Abstract
Conjugated linoleic acid (CLA) has attracted as novel type of fatty acids having unusual health-promoting properties such as anticarcinogenic and antiobesitic effects. The present work employed castor oil as substrate for one-pot production of CLA using washed cells of Lactobacillus plantarum (L. plantarum) and lipases as catalysts. Among the screened lipases, the lipase Rhizopus oryzae (ROL) greatly assisted resting cells to produce CLA. Mass spectral analysis of the product showed that two major isomers of CLA were produced in the reaction mixture i.e. cis-9, trans-11 56.55% and trans-10, cis-12 43.45%. Optimum factors for CLA synthesis were found as substrate concentration (8 mg/mL), pH (6.5), washed cell concentration (12% w/v), and incubation time of 20 h. Hence, the combination of ROL with L. plantarum offers one pot production of CLA selectively using castor oil as a cost-effective substrate.
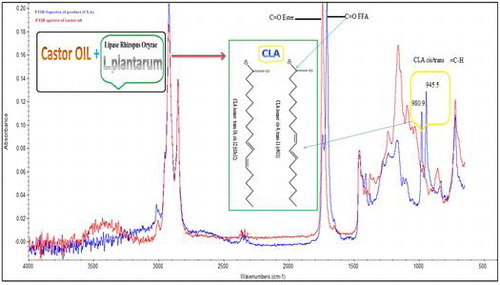
Conjugated linoleic acid (CLA) is a group of positional and geometrical isomers of linoleic acid. Naturally consumed sources of CLA are milk and dairy products of ruminant origin. Among all the CLA isomers, the cis-9, trans-11 and trans-10, cis-12 are major ones studied for the anticancer and body fat reducing properties.Citation1,2) Despite its nutritional benefits, CLA appears naturally at levels between 0.3 and 0.8% in beef and dairy products which is much lower than the recommended value as 3 g day−1.Citation1) Therefore, it is necessary to produce the desired physiological effects using specific CLA isomers without consuming undesirable amounts of saturated fats and cholesterol. For this reason, an alternative source that contains lower amount of saturated fat and cholesterol with high levels of CLA would be an excellent addition to the human diet.
At present, CLA supplements used by consumers are synthesized through chemical isomerization, in which undesirable mixture of CLA isomers is produced. To use CLA for nutraceutical and medicinal purposes, an isomer selective production is highly needed.Citation3) Biocatalysts are advantageous to chemical synthesis in that, the whole micro-organisms or purified enzymes are highly selective in the production of desired isomers. In our previous study, L. plantarum selectively isomerized linoleic acid into two major CLA isomers using linoleic acid as a substrate.Citation4) Castor oil is considered as a unique type of vegetable oil due to the presence of a hydroxy fatty acid i.e. ricinoleic acid (12-hydroxy-cis-9-octadecenoic acid) which constitutes 90% composition of castor oil.Citation5,6) Lactic acid bacteria do not isomerize triglyceride form of ricinoleic acid into CLA from castor oil; therefore, different lipases were used to make castor oil suitable substrate for CLA synthesis.Citation7) Different organisms of various genera such as Lactobacillus, Propionibacterium, and Butyrivibrio produced CLA in different amounts.Citation8) These bacteria produce CLA from polyunsaturated fatty acids as result of biohydrogenation to detoxify their living environment. Lactic acid bacteria can produce CLA from ricinoleic acid by two potential mechanisms i.e. direct conversion of ricinoleic acid into CLA by dehydration at Δ11 and second pathway involves the transformation of ricinoleic into linoleic acid followed by isomerization of linoleic acid into CLA.Citation9) The presence of linoleic acid and 10-hydroxy-18:1 in the reaction mixture with ricinoleic acid as the substrate for CLA production precedes through the latter pathway.Citation3)
It was first time that we used ROL for the hydrolysis of castor oil up to 90% free fatty acids (FFA) yield, which is the first step toward the biosynthesis of CLA using castor oil as a substrate.Citation11) The aim of the current study was to use one-pot strategy for the production of bioactive CLA isomers from castor oil as a cost-effective substrate by catalytic abilities of probiotic L. plantarum strain with lipase-assisted hydrolysis.
