products of the pha cluster in B. cereus YB-4 to proteins of other Bacillus spp.
The downstream gene (444 bp) of phaPYB4, which is one of the two genes absent from the B. megaterium-type pha cluster, encodes a protein composed of 147 amino acids with a molecular mass of 16,412 Da. The deduced amino acid sequence shows relatively high identities with the R-hydratases of P. aeruginosa (PhaJ1Pa, 41%)Citation3) and A. caviae (PhaJAc, 37%)Citation5); therefore, this gene would be a structural gene of B. cereus R-hydratase (PhaJYB4), and it was referred to as phaJYB4.
B. thuringiensis subsp. chinensis CT-43 also possesses a pha cluster, including a phaJ gene. Additionally, the deduced amino acid sequence of B. thuringiensis PhaJ is the same as that of PhaJYB4. Thus, the PHA metabolism of B. cereus YB-4 and B. thuringiensis subsp. chinensis CT-43 would be very similar. As for B. thuringiensis subsp. chinensis CT-43, a strong expression of phaJ was observed under the PHA accumulation conditions by transcriptional and proteomic analysesCitation33) (Table ). This observation suggests that PhaJ is a component of the pha cluster in B. thuringiensis subsp. chinensis CT-43. Unlike B. megaterium, B. cereus and B. thuringiensis might have acquired the metabolic versatility to supply PHA monomers. The physiological importance of PhaJ is of great interest for understanding PHA metabolism in Bacillus strains.
The other gene absent from the B. megaterium-type pha cluster, ORF1 (1,194 bp), encodes a protein composed of 397 amino acids with a molecular mass of 45,141 Da. The deduced amino acid sequence of ORF1 suggests that it has a glycine/ᴅ-amino acid oxidase domain and shows identity with the ʟ-proline dehydrogenase of Pyrococcus horikoshii (18%)Citation34); however, the product of ORF1 has never been reported to be involved in PHA metabolism. Additionally, as shown in Table , transcriptional and proteomic analyses revealed that the expression level of the gene corresponding to ORF1 in B. thuringiensis subsp. chinensis CT-43 was weak under PHA accumulation conditions.Citation33) Thus, the functional role of ORF1 in PHA metabolism is unknown at this moment.
In vitro activity assay of PhaJ
Qin et al.Citation35) proposed that R-hydratases have a hydratase 2 motif, which has the sequence [YF]-X(1,2)-[LVIG]-[STGC]-G-D-X-N-P-[LIV]-H-X(5)-[AS]. PhaJYB4 has a hydratase 2 motif with the sequence FAKLTGDVNPIHILDSFA. In this study, PhaJYB4 was expressed in E. coli DH5α under the control of lac promoter. As given in Table , high hydratase activity toward crotonyl-CoA was observed, but not toward octenoyl-CoA, for PhaJYB4-expressing strain. In addition, SDS-PAGE analysis showed the corresponding protein band in the soluble fraction of E. coli when PhaJYB4 was expressed (data not shown). These results suggest that PhaJYB4 has a hydratase activity. PhaJYB4 shows a high homology to short chain-length (C4–C6)-specific PhaJs (e.g. P. aeruginosa PhaJ1Pa and A. caviae PhaJAc as described above) rather than medium chain-length (C8–C12)-specific PhaJs (e.g. P. aeruginosa PhaJ4Pa)Citation4); therefore, PhaJYB4 activity is thought to be specific for short chain-length enoyl-CoA. This substrate specificity corresponds to that of PhaRCYB4, which polymerizes short chain length (C4–C5) monomers.Citation19)
Table 3. Enoyl-CoA hydratase activity in recombinant strains of E. coli DH5α.
Monomer-supplying capability of PhaJ in E. coli
The monomer-supplying capability of PhaJYB4 by modifying products of the β-oxidation cycle was assayed in vivo using E. coli LS5218 as a host for PHA accumulation. The results are given in Table .
