Abstract
A novel anti-mollusk conopeptide pn4c was isolated from the Conus pennaceus venom by repeated HPLC fractionation based on the activity against freshwater snails. The primary structure of pn4c was determined by the mass spectrometric de novo sequencing analysis. In addition, pn3a was isolated from the same fraction containing pn4c, as a peptide with unknown functions.
Predatory marine cone snails (~700 snail species) produce a highly complex mixture of small (usually 10–35 amino acids) and disulfide-rich (2–5 bonds) peptides commonly known as conotoxins or conopeptidesCitation1). Since the venom of each cone snail species contains over 100 unique peptides, a repertoire of over 70,000 peptides was estimated to be produced by the Conus species. Most of conotoxins modulate the function of neurotransmitter receptors, ion channels and transporters with high specificity. Therefore, conotoxins are particularly useful for drug discovery and valuable as probes for neurophysiological studiesCitation2,3). Cone snails are categorized into carnivorous gastropods that are divided into three groups according to their prey; (1) vermivorous (worm-hunting) species, (2) molluscivorous (snail-hunting) species, and (3) piscivorous (fish-hunting) species. It is reasonable to assume that Conus species belonging to each group have conotoxins that specifically act on feeding animals. For example, TxVIA was isolated as a mollusk-specific toxin from the molluscivorous snail Conus textile venomCitation4). The studies of these diet-specific conotoxins should provide useful information for understanding the evolutionary prey shifts of cone snails from worm-hunting to mollusk- or fish-huntingCitation5). In the present study, we report the identification of a novel conopeptides pn4c and pn3a from the venom of the snail-hunting cone snail Conus pennaceus collected in the Red Sea Coast of Egypt. The anti-mollusk conopeptide pn4c was isolated by repeated HPLC fractionations based on the toxicity against freshwater snails. In addition, pn3a was isolated from the same fraction containing pn4c, as a peptide with unknown functions. We determined their primary structures using tandem mass spectrometry (MS), and evaluated their biological activity using the chemically synthesized peptides.
Venom components extracted from the venom duct of C. pennaceus were separated by a C4 RP-HPLC column (Fig. (a)). Each fraction that was manually collected every 5 min was tested for anti-mollusk toxicity by intramuscular injection into the foot of the freshwater snail Clithon retropictus. Among them, the fraction eluted at 40–45 min showed the strongest toxicity among fractions. This fraction was subjected to further separation by a C18 RP-HPLC column (Fig. (b)). Each peak was tested for the toxicity, and the active peak A was separated. This was further purified using a different solvent system to obtain a single peptide (Fig. (c)). Injection of this peptide (about 1 μg) induced the paralysis in C. retropictus. Analysis using an ESI-ion trap (IT)-time-of-flight (TOF) mass spectrometer (LCMS-IT-TOF, Shimadzu, Kyoto, Japan) revealed that the active peptide has a monoisotopic molecular mass of 1775.6 Da. Aside from this peptide, the inactive peak B was isolated in the second purification step. This peak contained a single peptide with a monoisotopic molecular mass of 1851.6 Da (Fig. (d)).
Fig. 1. HPLC purification of the C. pennaceus venom. (a) First-step fractionation. Conditions; column, Protein C4, 10 × 250 mm, (Grace Vydac, Hesperia, CA, USA); solvents, 0.1% TFA in water/0.08% TFA in acetonitrile; flow rate, 2 ml/min; gradient, 5–60% of 0.08% TFA in acetonitrile over 55 min. The fraction eluted at 40–45 min (shaded) was obtained. (b) Second-step purification. Conditions; column, Everest C18 1.0 × 250 mm (Grace Vydac); solvents, 0.1% TFA in water/0.08% TFA in acetonitrile; flow rate, 0.05 ml/min; gradient, 10–50% of 0.08% TFA in acetonitrile over 40 min. (c) Final purification of peak A. Conditions; column, Everest C18, 1.0 x 250 mm (Grace Vydac); solvents, 0.1% formic acid in water/0.08% formic acid in acetonitrile; flow rate, 0.05 ml/min; gradient, 10–50% of 0.08% formic acid in acetonitrile over 40 min. (d) Final purification of peak B. Conditions are the same as described in (c).
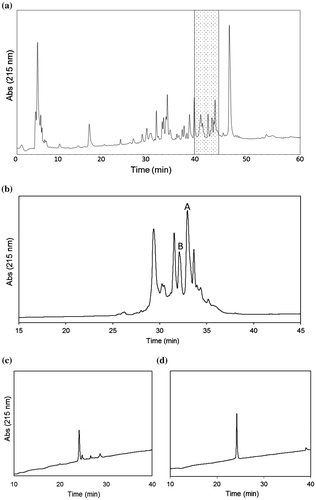
These two peptides were then subjected to structural determination by MS analysis. The peptides were treated sequentially with DTT and iodoacetamide for reduction and alkylation of the Cys side chains, which were analyzed by an ESI-IT-TOF mass spectrometer. After the reactions, a monoisotopic molecular mass of the active and inactive peptides increased to 2123.8 and 2199.8 Da, respectively. An increase in the molecular mass (348.2 Da) in both peptides corresponds to carbamoylmethylation of six Cys side chains (58.03 per Cys residue), indicating the presence of three disulfide bonds in both peptides. The primary structures of these peptides were then determined by MS/MS analysis.
