Abstract
In order to investigate the intermediacy of 2-(4-methylpent-3-en-1-yl)anthraquinone (MPAQ), a possible intermediate for the biosynthesis of anthraquinone derivatives in sesame (Sesamum indicum), 2H-labeled MPAQ was administered to a hairy root culture of S. indicum. Efficient conversion of fed MPAQ to 2-[(Z)-4-methylpenta-1,3-dien-1-yl]anthraquinone ((Z)-MPDEAQ) was observed. Furthermore, administration experiment with 2H-labeled 2-geranyl-1,4-naphthohydroquinone, another possible intermediate, showed that it was converted to MPAQ and (Z)-MPDEAQ. The results clearly demonstrated that these substrates are the actual precursors for the production of (Z)-MPDEAQ. In contrast, neither MPAQ nor 2-geranyl-1,4-naphthohydroquinone was converted to anthrasesamone B and 2,3-epoxyanthrasesamone B, other anthraquinone derivatives in the hairy roots, suggesting that these substrates may not be the common precursors in the biosynthesis of anthraquinone derivatives.
Biosynthetic pathway of two anthraquinone derivatives (1 and 2) from 2-geranyl-1,4-naphthohydroquinone (7) in sesame hairy roots.
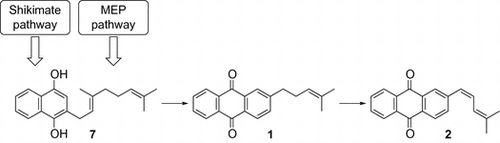
Sesame (Sesamum indicum L., Pedaliaceae) is a cultivated species that is one of the most important crops throughout the world, and its seeds have been considered a valuable health food and have been utilized for millennia as an oilseed and food material.Citation1) Recently, anthrasesamone F, which is a hydroxylated anthraquinone derivative possessing a potent radical scavenging activity, was identified as one of the characteristic components of black sesame seeds.Citation2,3)
On the other hand, investigation of the constituents of S. indicum roots and hairy roots established that the roots contain several anthraquinone derivatives, including 2-(4-methylpent-3-en-1-yl)anthraquinone (MPAQ) (1),Citation4,5) 2-[(Z)-4-methylpenta-1,3-dien-1-yl]anthraquinone ((Z)-MPDEAQ) (2),Citation6) anthrasesamones A (3), B (4) and CCitation5) and 2,3-epoxyanthrasesamone B (5)Citation7) (Fig. ). The carbon skeleton of MPAQ (1), which contains a branched C6 side chain at C-2 in the anthraquinone ring, is identical with that of other anthraquinone derivatives (2–5). The biogenetic conversion of MPAQ (1) to the anthraquinone derivatives that have the dehydrogenated diene side chain (2) or oxygenated anthraquinone rings (3–5) was deduced from their chemical structures, but biosynthetic investigations have not been made. In addition, we previously proposed that MPAQ (1) would be formed from 2-geranyl-1,4-naphthohydroquinone (7) on the basis of the biosynthetic pathway of shikonin by Lithospermum erythrorhizon, a naphthoquinone derivative having the same C6 side chain as MPAQ (1),Citation8) as well as the formation of anthraquinone derivatives from prenylated naphthoquinone (and/or naphthohydroquinone) derivatives by plants.Citation9,10) Later, 2-geranyl-1,4-naphthoquinone (6) corresponding to a quinone form of hydroquinone 7 has been isolated from sesame hairy roots.Citation11) Moreover, the incorporation of [1-13C]glucose into MPAQ (1) and 2-geranyl-1,4-naphthoquinone (6) demonstrated that these possible intermediates were biosynthesized through the same biogenetic route, the shikimate and methylerythritol phosphate (MEP) pathways.Citation12) However, the biogenetic relationships among quinone derivatives in sesame have not been definitively verified. We therefore undertook administration experiments with 2H-labeled MPAQ (1a) and 2-geranyl-1,4-naphthohydroquinone (7a) to elucidate the intermediacy of 1 and 7 in the biosynthesis of anthraquinone derivatives by S. indicum hairy roots.