Table 4. Accumulation of PHA in recombinant E. coli LS5218 harboring a PHA synthase gene (phaRCYB4 or phaC1Ps) with phaJYB4 in the presence of dodecanoate.Table Footnotea
The strain harboring phaRCYB4 alone did not accumulate any PHA, while cells co-expressing phaJYB4 accumulated 40 wt% of P(3HB) from dodecanoate. This result suggests that PhaJYB4 is an R-hydratase and functioned as a monomer supplier by modifying products of the β-oxidation cycle.
In an in vivo assay using the broad substrate-specificity PhaC, the strain harboring phaC1Ps alone accumulated 8 wt% PHA. This is because E. coli inherently has a weak ability to supply medium chain-length monomers by modifying β-oxidation productsCitation36) and Pseudomonas-type PhaCs are likely to accumulate PHA under such conditions.Citation37,38) When co-expressing phaJYB4, the PHA accumulation level was increased to 22 wt%, due to PhaJYB4’s function. As for the comonomer composition, the 3HHx fraction was the highest among 3HA units ranging from C4 to C12, and its relative content reached 63 mol%. This comonomer compositional trend is very similar to that observed when short chain-length specific R-hydratases were expressed.Citation4) Thus, PhaJYB4 was proven to be a short chain-length specific R-hydratase.
Transformation of pha cluster into E. coli LS5218
The function of PhaJYB4 within the pha cluster was assessed by transformation of the pha cluster into E. coli. To this end, the plasmid harboring the pha cluster with or without phaJYB4, pHY-phaYB4 or pHY-phaYB4ΔphaJ, was introduced into E. coli LS5218. The resultant strains were cultured in M9 medium containing various fatty acids. The results are given in Table .
Table 5. Accumulation of PHA in recombinant E. coli LS5218 harboring pHY-phaYB4 or pHYphaYB4ΔphaJ.Table Footnotea
The strain harboring the whole pha cluster accumulated PHA when grown on C4–C12 fatty acids. Due to the narrow substrate specificity of PhaRCYB4,Citation19) the detected 3HA units in PHA were almost all 3HB units. On the other hand, the strain harboring the phaJ-deleted pha cluster did not accumulate PHA. To confirm this result, an experiment with the genetic complementation of phaJYB4 was conducted by reconstructing the pha cluster using two plasmids, pHY-phaYB4ΔphaJ and pBBR-phaJYB4. The phaJYB4-complemented strain synthesised P(3HB) when grown on butyrate and dodecanoate as listed in Table . These results suggest that phaJYB4 in the pha cluster is functional and essential for PHA accumulation from fatty acids.
SDS-PAGE analysis of PHA granule-associated proteins
Native PHA granules were isolated by the sucrose gradient ultracentrifugation method from E. coli LS5218 cells harboring pHY-phaYB4 grown on butyrate. The SDS-PAGE analysis of PHA granule-associated proteins is shown in Fig. . The predicted protein sizes of PHA granule-associated proteins, such as PhaCYB4, PhaPYB4, PhaQYB4, and PhaRYB4 were 41.7, 20.3, 17.2, and 18.5 kDa, respectively, and the corresponding protein bands were detected in SDS-PAGE analysis. Because the bands of PhaQYB4 and PhaRYB4 migrated close together, only the protein bands corresponding to PhaPYB4 and PhaCYB4 were subjected to N-terminal sequencing. The obtained N-terminal sequence of the PhaPYB4 band was METKP, which is identical to the deduced N-terminal sequence of PhaPYB4. Thus, PhaPYB4 was proven to be expressed and present on the surface of PHA granules. Unfortunately, the N-terminal sequencing of PhaCYB4 failed due to a weak signal from the Edman degradation sequencer. Nevertheless, since a substantial accumulation of PHA was detected using several methods, there was overwhelming evidence for PhaCYB4 expression. Taken together, these results support that both operons in the pha cluster were functionally expressed in E. coli cells.
