Abstract
Clostridium thermocellum is a candidate bacterium for lignocellulose utilization due to its efficient lignocellulose solubilization ability. It has been reported that C. thermocellum efficiently degrades purified cellulose substrates, but cannot completely degrade milled lignocellulose powders. Evaluation of cellulose and hemicellulose contents in a lignocellulose residue after the cultivation of C. thermocellum indicated that C. thermocellum degraded cellulose and hemicellulose equally. Microscopic observations demonstrated that C. thermocellum significantly degraded small-sized lignocellulose particles, but it only partially degraded the larger sized particles. The lignin content of the large-sized particles was higher than that of the small particles. The remained large-sized particles included vascular tissues. These results suggest that the lignified structures such as vascular tissues in milled lignocellulose were less susceptible to bacterial lignocellulose solubilization.
Large-sized lignified structures in milled lignocellulose were less susceptible to bacterial lignocellulose solubilization.
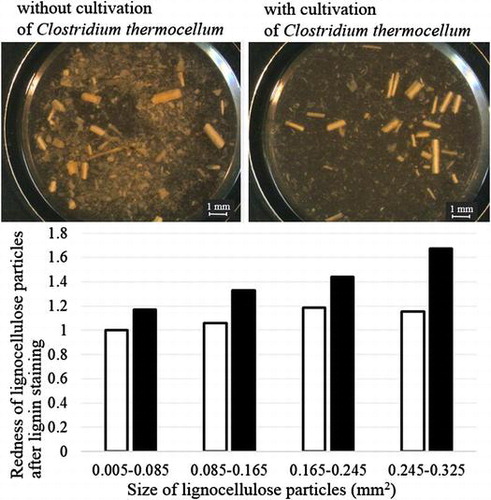
Chemical products and energies that support our lives are mainly produced from fossil resources. However, the use of fossil resources emits carbon dioxide, which is the main cause of global warming, and there are concerns about the depletion of these natural resources.Citation1) Therefore, the introduction of new resources to replace fossil fuels is required. Lignocellulose such as switchgrass, corn stover, and rice straw is a currently unutilized resource that is available in a renewable form.Citation2) Since lignocellulose is produced by absorbing carbon dioxide through photosynthesis, its use would not increase the amount of carbon dioxide in the atmosphere.
Synthetic biology approaches have utilized micro-organisms such as Escherichia coli or Saccharomyces cerevisiae to produce chemicals (e.g. fuel compounds) by bioprocessing.Citation3,4) During the bioprocessing of lignocellulose, lignocellulose-degrading enzymes are used to convert lignocellulose into fermentable sugars; however, the efficiency of the enzymatic degradation is low.Citation5)
Clostridium thermocellum is a candidate bacterium for lignocellulose utilization such as biofuel production because of its ability to solubilize lignocellulose while producing ethanol.Citation6) C. thermocellum has been shown to produce 1.3% ethanol from 10% Avicel cellulose.Citation7) A multiple-deletion strain of C. thermocellum removing [FeFe] hydrogenase maturase, lactate dehydrogenase, pyruvate-formate lyase, Pfl-activating enzyme, phosphotransacetylase, and acetate kinase genes that eliminates formate, acetate, and lactate production, as well as reduce H2 production, presented a titer of 2.2% ethanol from 6% Avicel cellulose.Citation8) A co-culture of a lactate dehydrogenase/phosphotransacetylase gene deletion strain and Thermoanaerobacterium saccharolyticum produced 3.8% ethanol from 9.2% Avicel cellulose in 146 h.Citation9) The cultivations of C. thermocellum can be a simplified consolidated bioprocessing. This is a promising method because it eliminates the need to add lignocellulose-degrading enzymes that significantly increase the cost of biofuel production.Citation10–12)