Materials and methods
General experimental procedures
1H and 13C NMR spectra were measured with a JEOL JNM-ECA600 FT NMR spectrometer in CDCl3 at 600 MHz for 1H and at 150 MHz for 13C. NMR chemical shifts were referenced to the solvent (δH 7.24, δC 77.0). The deuterium content of individual hydrogen atoms was estimated by comparison of the proton signal integrals between the 2H-labeled and unlabeled positions in each compound. 2H NMR spectra were recorded with the same instrument in CHCl3 at 92 MHz. Mass spectra were obtained with a JEOL JMS-700 mass spectrometer. [2H8]Naphthalene (99 atom% 2H) was purchased from Sigma-Aldrich, USA. Silica gel 60 (70–230 mesh, Nacalai Tesque, Japan) and Sephadex LH-20 (GE Healthcare Bio-Sciences AB, Sweden) were used for column chromatography. All experimental procedures were carried out in the dark or under as low light condition as possible, because some quinone derivatives, in particular (Z)-MPDEAQ,Citation6) are unstable to light.
Synthesis of [2H6]-1,4-naphthoquinone
A solution of ammonium cerium(IV) sulfate dihydrate (7.59 g) in 2 M H2SO4 (100 mL) was added to [2H8]naphthalene (273 mg) in MeCN (80 mL) and 2 M H2SO4 (20 mL), and the solution was stirred overnight at room temperature.Citation13) The reaction mixture was poured into H2O (200 mL) and treated with EtOAc (50 mL × 4). The EtOAc solution obtained was washed with saturated NaCl (100 mL × 2), dried over Na2SO4 and concentrated to dryness under reduced pressure to give [2H6]-1,4-naphthoquinone (315 mg, 96% yield).
Synthesis of 2-(4-methylpent-3-en-1-yl)[5,6,7,8-2H4]anthraquinone (1a)
[5,6,7,8-2H4]MPAQ (1a) was prepared by reference to the method used for the synthesis of unlabeled MPAQ (1).Citation14) A solution of [2H6]-1,4-naphthoquinone (151 mg) and 7-methyl-3-methyleneocta-1,6-diene (β-myrcene, 277 mg, 72% purity) in dry toluene (10 mL) was allowed to stand for 5 days at 60 °C. A solution of 1 M KOH in MeOH (5 mL) was added to the reaction mixture at room temperature, and the solution was stirred vigorously for 90 min without sealing. The reaction mixture was poured into H2O (50 mL) and treated with EtOAc (25 mL × 3). The EtOAc solution obtained was washed with saturated NaCl (40 mL × 2) and concentrated to dryness under reduced pressure. The residue was chromatographed on silica gel column by elution with EtOAc–hexane (5:95). The fractions containing 1a were combined and further purified by recrystallization from EtOAc–hexane to afford 2H-labeled 1a (210 mg, 78% yield). 1H NMR (CDCl3) δH: 1.52 (3H, br.s, H-6′), 1.66 (3H, br.s, H-5′), 2.36 (2H, br.dt, J = 7.2, 7.7 Hz, H-2′), 2.79 (2H, t-like, J = 7.7 Hz, H-1′), 5.13 (1H, tqq, J = 7.2, 1.4, 1.4 Hz, H-3′), 7.58 (1H, dd, J = 7.9, 1.8 Hz, H-3), 8.10 (1H, d, J = 1.8 Hz, H-1), 8.20 (1H, d, J = 7.9 Hz, H-4). Two residual proton signals were observed at 2H-labeled positions (>99 atom% 2H, δH): 7.77 (H-6 and H-7), 8.29 (H-5 and H-8). 13C NMR (CDCl3) δC: 17.7 (C-6′), 25.7 (C-5′), 29.4 (C-2′), 36.3 (C-1′), 122.7 (C-3′), 127.0 (C-1), 127.4 (C-4), 131.5 (C-4a), 133.1 (C-4′), 133.5 (C-9a), 133.6 (C-8a), 133.6 (C-10a), 134.5 (C-3), 149.6 (C-2), 183.1 (C-10), 183.5 (C-9). HR-EIMS m/z (M+): Calcd for C201H142H4O2, 294.1558; found, 294.1567.