Fig. 3. Sodium dodecyl sulfate-polyacrylamide gel electrophoresis of PHA granule-associated proteins isolated from E. coli LS5218 harboring pHY-phaYB4 cultured in M9 medium containing sodium butyrate (0.5 g/L × 5 times, every 12 h) for 72 h at 37 °C.
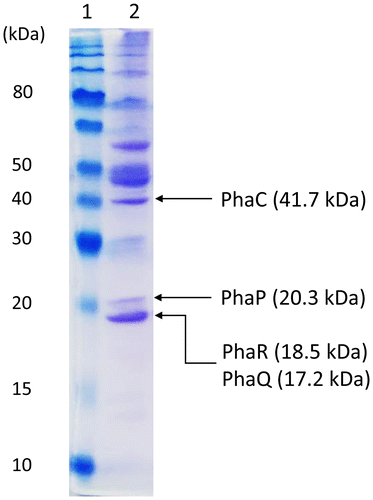
Growth and PHA accumulation of Bacillus spp. on fatty acids
Finally, the cell growth and PHA accumulation of four native strains of Bacillus grown on fatty acids was evaluated. B. thuringiensis HD-1011 and B. megaterium NBRC15308T are both genome-sequenced strains and have the B. cereus-type pha cluster (phaJ present) and the B. megaterium-type pha cluster (phaJ absent), respectively (Fig. ). To this end, B. cereus YB-4, B. thuringiensis HD-1011, and B. megaterium NBRC15308T as PHA-producing strains and B. subtilis ISW1214 as a non-PHA-producing strain were grown on LB-agar plates containing a carbon source (glucose or octanoate) and Nile red. PHA accumulation was observed as shown in Fig. . All tested strains could grow on the glucose plates and all strains except for B. subtilis showed PHA accumulation. On the octanoate plates, B. cereus YB-4 and B. thuringiensis HD-1011 grew and accumulated PHA, which is consistent with a previous study on a B. cereus-relative strain.Citation39) However, B. megaterium and B. subtilis showed poor growth without PHA accumulation. These observations suggest that B. cereus and B. thuringiensis have evolutionarily adapted to utilize and metabolize fatty acids more than B. megaterium has, and one apparent result of this difference is the presence or absence of phaJ in the pha cluster.
Fig. 4. Cell growth and PHA accumulation of Bacillus strains on glucose and octanoate.
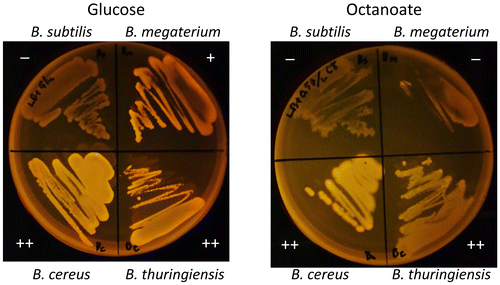
Conclusions
In this study, the pha cluster of B. cereus YB-4 was characterized in terms of PhaJ’s function. In an in vitro activity assay, the crude extract of the PhaJYB4-expressing E. coli strain showed a high hydratase activity toward crotonyl-CoA. The monomer-supplying capability of PhaJYB4 in E. coli was examined and the results showed that PhaJYB4 functioned to supply short chain-length PHA monomers by modifying the products of β-oxidation. Furthermore, the pha cluster from B. cereus YB-4 functioned to accumulate PHA in E. coli; however, it did not function when the phaJYB4 gene was deleted because this caused it to lack a monomer supplier. The analysis of PHA granule-associated proteins demonstrated the expression of PhaPYB4 from the pha cluster and its protein product was localized on the surface of the PHA granules. Bacteria possessing a B. cereus-type pha cluster tended to show better growth on fatty acids and PHA accumulation. These bacteria might have acquired phaJ to improve their fatty acid metabolism during their evolution. This study revealed that the B. cereus-type pha cluster is a new example of a pha cluster that contains the gene encoding PhaJ.