Material and methods
Chemicals
Castor oil was purchased from local market of Hyderabad, Sindh. Lipase Rhizopus oryzae, lipase Candida cylindracea and CLA standard mixture (cis-9, trans-11 trans-10, cis-12) were purchased from Sigma Aldrich Germany. Lipase OF from Candida rugosa, lipase from Mycobacterium, and lipase SL from Pseudomonas (Burkholderia) cepacia were obtained from Meito Sangyo Co. Ltd Japan. Methanol and Tween-80 were used for subsequent experiments.
Cultivation of bacteria and preparation of washed cells
The previously identified L. plantarumCitation4) strain was grown in MRS medium at 37 °C for 24 h with shaking speed (120 rpm). Cells were harvested by centrifugation (8000 rpm, 10 min), washed twice with sodium chloride (0.85%) solution then centrifuged again. The washed cells were used for the CLA production at various concentrations.
Biocatalysis protocol for CLA production
The reaction mixture contained castor oil (2–12 mg/mL), 0.1% Tween-80, 1 mL phosphate buffer solution (0.1 M, pH 5–7.5), and 0.1 mL of lipase solution (0.2 mg/mL). The reaction mixture was sonicated for 2 min after that the washed cells (2–14%) were mixed thoroughly in a 10-mL test tube. Then reaction mixture was kept on a shaking speed of 150 rpm for various time intervals (5–35 h) at 37 °C. All the reactions were carried out in triplicates under aerobic conditions.
Quantification of FFA
To determine the degree of castor oil hydrolysis, FFA content was quantified as reported earlier,Citation11) utilizing Attenuated total reflectance Fourier-transform infrared (ATR-FTIR) spectroscopic method. Briefly, a calibration curve was constructed using partial least square (PLS) model by gravimetric addition of oleic acid (10–50%) in castor oil to detect the carbonyl absorption of FFA in the region (1690–1730 cm−1). After completion of reaction, the mixture was centrifuged at 8000 rpm (5152 × g) to separate the water and oil phases. Hundred microliters of reaction mixture was taken from the upper oil layer, that was placed over ATR crystal and the FFA% content was determined from calibration curve obtained using the PLS model.
Extraction of fatty acids
At the end of reaction protocol, fatty acids were separated by adding chloroform/methanol (1:2 v/v) according to the procedure of Bligh and Dyer.Citation12)
UV–vis spectrophotometric monitoring and quantification of CLA
The product formation was monitored by UV/visible spectrophotometer (Biochrom Libra S22) at 233 nm, a particular wavelength for CLA confirmation.Citation13) Absorbance was measured in 1-cm quartz cuvettes at ambient conditions. The quantitation of the reaction product was done with straight line equation drawn from the calibration curve of CLA standard mixture.
FTIR instrumentation and analysis
FTIR analysis of the samples was carried out on a Thermo Nicolet 5700 FTIR spectrometer (Thermo Nicolet Analytical Instruments, Madison, WI) equipped with a pyroelectric deuterated triglycine sulfate (DTGS) detector. An ATR accessory with a removable ZnSe crystal was mounted in the sample compartment. The FTIR was controlled by OMNIC software (version 7.2) and analysis was carried out in the range between 4000 and 650 cm−1 by addition of 32 scans at a resolution of 4 cm−1. A few microliters of the sample mixture are enough to acquire the infrared spectrum of the sample.