Synthesis of 2-geranyl[3,5,6,7,8-2H5]-1,4-naphthoquinone (6a)
2-Geranyl[3,5,6,7,8-2H5]-1,4-naphthoquinone (6a) was prepared using a modification of the method described by Tsuchimoto et al.Citation15) (E)-3,7-Dimethylocta-2,6-dien-1-ol (geraniol, 463 mg, 526 μL) and scandium(III) trifluoromethanesulfonate (25 mg) were added to [2H6]-1,4-naphthoquinone (164 mg), and the solution was sonicated for 1 min and allowed to stand for 24 h at 50 °C. The reaction mixture was diluted with CH2Cl2 (2 mL × 5) and filtered through Celite, before the filtrate was evaporated to dryness. The residue was chromatographed on silica gel column by elution with acetone–hexane (5:95). The fractions containing 6a were combined and further purified by preparative HPLC (column, Cosmosil Cholester, 250 × 10 mm i.d., Nacalai Tesque; detection, 254 nm) using MeOH as the mobile phase (flow rate, 1.5 mL/min) to afford 2H-labeled 6a (18 mg, 6% yield). 1H NMR (CDCl3) δH: 1.59 (3H, br.s, H-9′), 1.64 (3H, br.s, H-10′), 1.67 (3H, br.s, H-8′), 2.09 (4H, m, H-4′ and H-5′), 3.26 (2H, br.d, J = 7.3 Hz, H-1′), 5.07 (1H, tm, J = 6.7 Hz, H-6′), 5.21 (1H, tm, J = 7.3 Hz, H-2′). Four residual proton signals were observed at 2H-labeled positions (approximately 99 atom% 2H, δH): 6.74 (H-3), 7.70 (H-6 and H-7), 8.04 (H-5), 8.09 (H-8). 13C NMR (CDCl3) δC: 16.1 (C-10′), 17.7 (C-9′), 25.7 (C-8′), 26.5 (C-5′), 27.8 (C-1′), 39.7 (C-4′), 118.1 (C-2′), 123.9 (C-6′), 131.8 (C-7′), 132.1 (C-4a), 132.3 (C-8a), 140.0 (C-3′), 150.7 (C-2), 185.3 (C-4), 185.4 (C-1). HR-EIMS m/z (M+): Calcd for C201H172H5O2, 299.1934; found, 299.1932.
Plant material
Hairy roots of S. indicum were induced by directly infecting axenic sesame seedlings with Agrobacterium rhizogenes ATCC 15834.Citation16) The established hairy root clone (SI-16) was maintained in a phytohormone-free Gamborg B5 liquid mediumCitation17) containing 2% glucose (pH 6.1) in the dark at 25 °C on a rotary shaker at 70 rpm at intervals of 14 days.
Administration of 2H-labeled MPAQ (1a)
The hairy roots (about 0.3 g fresh wt.) were cultured in a 100-mL conical flask containing 50 mL of the B5 liquid medium supplemented with 2% sucrose in the dark at 25 °C at 70 rpm. Twenty flasks containing hairy roots that had been grown for 14 days were washed with sterile H2O (25 mL × 2 per flask), before the medium was replaced with fresh B5 medium supplemented with 0.2% sucrose and 100 mM NaCl. 2H-labeled 1a (10 mg, 0.5 mg/flask) dissolved in EtOH (1.2 mL) was then fed to these flasks containing the fresh medium and washed hairy roots. The hairy roots were cultured under the same condition for four days with 2H-labeled 1a. The hairy roots and secreted metabolites were separated from the medium by gravity filtration through filter paper. The harvested hairy roots and filter paper were freeze-dried and sonicated for 30 min in MeOH (150 mL × 3). The MeOH solution obtained was concentrated to dryness under reduced pressure. The MeOH extract was partitioned between CH2Cl2 (100 mL × 3) and H2O (100 mL) to give the CH2Cl2-soluble fraction. This fraction was subjected to silica gel column chromatography using stepwise elution with acetone–hexane containing 0.1% AcOH. The 5 and 10% acetone fractions were combined and purified by Sephadex LH-20 column chromatography eluted with MeOH–CH2Cl2 (1:1). The fractions containing the metabolites were further purified by reversed-phase HPLC (column, Cosmosil 5C18-AR-II, 250 × 10 mm i.d., Nacalai Tesque; detection, 254 nm) using MeOH–AcOH as the mobile phase (solvent, 100:0.2; flow rate, 1.5 mL/min) to afford 1 (5 mg), 2 (3 mg), 4 (0.5 mg), 5 (3 mg) and 6 (<0.5 mg).