Multicellulolytic enzyme complexes, called cellulosomes, largely contribute to the lignocellulose solubilization ability of C. thermocellum.Citation13–15) Because cellulosomes show higher cellulolytic activity, they have been characterized in many papers.Citation16,17) As we mentioned above, if C. thermocellum is applied to a consolidated bioprocessing strategy, it should be characterized by how the bacterium degrades lignocellulose. Cellulose hydrolysis rates were higher for growing cultures of C. thermocellum compared to purified cellulase preparations from this organism.Citation18) It is known that when C. thermocellum is cultured using purified celluloses, they can be completely degraded.Citation7) C. thermocellum regulates the production of lignocellulose-degrading enzymes during cellulose solubilization.Citation19) It also regulates the production of enzymes for the efficient solubilization of various polysaccharide substrates.Citation20) Cellulosome samples from pretreated switchgrass-grown cultures displayed distinct differences in composition compared to cellulosome samples from crystalline cellulose-grown cultures.Citation21) Recent studies reported that alternative sigma factors regulate the production of lignocellulose-degrading enzymes.Citation22–26) Sand et al. showed that the deletion of a sigma factor, sigI6, decreased the expression level of the xylanase genes xyn11, xyn10Z, and xyn10D in C. thermocellum.Citation25)
Despite the regulations of lignocellulose-degrading enzyme production, C. thermocellum cannot degrade milled lignocellulose powders completely, e.g. it degraded 32.5%Citation27) or 65%Citation28) of milled switchgrass for five days. Milled lignocellulose powders include wide range of particle size distribution. These largely consist of cellulose, hemicellulose, and lignin. In this study, we evaluated the contents, sizes, and surface structures of lignocellulose particles during lignocellulose solubilization by C. thermocellum, and report that the lignified structures such as vascular tissues in milled lignocellulose were less susceptible to a bacterial lignocellulose solubilization.
Materials and methods
Strains and growth conditions
Corn hull was milled by knife milling, and sieved with a 0.5-mm sieve. Clostridium thermocellum DSM 1313 was inoculated in BM7CO medium (1.5 g/L KH2PO4, 2.9 g/L K2HPO4, 2.1 g/L urea, 6.0 g/L yeast extract, 0.5 g/L L-cysteine-HCl⋅H2O, 4.0 g/L Na2CO3, 0.5 mg/L MgCl2⋅6H2O, 7.5 μg/L CaCl2⋅2H2O, 250 μg/L resazurin, pH 7.0) containing 1% milled corn hull, and cultured at 60 °C under anaerobic conditions.Citation29)
Determination of cellulose, hemicellulose, and lignin content in lignocellulose
After cultivation of C. thermocellum, the culture broths were transferred into 50-mL tubes, centrifuged at 3500 rpm for 5 min, and then the supernatant was removed. The precipitates were frozen at −30 °C and freeze-dried for two days.
The amounts of cellulose, hemicellulose, and lignin in the precipitates were determined by measuring the neutral detergent fiber (NDF), the acid detergent fiber (ADF), and the acid detergent lignin (ADL) contents.Citation30) To measure the NDF contents, freeze-dried samples were transferred into a 100-mL neutral detergent solution (30 g/L sodium dodecyl sulfate, 18.6 g/L EDTA⋅2Na, 6.82 g/L B4O7Na2⋅10H2O, 11.48 g/L Na2HPO4, 10 mL triethylene glycol) with 250 units of heat-stable α-amylase (Sigma–Aldrich), and boiled for 1 h. Insoluble fractions were collected with glass filters by suction filtration. While filtering under suction, the insoluble fractions were washed with hot water and with 100 mL acetone, and then allowed to dry for 2 h at 135 °C. After cooling in a desiccator, the samples were weighed and then heated at 200 °C for 30 min and then at 500 °C for 2 h to incinerate them. Samples were cooled again in a desiccator and the incinerated samples were then weighed. The NDF contents (i.e. total amount of cellulose, hemicellulose, and lignin in lignocellulose) were calculated by subtracting the weights after incineration from the weights before incineration.