Author contribution
Experimental design and interpretation of data were conducted by all authors. T.K., A.H., and M.I.H. carried out experiments. K.M. and T.T. planned the project. T.K. and T.T. wrote the paper with input from the co-authors, and the other authors reviewed the manuscript.
Funding
This work was partially supported by JST CREST [grant number JPMJCR12B4], Japan.
Disclosure statement
No potential conflict of interest was reported by the authors.
References
- Sudesh K, Abe H, Doi Y. Synthesis, structure and properties of polyhydroxyalkanoates: biological polyesters. Prog Polym Sci. 2000;25:1503–1555.
- Tsuge T, Hyakutake M, Mizuno K. Class IV polyhydroxyalkanoate (PHA) synthases and PHA-producing Bacillus. Appl Microbiol Biotechnol. 2015;99:6231–6240.10.1007/s00253-015-6777-9
- Tsuge T, Fukui T, Matsusaki H, et al. Molecular cloning of two (R)-specific enoyl-CoA hydratase genes from Pseudomonas aeruginosa and their use for polyhydroxyalkanoate synthesis. FEMS Microbiol Lett. 2000;184:193–198.10.1111/fml.2000.184.issue-2
- Tsuge T, Taguchi S, Taguchi K, et al. Molecular characterization and properties of (R)-specific enoyl-CoA hydratases from Pseudomonas aeruginosa: metabolic tools for synthesis of polyhydroxyalkanoates via fatty acid ß-oxidation. Int J Biol Macromol. 2003;31:195–205.10.1016/S0141-8130(02)00082-X
- Fukui T, Doi Y. Cloning and analysis of the poly(3-hydroxybutyrate-co-3-hydroxyhexanoate) biosynthesis genes of Aeromonas caviae. J Bacteriol. 1997;179:4821–4830.
- Fukui T, Shiomi N, Doi Y. Expression and characterization of (R)-specific enoyl coenzyme A hydratase involved in polyhydroxyalkanoate biosynthesis by Aeromonas caviae. J Bacteriol. 1998;180:667–673.
- Reiser SE, Mitsky TA, Gruys KJ. Characterization and cloning of an (R)-specific trans-2,3-enoylacyl-CoA hydratase from Rhodospirillum rubrum and use of this enzyme for PHA production in Escherichia coli. Appl Microbiol Biotechnol. 2000;53(2):209–218.10.1007/s002530050010
- Mothes G, Babel W. Methylobacterium rhodesianum MB 126 possesses two stereospecific crotonyl-CoA hydratases. Can J Microbiol. 1995;41:68–72.10.1139/m95-170
- Liu G, Cai S, Hou J, et al. Enoyl-CoA hydratase mediates polyhydroxyalkanoate mobilization in Haloferax mediterranei. Sci Rep. 2016;6:24015.