Preparation of fatty acid methyl esters
Fatty acid samples were first subjected for saponification by adding 1-mL methanolic sodium hydroxide solution (0.5 N) and incubated at 100 °C for 10 min. After saponification, the reaction mixture was cooled at room temperature. In the next step, 1 mL of BF3-methanol (20% w/w) was added to the reaction mixture. The samples were incubated for 2 min (100 °C), after cooling 1 mL of n-hexane was mixed and samples were vortexed and reheated for 1 min. Finally, 1-mL saturated solution of sodium chloride was added to ensure the complete separation and transfer of the hexane phase containing the methyl esters.Citation14)
Formation of 4-methyl-1,2,4-triazoline-3,5-dione (MTAD) CLA adducts
MTAD derivatives were prepared by the Dobson method.Citation15) Solutions of CLA methyl ester (220 μg, 1.15 mM) and MTAD (425 μg, 5.79 mM) in dichloromethane (650 μL) were mixed in a test tube at 0 °C by agitating for less than 10 s. The molar ratio of conjugated fatty acid methyl ester to MTAD was 1:5. The reactions were immediately stopped by the addition of a twofold molar excess (relative to MTAD) of 1,3-hexadiene, followed by agitation for a few seconds. The mixtures were taken to dryness and reconstituted in dichloromethane to give approximately 0.1% (w/v) solutions of the fatty acid methyl ester adducts. Adducts were analyzed directly by GC–MS on a nonpolar capillary column DB-5. Ratios of the corresponding CLA isomers were calculated based on peak area of each CLA isomer divided by the total peak area of all CLA isomers. For percent ratios, obtained values were multiplied with 100 as reported earlier.Citation16)
GC-MS conditions for the separation of CLA-MTAD adducts
The GC-MS analysis of the CLA-MTAD adducts was carried out on a GCMS-QP2010 Plus with ion source of 70 eV. The separation of CLA-MTAD adducts was done on DB-5 30 m long capillary column having 0.25 mm internal diameter and 0.25 μm thickness. Initially, oven temperature was 160 °C for 13 min, followed by a ramp of 5 °C /min to reach at 350 °C. Helium was used as carrier gas and flow rate was 1 mL min−1. Ion source and interface temp were 250 °C and scanning range was 35–750 amu at (0.44) scans/Sec.
Statistical analysis
Data were statistically evaluated by one-way ANOVA test using SAS 16.0 (SAS Inst. Inc., Cary, N.C., U.S.A.) at the significance level of p < 0.05. The reported results were expressed as mean ± standard deviation (SD) of triplicate samples.
Results and discussion
Selection of lipases for CLA production
Castor oil is not a suitable substrate in triglyceride form for lactic acid bacteria to convert it into CLA; however, it can be transformed into a proper starting material for CLA synthesis when exposed to lipase hydrolysis.Citation17) Fig. shows that five different lipases (lipase OF, lipase candida cylendercia, lipase mycobacterium, lipase SL, and ROL) were used along with L. plantarum. It can be seen from the figure that highest amount of CLA was produced with the lipase ROL because of its higher ability to hydrolyze (FFA 90.56%) the castor which assisted the L. plantarum to produce CLA.Citation11) Therefore, further study was carried out with ROL.
Effect of castor oil concentration on CLA production
Various concentrations of castor oil (2–12 mg mL−1) were used in the reaction protocol for CLA synthesis. Fig. shows that CLA formation and FFA% increased with increasing the substrate amount and maximum CLA yield (316 μg/mL) was achieved at 8 mg of castor oil. Further increasing the substrate concentration did not confer positive effect on CLA yield possibly due to the saturation of enzyme active sites with substrate.
Effect of pH on CLA yield
Biocatalytic reactions are mostly affected by a small variation of pH in the reaction mixture because each enzyme works best at its optimum value.Citation7,18) Therefore, the impact of variable pH was studied for CLA conversion from castor. As depicted in Fig. CLA production increased regularly from pH 5 to 6, the higher yield of CLA was observed when reaction pH was kept 6.5. While FFA% increased from pH 5.5–7.0 and no further increased was observed beyond that pH value. Keeping in view the optimum CLA production at pH 6.5, it was considered for further optimization.
Effect of incubation time on CLA yield
Incubation time of CLA synthesis by L. plantarum was kept from 5 to 35 h at an interval of 5 h. Fig. shows an increase in CLA yield and amount of FFA from 5 to 15 h and maximum yield of CLA was detected after 20 h of incubation. The prolonged incubation did not cause a further increase in CLA yield because LA isomerase did not recycle like a normal enzyme to catalyze more substrate, into CLA.Citation19) Therefore, 20 h was the optimum incubation period for further experiments for CLA synthesis.