Compound 2
1H NMR (CDCl3) δH: 1.86 and 1.87 (each 3H, br.s, H-5′ and H-6′), 6.37 (1H, dm, J = 11.7 Hz, H-3′), 6.38 (1H, d, J = 11.7 Hz, H-1′), 6.62 (1H, dd, J = 11.7, 11.7 Hz, H-2′), 7.73 (1H, dd, J = 8.0, 1.8 Hz, H-3), 7.78 (0.48H, m, H-6 and H-7), 8.24 (1H, d, J = 1.8 Hz, H-1), 8.25 (1H, d, J = 8.0 Hz, H-4), 8.30 (0.48H, m, H-5 and H-8). EIMS m/z (rel. int.): 288 (M+, 3.4), 292 (M++4, 12.4).
Administration of 2H-labeled 2-geranyl-1,4-naphthohydroquinone (7a)
The administration experiment with 2H-labeled 7a was carried out by the same method as that used for 2H-labeled 1a, except for the preparation of 7a from 6a just before the administration of the labeled substrate. A solution of 6a (15 mg) in Et2O (10 mL) was shaken with 15% sodium dithionite (10 mL). The Et2O layer obtained was washed with a mixture of saturated NaCl (10 mL) and 15% sodium dithionite (2 mL), passed through Na2SO4 and concentrated to dryness under reduced pressure. Without storage, the reduced product (7a) was dissolved in EtOH (2.4 mL) and immediately fed to the hairy root cultures. After the final purification by reversed-phase HPLC, 1 (1 mg), 2 (2 mg), 4 (0.5 mg) and 5 (1 mg) and 6 (4 mg) were isolated.
Compound 1
1H NMR (CDCl3) δH: 1.53 (3H, br.s, H-6′), 1.66 (3H, br.s, H-5′), 2.36 (2H, br.dt, J = 7.2, 7.7 Hz, H-2′), 2.80 (2H, t-like, J = 7.7 Hz, H-1′), 5.13 (1H, tqq, J = 7.2, 1.4, 1.4 Hz, H-3′), 7.59 (1H, dd, J = 7.9, 1.8 Hz, H-3), 7.77 (0.9H, m, H-6 and H-7), 8.11 (1H, d, J = 1.8 Hz, H-1), 8.21 (1H, d, J = 7.9 Hz, H-4), 8.29 (0.9H, m, H-5 and H-8). EIMS m/z (rel. int.): 290 (M+, 19.5), 294 (M++4, 22.2).
Compound 2
1H NMR (CDCl3) δH: 1.86 and 1.87 (each 3H, br.s, H-5′ and H-6′), 6.37 (1H, dm, J = 11.7 Hz, H-3′), 6.38 (1H, d, J = 11.6 Hz, H-1′), 6.62 (1H, dd, J = 11.7, 11.6 Hz, H-2′), 7.74 (1H, dd, J = 8.0, 1.8 Hz, H-3), 7.78 (0.86H, m, H-6 and H-7), 8.24 (1H, d, J = 1.8 Hz, H-1), 8.26 (1H, d, J = 8.0 Hz, H-4), 8.30 (0.86H, m, H-5 and H-8). EIMS m/z (rel. int.): 288 (M+, 30.5), 292 (M++4, 47.7).