To measure the ADF and ADL contents, the freeze-dried lignocelluloses were transferred into a 100-mL acid detergent solution (26.3 mL concentrated sulfuric acid, 20 g/L hexadecyltrimethylammonium bromide), then boiled, washed in hot water, treated with acetone, and dried in the same manner as used for the NDF measurements. After cooling in a desiccator, the samples were weighed. Subsequently, 20 mL of 72% sulfuric acid was added to the samples on the glass filter, mixed gently, and incubated for 30 min. After repeating this operation for 3 h, suction filtration was carried out to remove sulfuric acid from the glass filter, and washed with hot water. The samples were dried at 135 °C for 2 h. After cooling in a desiccator, the samples were weighed. The samples were heated at 200 °C for 30 min and then at 500 °C for 2 h to incinerate them. These were cooled in a desiccator and then weighed again. The ADF contents (i.e. total amount of cellulose and lignin in lignocellulose) were calculated by subtracting the weight after incineration from the weight before the 72% sulfuric acid treatment. The ADL contents (i.e. total amount of lignin in lignocellulose) were calculated by subtracting the weights after incineration from the weights after the 72% sulfuric acid treatment.
The value of ADL was subtracted from the value of ADF to calculate the amount of cellulose. The value of ADF was subtracted from the value of NDF to calculate the amount of hemicellulose. Citation30)
Measurement of size and specific surface area of lignocellulose particles
C. thermocellum was inoculated in BM7CO medium containing 1% milled corn hull, and cultured at 60 °C under anaerobic conditions for 10 days. The culture broth was sampled and the residue particles were observed with a stereo microscope (SZX9, Olympus, Japan) or a scanning electron microscope (TM-1000 Miniscope, Hitachi High-Technologies, Japan). Images of residue particles were acquired. The area and the circumferential length of residual particles were measured with image analysis software, ImageJ (NIH, USA). In this study, area was defined as size of the residue particles. Specific surface area (mm−1) of residue particles was determined by dividing the value of circumferential length (mm) by the value of area (mm2).
Evaluation of lignin content in lignocellulose particles
Wiesner stain solution, which stains lignin red, was prepared by dissolving 0.3 g phloroglucinol in 10 mL ethanol and then mixing with 5 mL concentrated HCl.Citation31,32) Milled corn hull was mixed gently by inversion with the Wiesner staining solution. The stained lignocellulose residues were observed under a stereo microscope. The acquired image was split to extract the red color image and the area and brightness of the detected particles were measured with the image analysis software ImageJ.
Results
Cellulose, hemicellulose, and lignin content in lignocellulose after cultivation of C. thermocellum
C. thermocellum was cultivated with 1% milled corn hull. The cellulose, hemicellulose, and lignin contents in the residues were evaluated as 0.34, 0.55, and 0.02 g per gram of corn hull, respectively (Fig. ). The amount of cellulose decreased during the cultivation of C. thermocellum: 0.09 g at one week and 0.07 g at two weeks. The amount of hemicellulose also decreased: 0.16 g at 1 week and 0.11 g at two weeks. Thus, 70% and 80% of both contents degraded equally for one and two weeks, respectively. The amount of lignin did not decrease during the cultivation of C. thermocellum (Fig. ).
Microscopic observations of lignocellulose residues after cultivation of C. thermocellum
Observations with a stereo microscope demonstrated that shape and size of corn hull particles were diverse (Fig. (a) and (b)). Small particles, which were less than 1 mm in length, were significantly degraded after cultivation of C. thermocellum for 10 days, while large fiber-like particles, which were approximately 1 mm in length, tended to remain (Fig. (c)). Particles measuring 50 μm or less were not observed significantly after the cultivation of C. thermocellum (Fig. (d)).
Fig. 2. Microscopic observations of lignocellulose particles with or without cultivation of C. thermocellum.