Effect of washed cell concentration on CLA yield
Cellular concentration has direct relationship to the enzyme concentration which is directly proportional to the rate of reaction. The washed cell concentration was kept between (2–14% w/v). As shown in the Fig. increasing the washed cell amount conferred the positive effect on CLA yield and reached maximum (406 μg mL−1) at 12% w/v. Further, addition of washed cells didn’t increase the CLA yield. It is probably that CLA production depends on washed cell density and high cell concentration brings about the mass transfer limitation due to high viscosity as reported earlier by Zhao et al.Citation20) and li et al.Citation21)
Analysis of the product (CLA) by spectroscopic techniques
CLA contain two double bonds in a conjugated manner, Fig. (a) shows the absorption band of CLA isomers appearing at 233 nm. UV–vis spectroscopy offers a rapid analysis method for the determination of CLA in the reaction mixture when large number of optimization and screening of CLA producing species is required. The samples were scanned from 200 to 400 nm and the quantitation of total CLA was done by putting the absorbance value in straight line equation.Citation4)
Fig. 6. (a) Monitoring of the CLA formation by UV-Visible spectroscopy (b) FT-IR analysis of the castor oil before and after one pot biocatalysis using ROL and L. plantaram at optimized conditions.
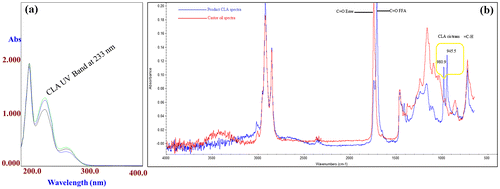
The geometrical isomers of CLA have a “fingerprint” absorption peaks in the mid-IR spectrum, the trans/trans isomers absorb at 988 cm−1. While the cis/cis and cis/trans CLA isomers produce two prominent peaks at 981 and 947 cm−1, respectively. The changes in the intensities of the CLA absorptions at 981 and 944 cm−1 are due to the sloping of the spectral region (1000–900 cm−1).Citation22) Fig. (b) shows the FTIR spectra of the product and castor oil. The two peaks at 980.9 and 945.5 cm−1 are due to the CH out-of-plane bending vibration, which are characteristics of a mono-trans CLA. In addition, the appearance of FFA, C = O band at 1708.7 cm−1 in the product spectrum, which clearly shows the hydrolysis of castor oil by ROL.Citation11) Other peaks in the FTIR spectrum were, 2923.cm−1 (asymmetric –CH2 stretch), 2853.4 cm−1 (symmetric –CH2 stretch), 1456.9 cm−1 (asymmetric –CH3 bending), and 723 cm−1 (–CH2 rocking vibration). The small peak at 3019 cm−1 represents the cis double bond stretching of the unsaturated fatty acid.Citation23)
Structural analysis of CLA using Diels Alder reagent (MTAD)
For the confirmation of the positions of the conjugated double bonds in CLA, a simple method is to yield Diels–Alder adducts with 4-methyl-1,2,4-triazoline-3,5-dione (MTAD), which reacts selectively and instantaneously with conjugated systems at 0 °C.Citation24) Fig. shows the mass spectra of two major bioactive CLA isomers with MTAD adducts. The molecular ion peak (M+) of MTAD-CLA derivatives appears at m/z 407. The mass spectrum of each isomer gives a very simple and informative spectral pattern, subjugated by two intense ions, specific of each positional isomer, formed by α-cleavage of the side chains around the bicyclic ring structure. In Fig. (a), the abundant fragments at m/z 322 ([M – 85]+) and 250 ([M – 157]+) are formed as a result of α-cleavage to the ring structure, showing that the original conjugated double bonds at 9th and 11th carbon. The large peak at m/z 290 represents loss of methanol from the ion at m/z = 322. In the second spectrum Fig. (b), two abundant peaks at m/z 336 ([M – 71]+) and 236 ([M – 171]+) are confirming that the double bond positions were at 10th and 12th carbons in the CLA isomer. Two noticeable ion peaks (m/z 304 and 376) in the second spectra, were accounted for, by the loss of methanol ([M – R1]+) and methoxy radical (from ester substituent) from the molecular ion, respectively. The appearance of ion peak at m/z 166 in both spectra was due to the loss of both side chains from the CLA-MTAD adducts. We have identified the CLA isomers based on retention time and matching mass spectra of product and analytical standards of CLA. Further from the literature,Citation24,25) it can be assumed that major CLA isomers are cis-9, trans-11 and trans-10,cis-12. From the peak area calculations of CLA isomers, it was found that cis-9, trans-11 accounted for 56.55% of total CLA, while 43.45% was contributed by trans-10, cis-12 isomer.