Results and discussion
We examined whether MPAQ (1) and 2-geranyl-1,4-naphthohydroquinone (7) serve as intermediates for anthraquinone derivatives produced by S. indicum hairy roots. A preliminary administration experiment using a conventional B5 medium was unsuccessful because of very low or no accumulation of anthraquinone derivatives such as 3–5 (data not shown). In order to improve the production of anthraquinone derivatives in the hairy roots, several culture conditions were tested. The addition of sodium chloride at 100 mM in the B5 mediumCitation7) gave a sufficient amount of 2,3-epoxyanthrasesamone B (5) together with a small amount of anthrasesamone B (4), although anthrasesamone A (3) unfortunately did not accumulate. Administration experiments with 2H-labeled substrates were therefore carried out using this improved medium. In contrast, the conventional B5 medium was utilized as the growth medium for reducing the endogenous production of anthraquinone derivatives, prior to administration of the labeled substrates.
[5,6,7,8-2H4]MPAQ (1a) was synthesized by the Diels–Alder cycloaddition reaction of [2H6]-1,4-naphthoquinone and β-myrcene and subsequent aromatization of the adduct.Citation14) 2H-labeled MPAQ (1a) (>99 atom% 2H) was administered to a two-week-old hairy root culture of S. indicum. After four days of incubation with 2H-labeled MPAQ (1a), quinone derivatives were extracted and isolated as described in the above section. The 1H NMR spectrum of (Z)-MPDEAQ (2) obtained from this experiment showed that two aromatic proton signals at δH 7.78 (H-6 and H-7) and 8.30 (H-5 and H-8) in isolated 2 had remarkably decreased intensities (Fig. (A)) compared to natural abundance 2 (Fig. (C)) and that the content of deuterium atoms in isolated 2 was 76% at these positions, indicating that exogenously supplied 1a was efficiently converted to 2. Moreover, the existence of the deuterium labels in isolated 2 was confirmed by the 2H NMR analysis, where two broad singlet signals having almost the same signal integral were observed at the corresponding positions. These results clearly demonstrate that MPAQ (1) is an actual biosynthetic precursor for the formation of (Z)-MPDEAQ (2). As expected, the hairy roots did not convert labeled MPAQ (1a) to 2-geranyl-1,4-naphthoquinone (6). Contrary to our presumption, however, the conversion of labeled MPAQ (1a) to anthrasesamone B (4) and 2,3-epoxyanthrasesamone B (5), which are respectively 1,4-dihydroxy and 2,3-epoxy-1,4-dihydroxy analogs of 1, was not observed in the 1H and 2H NMR spectra of isolated 4 and 5. Dilution of fed MPAQ (1a) by endogenous unlabeled 1 was excluded because recovered 1 was still fully enriched with the deuterium atoms (94%). These findings suggested that anthrasesamone B (4) and 2,3-epoxyanthrasesamone B (5) may be formed without passing through MPAQ (1).
Fig. 2. Partial 1H NMR spectra of (Z)-MPDEAQ (2).