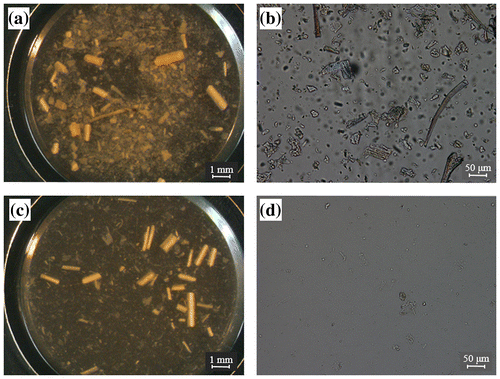
The degradation and size of the residue particles were quantified with the microscopic images. Number of lignocellulose particles having an area of 0.005–0.085, 0.085–0.165, 0.165–0.245, and 0.245–0.325 mm² were 71, 55, 54, and 17% decreased with cultivation of C. thermocellum, as compared to that without the cultivation (Fig. (a)). This result demonstrated that particle size is important for lignocellulose solubilization by C. thermocellum. This was also confirmed by observing which fractions of milled corn hull, sieved with a 0.1-mm mesh, were significantly degraded compared with the residual fraction (Fig. (b)).
Fig. 3. Effects of particle size on lignocellulose solubilization by C. thermocellum.
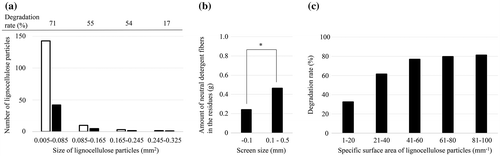
Specific surface area depends on the size and shape of particles. In this study, specific surface area (mm−1) of the particles was determined by dividing the circumferential length (mm) by the area (mm2). Particles having a specific surface area of 1–20, 21–40, 41–60, 61–80, and 81–100 mm−Citation1 were degraded by 32, 62, 77, 80, and 81%, respectively (Fig. (c)), suggesting that the larger the specific surface area of the lignocellulose particles, the higher the degradation rate.
Surface structure of residue particles were observed with a scanning electron microscope. While diverse structures were observed without the cultivation of C. thermocellum as is the case in the observation with a stereo microscope, only fiber-like particles were observed after the cultivation of C. thermocellum (Fig. ). Disruptions and cracks in epidermal tissues were often observed. Conductive structures were often observed in the residue particles (Fig. ).
Fig. 4. Surface structure of lignocellulose particles after cultivation of C. thermocellum.
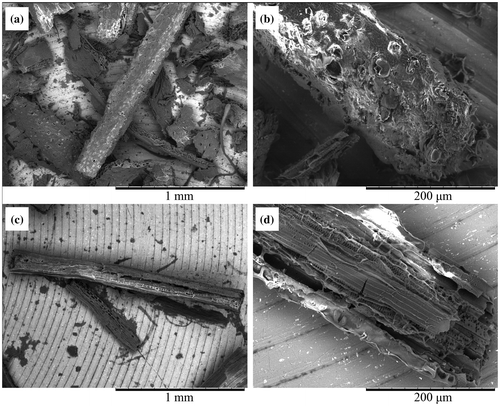
Evaluation of lignin content in lignocellulose particles
Phloroglucinol in Wiesner staining is the most commonly used stain for lignin determination and can provide clues to the amount of the cinnamaldehydes present in lignocellulose.Citation31) The redness of dyed milled corn hull particles was not homologous; only some particles were an obvious red after staining with phloroglucinol (Fig. (a) and (b)). By analyzing the acquired images, the redness of the particles was successfully quantified, and the relationship between particle size and the redness of the particle after lignin staining was evaluated. The redness in every lignocellulose size fraction significantly increased after Wiesner staining with phloroglucinol (p < 0.05 in Student’s t-test). While the redness of the 0.005–0.085 mm2 fraction increased 1.2 times, the redness of the 0.245–0.325 mm2 fraction increased 1.5 times after the staining with phloroglucinol (Fig. (c)), indicating that larger particles, which tend to remain after cultivation of C. thermocellum, include a higher lignin content than smaller particles.
Fig. 5. Relationship between lignocellulose particle sizes and lignin content.