- Fiedler S, Steinbüchel A, Rehm BH. The role of the fatty acid β-oxidation multienzyme complex from Pseudomonas oleovorans in polyhydroxyalkanoate biosynthesis: molecular characterization of the fadBA operon from P. oleovorans and of the enoyl-CoA hydratase genes phaJ from P. oleovorans and Pseudomonas putida. Arch Microbiol. 2002;178:149–160.10.1007/s00203-002-0444-0
- Sato S, Kanazawa H, Tsuge T. Expression and characterization of (R)-specific enoyl coenzyme A hydratases making a channeling route to polyhydroxyalkanoate biosynthesis in Pseudomonas putida. Appl Microbiol Biotechnol. 2011;90:951–959.10.1007/s00253-011-3150-5
- Vo MT, Lee KW, Jung YM, et al. Comparative effect of overexpressed phaJ and fabG genes supplementing (R)-3-hydroxyalkanoate monomer units on biosynthesis of mcl-polyhydroxyalkanoate in Pseudomonas putida KCTC1639. J Biosci Bioeng. 2008;106:95–98.10.1263/jbb.106.95
- Kawashima Y, Cheng W, Mifune J, et al. Characterization and functional analyses of R-specific enoyl coenzyme A hydratases in polyhydroxyalkanoate-producing Ralstonia eutropha. Appl Environ Microbiol. 2012;78(2):493–502.10.1128/AEM.06937-11
- Chung MG, Rhee YH. Overexpression of the (R)-specific enoyl-CoA hydratase gene from Pseudomonas chlororaphis HS21 in Pseudomonas strains for the biosynthesis of polyhydroxyalkanoates of altered monomer composition. Biosci Biotechnol Biochem. 2012;76:613–616.10.1271/bbb.110871
- Davis R, Chandrashekar A, Shamala TR. Role of (R)-specific enoyl coenzyme A hydratases of Pseudomonas sp. in the production of polyhydroxyalkanoates. Antonie van Leeuwenhoek. 2008;93(3):285–296.10.1007/s10482-007-9203-1
- Lu XY, Wu Q, Zhang WJ, et al. Molecular cloning of polyhydroxyalkanoate synthesis operon from aeromonas hydrophila and its expression in Escherichia coli. Biotechnol Prog. 2004;20(5):1332–1336.10.1021/bp0499202
- McCool GJ, Cannon MC. Polyhydroxyalkanoate inclusion body-associated proteins and coding region in Bacillus megaterium. J Bacteriol. 1999;181:585–592.
- Mizuno K, Ohta A, Hyakutake M, et al. Isolation of polyhydroxyalkanoate-producing bacteria from a polluted soil and characterization of the isolated strain Bacillus cereus YB-4. Polym Degrad Stab. 2010;95:1335–1339.10.1016/j.polymdegradstab.2010.01.033
- Hyakutake M, Saito Y, Tomizawa S, et al. Polyhydroxyalkanoate (PHA) synthesis by class IV PHA synthases employing Ralstonia eutropha PHB−4 as host strain. Biosci Biotechnol Biochem. 2011;75:1615–1617.10.1271/bbb.110229
- Tomizawa S, Hyakutake M, Saito Y, et al. Molecular weight change of polyhydroxyalkanoate (PHA) caused by the PhaC subunit of PHA synthase from Bacillus cereus YB-4 in recombinant Escherichia coli. Biomacromolecules. 2011;12(7):2660–2666.10.1021/bm2004687
- Hyakutake M, Tomizawa S, Mizuno K, et al. Alcoholytic cleavage of polyhydroxyalkanoate chains by class IV synthases induced by endogenous and exogenous ethanol. Appl Environ Microbiol. 2014;80:1421–1429.10.1128/AEM.03576-13
- Hyakutake M, Tomizawa S, Mizuno K, et al. A common active site of polyhydroxyalkanoate synthase from Bacillus cereus YB-4 is involved in polymerization and alcoholysis reactions. Appl Microbiol Biotechnol. 2015;99(11):4701–4711.10.1007/s00253-014-6276-4
- Hyakutake M, Tomizawa S, Sugahara I, et al. Carboxy-terminal modification of polyhydroxyalkanoate (PHA) via alcoholysis reaction catalyzed by class IV PHA synthase. Polym Degrad Stab. 2015;117:90–96.10.1016/j.polymdegradstab.2015.04.002
- Tsuge T. Fundamental factors determining the molecular weight of polyhydroxyalkanoate during biosynthesis. Polym J. 2016;48:1051–1057.10.1038/pj.2016.78