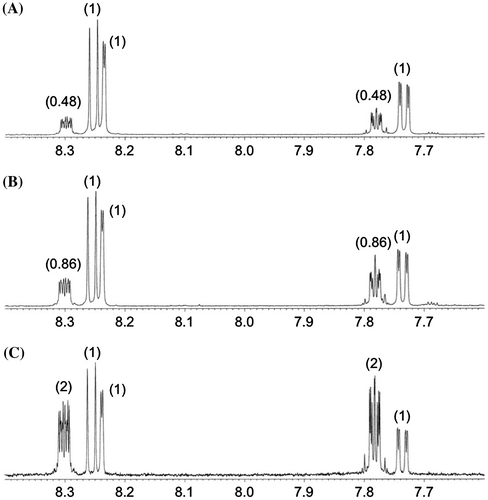
It is well known that shikonin is biosynthesized through the formation of a geranylated intermediate, geranylhydroquinone, and subsequent cyclization with the geranyl side chain to construct the naphthoquinone ring.Citation18,19) In addition, LeistnerCitation20) reported that 2-prenyl-1,4-naphthohydroquinone was incorporated into alizarin, an anthraquinone derivative in Rubia tinctorum roots, whereas 2-prenyl-1,4-naphthoquinone was not. Based on these previous findings, we selected 2-geranyl-1,4-naphthohydroquinone (7), but not 2-geranyl-1,4-naphthoquinone (6), as the most effective substrate for further administration experiment. 2H-labeled 7a was prepared by reduction of previously synthesized 6a just before its administration, because in general, naphthohydroquinones change to the corresponding naphthoquinones by air oxidation. 2-Geranyl[3,5,6,7,8-2H5]-1,4-naphthoquinone (6a) was synthesized by the scandium triflate-catalyzed Friedel–Crafts alkylation reaction of [2H6]-1,4-naphthoquinone with geraniolCitation15) and then was reduced to 2-geranyl[3,5,6,7,8-2H5]-1,4-naphthohydroquinone (7a) with sodium dithionite. 2H-labeled 7a (about 99 atom% 2H) was next administered to the hairy root culture, and quinone derivatives were isolated again as described above. Incidentally, the deuterium content of recovered 6 was 97% at the corresponding positions. In the 1H NMR spectrum of MPAQ (1) obtained from this experiment, two aromatic proton signals at δH 7.77 (H-6 and H-7) and 8.29 (H-5 and H-8) had reduced intensities (Fig. (A)) weaker than natural abundance 1 (Fig. (B)), and the deuterium content was evaluated to be 55%, evidence that 2-geranyl-1,4-naphthohydroquinone (7) is a substantial precursor for the formation of MPAQ (1). Labeled 7a was also converted to (Z)-MPDEAQ (2) (57%) (Fig. (B)). These data support the supposition that (Z)-MPDEAQ (2) is derived from MPAQ (1), which is generated from 2-geranyl-1,4-naphthohydroquinone (7). In contrast to MPAQ (1) and (Z)-MPDEAQ (2) formation, labeled 7a was not converted to anthrasesamone B (4) and 2,3-epoxyanthrasesamone B (5), in analogy with the result using 1a, suggesting that 2-geranyl-1,4-naphthohydroquinone (7), as well as 1, does not seem to participate in the biosynthesis of 4 and 5.
Fig. 3. Partial 1H NMR spectra of MPAQ (1).
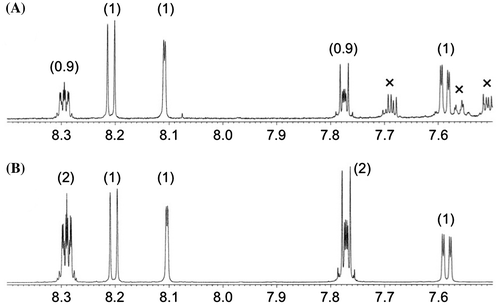
On the basis of the above findings, we propose the biosynthetic pathways of 2-geranyl-1,4-naphthohydroquinone (7), MPAQ (1) and (Z)-MPDEAQ (2) to be as shown in Fig. . These quinone derivatives in S. indicum hairy roots originate from 2-carboxy-1,4-naphthohydroquinone (1,4-dihydroxy-2-naphthoic acid) or 2-carboxy-2,3-dihydro-1,4-naphthoquinone (2-carboxy-4-oxotetralone) via the shikimate pathway and geranyl diphosphate via the MEP pathway.Citation12) Geranylation and decarboxylation at C-2 in the aromatic intermediate produce 2-geranyl-1,4-naphthohydroquinone (7). In shikonin biosynthesis, 4-hydroxybenzoic acid is geranylated with geranyl diphosphate by 4-hydroxybenzoate geranyltransferaseCitation21) to produce 3-geranyl-4-hydroxybenzoic acid, which is then converted to geranylhydroquinone. Moreover, geranylhydroquinone 3˝-hydroxylase, a cytochrome P-450 monooxygenase, has been identified as a key enzyme involved in the formation of the naphthoquinone ring in shikonin.Citation22) This enzyme specifically hydroxylates one of the three methyl groups present in the geranyl side chain of geranylhydroquinone before the cyclization reaction to generate the naphthoquinone ring. Accordingly, oxidation of the methyl group present at C-3′ in the geranyl side chain of 7 and subsequent cyclization between the naphthohydroquinone ring and the oxidized side chain to generate the anthraquinone ring would lead to the formation of MPAQ (1). Dehydrogenation of the C6 side chain in 1 would produce (Z)-MPDEAQ (2).