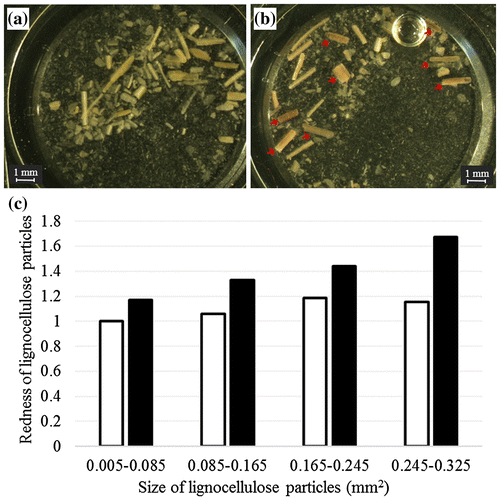
Discussion
In the case of the solubilization of 1% purified cellulose such as the Avicel cellulose, C. thermocellum completely degraded it in less than three days.Citation7) However, C. thermocellum was not able to completely degrade milled lignocellulose powders.Citation27,28) Since C. thermocellum was isolated as a cellulolytic bacterium,Citation33) we first speculated that it would degrade preferentially cellulose in milled lignocellulose powder and that the largest portion of the hemicellulose fraction would remain in the residues after the cultivation. However, the evaluation of the amount of cellulose, hemicellulose, and lignin contents in the residues during the solubilization by C. thermocellum demonstrated that the degradation rates of cellulose and hemicellulose were quite identical during cultivation (Fig. ). It is expected that hemicellulose and cellulose bind to each other, forming complex structures in lignocellulose.Citation34) Cellulosomes, C. thermocellum-produced enzyme complexes having a powerful cellulose-degrading ability,Citation16) have been reported as able to attach to the cell wall surface in a lignocellulose structure, but cannot penetrate it.Citation38,39) C. thermocellum did not specifically degrade cellulose in milled corn hull powders.
It was also reported that C. thermocellum strains, which show higher xylanase activity, degraded milled wheat straw more efficiently than type strains.Citation35) Enzymatic hydrolysis using xylanase and pectinase prior to C. thermocellum fermentation promoted the solubilization of ammonia fiber expansion-pretreated corn stover.Citation36) Verbeke et al. reported that xylose-induced biogenesis of a cyclical AgrD-type pentapeptide inhibits the growth of C. thermocellum.Citation37) These reports suggested that the hemicellulose content in lignocellulose inhibits the solubilization by C. thermocellum.
Since degradation rates of cellulose and hemicellulose were quite identical during the cultivation, next we hypothesized that size and specific surface area of lignocellulose particles are affect the bacterial solubilization. Microscopic observations demonstrated that small particles, less than 1 mm in length, were degraded after the cultivation of C. thermocellum, while large fiber-like particles, of 1 mm in length, tended to remain (Fig. (c)). The quantification analysis of particle size showed that particles having an area of 0.245–0.325 mm² were degraded by only 17% (Fig. (a)). Additionally, the passing fraction of milled corn hull sieved with a 0.1-mm mesh was degraded significantly compared to the residual fraction remaining after cultivation of C. thermocellum (Fig. (b)). C. thermocellum efficiently degraded lignocellulose particles with larger specific surface areas (Fig. (c)). These results indicate that particle size relates to the solubilization. Zhu et al. reported that specific surface of milled spruce related to the efficiency of enzymatic saccharification by Celluclast 1.5L and Novozyme 188.Citation40) Rajesh et al. also reported that 50% more glucose was produced from the small size particles (33–75 μm) of 10% red-oak sawdust slurries than the large size ones (590–850 μm) by the Multifect GC Cellulase enzyme. Citation41) The results of bacterial lignocellulose solubilization in this study showed good agreement with their results. For the particles of 61–80 and 81–100 mm−1, degradation rates ceased, suggesting the influence of other inhibiting components when the specific surface area is larger than 61 mm−1 (Fig. (c)).