- Kovach ME, Elzer PH, Hill DS, et al. Four new derivatives of the broad-host-range cloning vector pBBR1MCS, carrying different antibiotic-resistance cassettes. Gene. 1995;166:175–176.10.1016/0378-1119(95)00584-1
- Jenkins LS, Nunn WD. Genetic and molecular characterization of the genes involved in short-chain fatty acid degradation in Escherichia coli: the ato system. J Bacteriol. 1987;169:42–52.10.1128/jb.169.1.42-52.1987
- Taguchi K, Aoyagi Y, Matsusaki H, et al. Co-expression of 3-ketoacyl-ACP reductase and polyhydroxyalkanoate synthase genes induces PHA production in Escherichia coli HB101 strain. FEMS Microbiol Lett. 1999;176:183–190.10.1111/fml.1999.176.issue-1
- Kato M, Bao HJ, Kang CK, et al. Production of a novel copolyester of 3-hydroxybutyric acid and medium-chain-length 3-hydroxyalkanoic acids by Pseudomonas sp. 61-3 from sugars. Appl Microbiol Biotechnol. 1996;45:363–370.10.1007/s002530050697
- Spiekermann P, Rehm BH, Kalscheuer R, et al. A sensitive, viable-colony staining method using Nile red for direct screening of bacteria that accumulate polyhydroxyalkanoic acids and other lipid storage compounds. Arch Microbiol. 1999;171:73–80.10.1007/s002030050681
- Tsuge T, Takase K, Taguchi S, et al. An extra large insertion in the polyhydroxyalkanoate synthase from Delftia acidovorans DS-17: its deletion effects and relation to cellular proteolysis. FEMS Microbiol Lett. 2004;231:77–83.10.1016/S0378-1097(03)00930-3
- McCool G, Cannon MC. PhaC and PhaR are required for polyhydroxyalkanoic acid synthase activity in Bacillus megaterium. J Bacteriol. 2001;183:4235–4243.10.1128/JB.183.14.4235-4243.2001
- Lee TR, Lin JS, Wang SS, et al. PhaQ, a new class of poly-β-hydroxybutyrate (PHB)-responsive repressor, regulates phaQ and phaP (phasin) expression in Bacillus megaterium through interaction with PHB. J Bacteriol. 2004;186:3015–3021.10.1128/JB.186.10.3015-3021.2004
- Wang J, Mei H, Zheng C, et al. The metabolic regulation of sporulation and parasporal crystal formation in Bacillus thuringiensis revealed by transcriptomics and proteomics. Mol Cell Proteomics. 2013;12:1363–1376.10.1074/mcp.M112.023986
- Tsuge H, Kawakami R, Sakuraba H, et al. Crystal structure of a novel FAD-, FMN-, and ATP-containing L-proline dehydrogenase complex from Pyrococcus horikoshii. J Biol Chem. 2005;280:31045–31049.10.1074/jbc.C500234200
- Qin YM, Haapalainen AM, Kilpeläinen SH, et al. Human peroxisomal multifunctional enzyme type 2. J Biol Chem. 2000;275:4965–4972.10.1074/jbc.275.7.4965
- Park SJ, Lee SY. Identification and characterization of a new enoyl coenzyme A hydratase involved in biosynthesis of medium-chain-length polyhydroxyalkanoates in recombinant Escherichia coli. J Bacteriol. 2003;185:5391–5397.10.1128/JB.185.18.5391-5397.2003
- Langenbach S, Rehm BH, Steinbuchel A. Functional expression of the PHA synthase gene phaC1 from Pseudomonas aeruginosa in Escherichia coli results in poly(3-hydroxyalkanoate) synthesis. FEMS Microbiol Lett. 1997;150:303–309.10.1016/S0378-1097(97)00142-0
- Tsuge T, Sato S, Hiroe A, et al. Contribution of the distal pocket residue to the acyl-chain-length specificity of (R)-specific enoyl-coenzyme A hydratases from Pseudomonas spp. Appl Environ Microbiol. 2015;81:8076–8083.10.1128/AEM.02412-15
- Tajima K, Igari T, Nishimura D, et al. Isolation and characterization of Bacillus sp. INT005 accumulating polyhydroxyalkanoate (PHA) from gas field soil. J Biosci Bioeng. 2003;95:77–81.10.1016/S1389-1723(03)80152-4