Fig. 4. Proposed biosynthetic pathway of MPAQ (1) and (Z)-MPDEAQ (2) from 2-geranyl-1,4-naphthohydroquinone (7) through the shikimate and MEP pathways in S. indicum hairy roots.
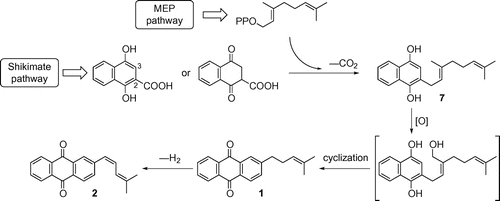
On the other hand, we also deduced the production of anthrasesamone B (4) and 2,3-epoxyanthrasesamone B (5) from 2-geranyl-1,4-naphthohydroquinone (7) and MPAQ (1), but our present study rules out this hypothesis. This suggests that 7 and 1 may not be the common precursors in the biosynthesis of anthraquinone derivatives by S. indicum and that the biogenetic route of 4 and 5 may be different from that of (Z)-MPDEAQ (2). Although several possibilities are assumed at the present state, one of the potential intermediates may be 2-carboxy-2-geranyl-2,3-dihydro-1,4-naphthoquinone, and it might be used as the substrate without conversion to 7 (Fig. S1).Citation23) Alternatively, 2-carboxy-3-geranyl-1,4-naphthohydroquinone may be the intermediate, which might be used without decarboxylation to 7 (Fig. S1).Citation10) Furthermore, cyclization of these newly presumed intermediates via certain metabolic steps might lead to the formation of hydroxylated anthraquinone derivatives such as anthrasesamones A (3) and B (4) and 2,3-epoxyanthrasesamone B (5) without the involvement of 1. The difference in the biosynthetic pathways among anthraquinone derivatives produced by S. indicum is an interesting topic and has to be further investigated.
Supplemental data
Supplemental data for this article can be accessed at https://doi.org/10.1080/09168451.2017.1362974.
Disclosure statement
No potential conflict of interest was reported by the authors.
Author contributions
T.F. conceived and designed the experiments, discussed the results, and wrote the manuscript. T.F. and R.S. performed the experiments and analyzed the data.
BBB-FigS1-R1.pdf
Download PDF (63.6 KB)Acknowledgments
We are grateful to Prof. Yukihiro Sugimoto of Kobe University for his technical support on mass spectrometric measurement.
References
- Morris JB. Food, industrial, nutraceutical, and pharmaceutical uses of sesame genetic resources. In: Janick J, Whipkey A, editors. Trends in new crops and new uses. Alexandria (VA): ASHS Press; 2002. p. 153–156.