Surface structure of the remained particles was disrupted after the cultivation of C. thermocellum. Conductive structures were obviously observed, indicating that the remained particles were vascular tissues in the corn hull (Fig. (c) and (d)). Lignin is difficult to biodegrade due to its complex chemical structure, it is especially accumulated in conductive structures in leaf organ. Delignification of lignocellulose significantly facilitated the binding and the solubilization of cellulosomes.Citation38) The amount of lignin was not decreased during the solubilization by C. thermocellum (Fig. ). It is speculated that the amount of lignin was higher in the large particles that were more difficult to degrade (Fig. (a)). In this study, lignin content in lignocellulose particles was successfully evaluated. The increase in the redness of the 0.245–0.325 mm2 fraction was higher than that of the 0.005–0.085 mm2 fraction, suggesting that the lignin content of larger lignocellulose particles was higher than that of smaller particles (Fig. (c)).
For efficient lignocellulose utilization with consolidated bioprocessing strategy, bacterial lignocellulose solubilization should be characterized in detail. As we have shown, C. thermocellum was not able to completely degrade milled lignocellulose powders. C. thermocellum degraded cellulose and hemicellulose of milled corn hull equally. It only partially degraded larger sized particles which included conductive structures. Lignin content of the large-sized particles was higher than small ones. These results suggest that the lignified structures such as vascular tissues in milled lignocellulose were less susceptible to a bacterial lignocellulose solubilization.
Author contributions
S. Ichikawa and S. Karita designed the experiments. S. Ichikawa, A. Nishida and S. Yasui performed the experiments. S. Ichikawa, A. Nishida and S. Yasui analyzed and interpreted the data. S. Ichikawa drafted the manuscript. All authors reviewed and approved the final manuscript.
Acknowledgments
We thank Dr. Yosuke Matsuda, Dr. Takashi Mishima, and Dr. Makoto Kondo, Mie University, for their instructions in microscopic observation and measurement of fiber contents of lignocellulose.
Disclosure statement
No potential conflict of interest was reported by the authors.
References
- Tett SFB, Stott PA, Allen MR, et al. Causes of twentieth-century temperature change near the Earth’s surface. Nature. 1999;399:569–572.10.1038/21164
- Tilman D, Hill J, Lehman C. Carbon-negative biofuels from low-input high-diversity grassland biomass. Science. 2006;314:1598–1600.10.1126/science.1133306
- Stephanopoulos G. Challenges in engineering microbes for biofuels production. Science. 2007;315:801–804.10.1126/science.1139612
- Peralta-Yahya PP, Zhang F, del Cardayre SB, et al. Microbial engineering for the production of advanced biofuels. Nature. 2012;488:320–328.10.1038/nature11478
- Himmel ME, Ding SY, Johnson DK, et al. Biomass recalcitrance: engineering plants and enzymes for biofuels production. Science. 2007;315:804–807.10.1126/science.1137016
- Blumer-Schuette SE, Brown SD, Sander KB, et al. Thermophilic lignocellulose deconstruction. FEMS Microbiol Rev. 2014;38:393–448.10.1111/1574-6976.12044
- Holwerda EK, Thorne PG, Olson DG, et al. The exometabolome of Clostridium thermocellum reveals overflow metabolism at high cellulose loading. Biotechnol Biofuels. 2014;7:155. Available from: https://biotechnologyforbiofuels.biomedcentral.com/articles/10.1186/s13068-014-0155-110.1186/s13068-014-0155-1