Fig. 7. Mass spectra of CLA adducts with 4-methyl-1,2,4-triazoline-3,5-dione (a) Methyl 9 cis,11trans-octadecadienoate and (b) Methyl 10 trans, 12cis octadecadienoate isomers.
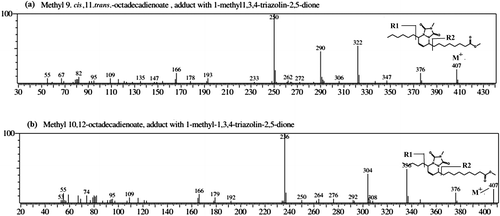
Similar to our findings, Hosseini et al.Citation18) have reported that biological CLA production from castor oil by lactic acid bacteria can be much more isomer selective than chemical methods. The authors revealed that produced CLA was a mixture of two bioactive isomers including cis-9, trans-11 and trans-10, cis-12-CLA.
Conclusions
Lipase Rhizopus oryzae along with washed cells of Lactobacillus plantarum offers one pot production of two major bioactive CLA isomers selectively. Spectroscopic and MTAD derivative analysis of the product confirmed the formation of cis-9, trans-11 and trans-10, cis-12 isomers. Under mild experimental conditions, optimum production of CLA (406 μg/mL) from castor oil was achieved with phosphate buffer (pH 6.5, 0.2 M) in 20 h of incubation. Hence, the current study offers a one-pot synthesis of two major bioactive CLA isomers using the combination of lipase and washed cells along with castor oil as cost effective substrate.
Authors contributions
AAK, FNT, and AC planned the experimental work. AAK conducted the biotransformation reactions with the assistance of FNT and AC. While SJ, MAS, and HIA helped in GC-MS, UV-Visible, and FTIR analysis. AAK and FNT prepared the manuscript draft and all authors approved the manuscript.
Disclosure statement
No potential conflict of interest was reported by the authors.
Acknowledgments
Abid Ali Khaskheli greatly acknowledges the National Centre of Excellence in Analytical Chemistry, University of Sindh for providing monthly stipend to carry out this research work. No further funding was available.
Notes
Abbreviations: CLA, linoleic acid; ROL, Rhizopus oryzae; L. plantarum, Lactobacillus plantarum.
References
- Bulut S, Bodur E, Colak R, et al. Effects of conjugated linoleic acid supplementation and exercise on post-heparin lipoprotein lipase, butyrylcholinesterase, blood lipid profile and glucose metabolism in young men. Chem Biol Interact. 2013;203:323–329.10.1016/j.cbi.2012.09.022
- Watras AC, Buchholz AC, Close RN, et al. The role of conjugated linoleic acid in reducing body fat and preventing holiday weight gain. Int J Obes. 2007;31:481–487.10.1038/sj.ijo.0803437
- Griinari JM, Corl BA, Lacy SH, et al. Conjugated linoleic acid is synthesized endogenously in lactating dairy cows by Delta(9)-desaturase. J Nutr. 2000;130:2285–2291.
- Khaskheli AA, Talpur FN, Demir AS, et al. A highly selective whole cell biocatalysis method for the production of two major bioactive conjugated linoleic acid isomers. Biocatal Agric Biotechnol. 2013;2:328–332.10.1016/j.bcab.2013.06.004
- Mutlu H, Meir MAR. Castor oil as a renewable resource for chemical industry. Eur J Lipid Sci Technol. 2010;112:10–30.
- Naughton FC. Production, chemistry and commercial applications of various chemicals from castor oil. J Am Oil Chem Soc. 1974;51:65–71.
- Ando A, Ogawa J, Kishino S, et al. Conjugated linoleic acid production from castor oil by Lactobacillus plantarum JCM 1551. Enzyme Microb Technol. 2004;35:40–45.10.1016/j.enzmictec.2004.03.013
- Dubey V, Ghosh AR, Mandal BK. Appraisal of conjugated linoleic acid production by probiotic potential of Pediococcus spp. GS4. Appl Biochem Biotechnol. 2012;168:1265–1276.10.1007/s12010-012-9855-9
- Corl BA, Baumgard LH, Dwyer DA, et al. The role of Delta(9)-desaturase in the production of cis-9, trans-11 CLA. J Nutr Biochem. 2001;12:622–630.10.1016/S0955-2863(01)00180-2
- Sels B, Philippaerts A. Conjugated linoleic acids and conjugated vegetable oils. In: Ogawa J, Takeuchi M, Kishino S, editors. Recent advances in the production of CLA and conjugated vegetable oils: microbial and enzymatic production of conjugated fatty acids and related fatty acids in biohydrogenation metabolism. London: Royal Society of Chemistry; 2014. p. 131–150.