- Kim KS, Park SH. Anthrasesamone F from the seeds of black Sesamum indicum. Biosci Biotechnol Biochem. 2008;72:1626–1627.10.1271/bbb.80051
- Furumoto T, Nishimoto K. Identification of a characteristic antioxidant, anthrasesamone F, in black sesame seeds and its accumulation at different seed developmental stages. Biosci Biotechnol Biochem. 2016;80:350–355.10.1080/09168451.2015.1091717
- Ogasawara T, Chiba K, Tada M. Production in high-yield of a naphthoquinone by a hairy root culture of Sesamum indicum. Phytochemistry. 1993;33:1095–1098.10.1016/0031-9422(93)85029-Q
- Furumoto T, Iwata M, Feroj Hasan AFM, et al. Anthrasesamones from roots of Sesamum indicum. Phytochemistry. 2003;64:863–866.10.1016/j.phytochem.2003.09.004
- Furumoto T, Jindai A. Isolation and photoisomerization of a new anthraquinone from hairy root cultures of Sesamum indicum. Biosci Biotechnol Biochem. 2008;72:2788–2790.10.1271/bbb.80373
- Furumoto T, Hoshikuma A. Effect of chloride ions on anthrasesamone C production in a Sesamum indicum hairy root culture and identification of the precursor for its abiotic formation. Biosci Biotechnol Biochem. 2012;76:305–308.10.1271/bbb.110688
- Tabata M. The mechanism of shikonin biosynthesis in Lithospermum cell cultures. Plant Tissue Cult Lett. 1996;13:117–125.10.5511/plantbiotechnology1984.13.117
- Herbert RB. The biosynthesis of secondary metabolites. 2nd ed. London: Chapman and Hall; 1989.10.1007/978-94-010-9132-9
- Seigler DS. Plant secondary metabolism. Boston (MA): Kluwer Academic Publishers; 1998.10.1007/978-1-4615-4913-0
- Furumoto T, Ohara T, Kubo T, et al. 2-Geranyl-1,4-naphthoquinone, a possible intermediate of anthraquinones in a Sesamum indicum hairy root culture. Biosci Biotechnol Biochem. 2007;71:2600–2602.10.1271/bbb.70352
- Furumoto T, Hoshikuma A. Biosynthetic origin of 2-geranyl-1,4-naphthoquinone and its related anthraquinone in a Sesamum indicum hairy root culture. Phytochemistry. 2011;72:871–874.10.1016/j.phytochem.2011.03.012
- Periasamy M, Bhatt MV. A convenient method for the oxidation of polycyclic aromatic hydrocarbons to quinones. Synthesis. 1977;1977: 330–332.
- Eckert RC, Amos LW. Influence of hydrophilicity on the delignification efficiency of anthraquinone derivatives. J Wood Chem Technol. 1982;2:57–71.10.1080/02773818208085120
- Tsuchimoto T, Tobita K, Hiyama T, et al. Scandium(III) triflate-catalyzed Friedel−Crafts alkylation reactions. J Org Chem. 1997;62:6997–7005.10.1021/jo970599u
- Shimomura K, Sudo H, Saga H, et al. Shikonin production and secretion by hairy root cultures of Lithospermum erythrorhizon. Plant Cell Rep. 1991;10:282–285.
- Gamborg OL, Miller RA, Ojima K. Nutrient requirements of suspension cultures of soybean root cells. Exp Cell Res. 1968;50:151–158.10.1016/0014-4827(68)90403-5
- Inouye H, Ueda S, Inoue K, et al. Biosynthesis of shikonin in callus cultures of Lithospermum erythrorhizon. Phytochemistry. 1979;18:1301–1308.10.1016/0031-9422(79)83012-5
- Yazaki K, Fukui H, Tabata M. Isolation of the intermediates and related metabolites of shikonin biosynthesis from Lithospermum erythrorhizon cell cultures. Chem Pharm Bull. 1986;34:2290–2293.10.1248/cpb.34.2290
- Leistner E. Mode of incorporation of precursors into alizarin (1,2-dihydroxy-9,10-anthraquinone). Phytochemistry. 1973;12:337–345.10.1016/0031-9422(73)80015-9
- Boehm R, Li SM, Melzer M, et al. 4-Hydroxybenzoate prenyltransferases in cell-free extracts of Lithospermum erythrorhizon cell cultures. Phytochemistry. 1997;44:419–424.10.1016/S0031-9422(96)00458-X
- Yamamoto H, Inoue K, Li SM, et al. Geranylhydroquinone 3″-hydroxylase, a cytochrome P-450 monooxygenase from Lithospermum erythrorhizon cell suspension cultures. Planta. 2000;210:312–317.10.1007/PL00008139
- Inouye H, Ueda S, Inoue K, et al. (2R)-Catalponone, a biosynthetic intermediate for prenylnaphthoquinone congeners of the wood of Catalpa ovata. Phytochemistry. 1981;20:1707–1710.