- Tian L, Papanek B, Olson DG, et al. Simultaneous achievement of high ethanol yield and titer in Clostridium thermocellum. Biotechnol Biofuels. 2016;9:116. Available from: https://biotechnologyforbiofuels.biomedcentral.com/articles/10.1186/s13068-016-0528-810.1186/s13068-016-0528-8
- Argyros DA, Tripathi SA, Barrett TF, et al. High ethanol titers from cellulose by using metabolically engineered thermophilic, anaerobic microbes. Appl Environ Microbiol. 2011;77:8288–8294.10.1128/AEM.00646-11
- Olson DG, McBride JE, Shaw AJ, et al. Recent progress in consolidated bioprocessing. Curr Opin Biotechnol. 2012;23:396–405.10.1016/j.copbio.2011.11.026
- Mazzoli R, Lamberti C, Pessione E. Engineering new metabolic capabilities in bacteria: lessons from recombinant cellulolytic strategies. Trends Biotechnol. 2012;30:111–119.10.1016/j.tibtech.2011.08.003
- Lynd LR, van Zyl WH, McBride JE, et al. Consolidated bioprocessing of cellulosic biomass: an update. Curr Opin Biotechnol. 2005;16:577–583.10.1016/j.copbio.2005.08.009
- Zverlov VV, Klupp M, Krauss J, et al. Mutations in the scaffoldin gene, cipA, of Clostridium thermocellum with impaired cellulosome formation and cellulose hydrolysis: insertions of a new transposable element, IS1447, and implications for cellulase synergism on crystalline cellulose. J Bacteriol. 2008;190:4321–4327.10.1128/JB.00097-08
- Olson DG, Giannone RJ, Hettich RL, et al. Role of the CipA scaffoldin protein in cellulose solubilization, as determined by targeted gene deletion and complementation in Clostridium thermocellum. J Bacteriol. 2013;195:733–739.10.1128/JB.02014-12
- Xu Q, Resch MG, Podkaminer K, et al. Dramatic performance of Clostridium thermocellum explained by its wide range of cellulase modalities. Sci Adv. 2016;2:e1501254.
- Doi RH, Kosugi A. Cellulosomes: plant-cell-wall-degrading enzyme complexes. Nat Rev Microbiol. 2004;2:541–551.
- Bayer EA, Lamed R, White BA, et al. From cellulosomes to cellulosomics. Chem Rec. 2008;8:364–377.10.1002/tcr.v8:6
- Lu Y, Zhang YH, Lynd LR. Enzyme-microbe synergy during cellulose hydrolysis by Clostridium thermocellum. Proc Nat Acad Sci USA. 2006;103(44):16165–16169.10.1073/pnas.0605381103
- Riederer A, Takasuka TE, Makino S, et al. Global gene expression patterns in Clostridium thermocellum as determined by microarray analysis of chemostat cultures on cellulose or cellobiose. Appl Environ Microbiol. 2011;77:1243–1253.10.1128/AEM.02008-10
- Zverlov VV, Schwarz WH. Bacterial cellulose hydrolysis in anaerobic environmental subsystems–Clostridium thermocellum and Clostridium stercorarium, thermophilic plant-fiber degraders. Ann N. Y. Acad Sci. 2008;1125:298–307.10.1196/annals.1419.008
- Raman B, Pan C, Hurst GB, et al. Impact of pretreated Switchgrass and biomass carbohydrates on Clostridium thermocellum ATCC 27405 cellulosome composition: a quantitative proteomic analysis. PLoS ONE. 2009;4:e5271.10.1371/journal.pone.0005271
- Nataf Y, Bahari L, Kahel-Raifer H, et al. Clostridium thermocellum cellulosomal genes are regulated by extracytoplasmic polysaccharides via alternative sigma factors. Proc Nat Acad Sci USA. 2010;107:18646–18651.10.1073/pnas.1012175107
- Kahel-Raifer H, Jindou S, Bahari L, et al. The unique set of putative membrane-associated anti-σ factors in Clostridium thermocellum suggests a novel extracellular carbohydrate-sensing mechanism involved in gene regulation. FEMS Microbiol Lett. 2010;308:84–93.10.1111/fml.2010.308.issue-1
- Bahari L, Gilad Y, Borovok I, et al. Glycoside hydrolases as components of putative carbohydrate biosensor proteins in Clostridium thermocellum. J Ind Microbiol Biotechnol. 2011;38:825–832.10.1007/s10295-010-0848-9