- Khaskheli AA, Talpur FN, Ashraf MA, et al. Monitoring the Rhizopus oryzae lipase catalyzed hydrolysis of castor oil by ATR-FTIR spectroscopy. J Mol Catal B Enzym. 2015;113:56–61.10.1016/j.molcatb.2015.01.002
- Bligh EG, Dyer WJ. A rapid method of total lipid extraction and purification. Can J Biochem Physiol. 1959;37:911–917.10.1139/o59-099
- Barrett E, Ross R, Fitzgerald G, et al. Rapid screening method for analyzing the conjugated linoleic acid production capabilities of bacterial cultures. Appl Environ Microbiol. 2007;73:2333–2337.10.1128/AEM.01855-06
- Park SJ, Park CW, Kim SJ, et al. Methylation methods for the quantitative analysis of conjugated linoleic acid (CLA) isomers in various lipid samples. J Agric Food Chem. 2002;50:989–996.10.1021/jf011185b
- Dobson G. Identification of conjugated fatty acids by gas chromatography-mass spectrometry of 4-methyl-1,2,4-triazoline-3,5-dione adducts. J Am Oil Chem Soc. 1998;75:137–142.10.1007/s11746-998-0024-9
- Dodds ED, McCoy MR, Rea LD, et al. Gas chromatographic quantification of fatty acid methyl esters: flame ionization detection vs. electron impact mass spectrometry. Lipids. 2005;40:419–428.10.1007/s11745-006-1399-8
- Kishino S, Ogawa J, Ando A, et al. Ricinoleic acid and castor oil as substrates for conjugated linoleic acid production by washed cells of lactobacillus plantarum. Biosci Biotechnol Biochem. 2002;66:2283–2286.10.1271/bbb.66.2283
- Hosseini ES, Kermanshahi RK, Hosseinkhani S, et al. onjugated linoleic acid production from various substrates by probiotic Lactobacillus plantarum. Ann Microbiol. 2015;65:27–32.10.1007/s13213-014-0832-0
- Kim YJ, Liu RH, Bond DR, et al. Effect of linoleic acid concentration on conjugated linoleic acid production by butyrivibrio fibrisolvens A38. Appl Environ Microbiol. 2000;66:5226–5230.10.1128/AEM.66.12.5226-5230.2000
- H-w Zhao, J-p Lv, Li SR. Production of conjugated linoleic acid by whole-cell of Lactobacillus plantarum A6-1F. Biotechnol Biotechnol Equip. 2011;25:2266–2272.
- Li H, Li Z, Ruan G, et al. Asymmetric reduction of acetophenone into R-(+)-1-phenylethanol by endophytic fungus Neofusicoccum parvum BYEF07 isolated from Illicium verum. Biochem Biophys Res Commun. 2016;473:874–878.10.1016/j.bbrc.2016.03.142
- Kadamne JV, Castrodale CL, Proctor A. Measurement of conjugated linoleic acid (CLA) in CLA-rich potato chips by ATR-FTIR spectroscopy. J Agric Food Chem. 2011;59:2190–2196.10.1021/jf104204e
- Kadamne JV, Jain VP, Saleh M, et al. Measurement of conjugated linoleic acid (CLA) in CLA-rich soy oil by attenuated total reflectance−fourier transform infrared spectroscopy (ATR−FTIR). J Agric Food Chem. 2009;57:10483–10488.10.1021/jf902445v
- Dobson G. Identification of conjugated fatty acids by gas chromatography-mass spectrometry of 4-methyl-1,2,4-triazoline-3,5-dione adducts. J Am Oil Chem Soc. 1998;75:137–142.10.1007/s11746-998-0024-9
- Berdeaux O, Christie WW, Gunstone FD, et al. Large-scale synthesis of methyl cis-9, trans-11-octadecadienoate from methyl ricinoleate. J Am Oil Chem Soc. 1997;74:1011–1015.10.1007/s11746-997-0018-z