- Sand A, Holwerda EK, Ruppertsberger NM, et al. Three cellulosomal xylanase genes in Clostridium thermocellum are regulated by both vegetative SigA (σ(A)) and alternative SigI6 (σ(I6)) factors. FEBS Lett. 2015;589:3133–3140.10.1016/j.febslet.2015.08.026
- Muñoz-Gutiérrez I, Ortiz de Ora L, Rozman Grinberg I, et al. Decoding biomass-sensing regulons of Clostridium thermocellum alternative sigma-I factors in a heterologous Bacillus subtilis host system. PLOS ONE. 2016;11:e0146316.10.1371/journal.pone.0146316
- Izquierdo JA, Pattathil S, Guseva A, et al. Comparative analysis of the ability of Clostridium clariflavum strains and Clostridium thermocellum to utilize hemicellulose and unpretreated plant material. Biotechnol Biofuels. 2014;7:136. Available from: https://biotechnologyforbiofuels.biomedcentral.com/articles/10.1186/s13068-014-0136-410.1186/s13068-014-0136-4
- Paye JM, Guseva A, Hammer SK, et al. Biological lignocellulose solubilization: comparative evaluation of biocatalysts and enhancement via cotreatment. Biotechnol Biofuels. 2016;9:8. Available from: https://biotechnologyforbiofuels.biomedcentral.com/articles/10.1186/s13068-015-0412-y10.1186/s13068-015-0412-y
- Mori Y. Characterization of a symbiotic coculture of Clostridium thermohydrosulfuricum YM3 and Clostridium thermocellum YM4. Appl Environ Microbiol. 1990;56:37–42.
- Van Soest PJ, Robertson JB, Lewis BA. Methods for dietary fiber, neutral detergent fiber, and nonstarch polysaccharides in relation to animal nutrition. J Dairy Sci. 1991;74:3583–3597.10.3168/jds.S0022-0302(91)78551-2
- Adler E. Lignin chemistry-past, present and future. Wood Sci Technol. 1977;11:169–218.10.1007/BF00365615
- Liljegren S. 2010. Phloroglucinol stain for lignin. Cold Spring Harbor Protocols. pdb.prot4954.
- McBEE RH. The characteristics of Clostridium thermocellum. J Bacteriol. 1954;67:505–506.
- Burton RA, Gidley MJ, Fincher GB. Heterogeneity in the chemistry, structure and function of plant cell walls. Nat Chem Biol. 2010;6:724–732.10.1038/nchembio.439
- Resch MG, Donohoe BS, Baker JO, et al. Fungal cellulases and complexed cellulosomal enzymes exhibit synergistic mechanisms in cellulose deconstruction. Energy Environ Sci. 2013;6:1858–1867.10.1039/c3ee00019b
- Ding SY, Liu YS, Zeng Y, et al. How does plant cell wall nanoscale architecture correlate with enzymatic digestibility? Science. 2012;338:1055–1060.10.1126/science.1227491
- Shao X, Jin M, Guseva A, et al. Conversion for Avicel and AFEX pretreated corn stover by Clostridium thermocellum and simultaneous saccharification and fermentation: insights into microbial conversion of pretreated cellulosic biomass. Bioresour Technol. 2011;102:8040–8045.10.1016/j.biortech.2011.05.021
- Koeck DE, Koellmeier T, Zverlov VV, et al. Differences in biomass degradation between newly isolated environmental strains of Clostridium thermocellum and heterogeneity in the size of the cellulosomal scaffoldin. Syst Appl Microbiol. 2015;38:424–432.10.1016/j.syapm.2015.06.005
- Verbeke TJ, Giannone RJ, Klingeman DM, et al. Pentose sugars inhibit metabolism and increase expression of an AgrD-type cyclic pentapeptide in Clostridium thermocellum. Sci Rep. 2017;7:43355.10.1038/srep43355
- Zhu JY, Wang GS, Pan XJ, et al. Specific surface to evaluate the efficiencies of milling and pretreatment of wood for enzymatic saccharification. Chem Eng Sci. 2009;64:474–485.10.1016/j.ces.2008.09.026
- Dasari RK, Berson RE. The effect of particle size on hydrolysis reaction rates and rheological properties in cellulosic slurries. Appl Biochem Biotechnol. 2007;289:136–140.