First, the structure of the active peptide isolated from peak A was analyzed using a MALDI-TOF-TOF mass spectrometer. The fragment ions observed in the product ion spectrum were assigned to determine the sequence as CC(K/Q)YGWTCV(L/I)GCSPCGC (Fig. (a)). From this spectrum, discrimination between Lys and Gln residues at the third residue was not possible because they have a very small mass difference (0.036 Da). Discrimination between Leu and Ile residues, which have the same mass, at the 10th residue also could not be achieved due to the absence of side-chain fragmentation. To determine the third residue, MS/MS analysis was performed using an ESI-IT-TOF mass spectrometer, which can provide accurate m/z values sufficient for discrimination between Lys and Gln. The mean difference between calculated and measured m/z values of b3−b7 ions is 0.006 in the case of Lys, whereas that in the case of Gln is 0.030, allowing the assignment of the third residue as Lys (Fig. S1). On the other hand, assignment of the 10th residue was performed based on the sequence database searching. BLAST search analysis revealed that the peptide shares the same sequence with PnMMSK-D013, which was identified from the C. pennaceus venom grand only at the transcript levelCitation6). It is highly likely that the peptide identified in this study is the mature form of PnMMSK-D013. Therefore, the 10th residue was deduced as Leu based on the sequence of PnMMSK-D013, and the peptide was named pn4c according to the nomenclature of conotoxinsCitation3). The peptide pn4c showed the high sequence similarity to conotoxins PnIVA and PnIVB, which were identified from the C. pennaceus venom (Fig. )Citation7). In the present study, PnIVB was observed in the HPLC fraction eluted at 45–50 min, whereas PnIVA was not detected, probably due to its low abundance in the venom used.
Fig. 2. Sequence determination by MALDI-TOF-TOF MS analysis. (a) Product ion spectrum of pn4c. (b) Product ion spectrum of pn3a.
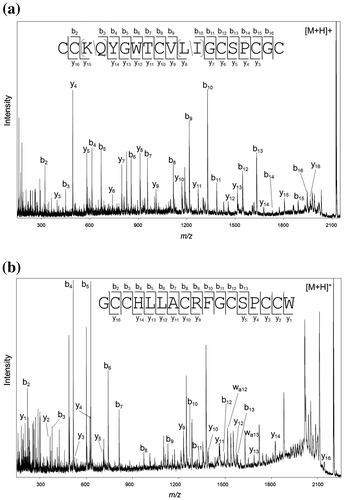
Fig. 3. Comparison of the amino acid sequences. (a) pn4c and its similar peptides. PnIVA and PnIVB were previously identified from the C. pennaceus venom. (b) pn3a and its similar peptides. mr3b and conotoxin1 were previously identified from the C. marmoreus and C. textile venom, respectively. O, 4-hydroxyproline.
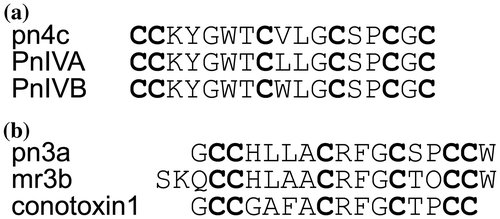
Next, the primary structure of the inactive peptide isolated from peak B was determined in a similar fashion. The result obtained by MALDI-TOF-TOF MS analysis allowed the determination of its sequence as GCCHLLACRFGCSPCCW (Fig. (b)). In this case, Leu/Ile discrimination at the fifth and sixth residues was achieved by observation of fragment ions generated through side-chain fragmentation (wa12 and wa13 ions). This assignment was further confirmed by ESI-IT-TOF MS analysis (Fig. S1). This is the first example of conopeptides with the cysteine framework III from the C. pennaceus venom, and named pn3a. Conopeptides similar to pn3a were identified from the venom of other snail-hunting species (Fig. ), but unlike pn4c, similar peptides were also found from fish-hunting or worm-hunting Conus species. This suggests that peptides belong to this family have some general functions rather than those specific to their feeding animals.
To confirm the structure and activity of pn4c and pn3a, the peptides were chemically synthesized using the Fmoc-based solid-phase peptide synthesis (SPPS) technique. Cl-Trt(2-Cl) resin or Wang resin was used for synthesis of pn4c and pn3a, respectively. Elongation of the peptide chain was carried out using 1-[(1-(cyano-2-ethoxy-2-oxoethylideneaminooxy) dimethylaminomorpholino)] uronium hexafluorophosphate (COMU) as a coupling reagent. After completion of peptide chain elongation, the peptides were cleaved from the resin with TFA containing scavengers (5% phenol, 5% thioanisole, 2.5% ethanedithiol, 1% triisopropylsilane, and 5% water). The resultant peptides were purified by RP-HPLC. The formation of disulfide bonds was carried out using following conditions; for pn4c, Tris-HCl buffer (pH 8.0) containing 2 mM reduced glutathione, 0.2 mM oxidized glutathione, and 3 M guanidine at the peptide concentration of 0.02 mg/ml for 24 h at room temperature; for pn3a, Tris-HCl buffer (pH 8.0) containing 2 mM reduced glutathione, 0.2 mM oxidized glutathione, 3 M guanidine, and 40% DMSO at the peptide concentration of 0.3 mg/ml for 24 h at 4 °C. In each case, a peptide having a molecular mass of the fully oxidized form was observed as a major peak in LC/MS analysis after the reaction, which were purified by RP-HPLC and lyophilized. Both synthetic and natural sample showed the same retention times in HPLC analysis (Fig. S2). This result indicates that the synthesized peptides are identical to natural ones, although the disulfide linkage pattern in each peptide remains to be determined.
The biological activity of pn4c and pn3a was examined using the freshwater snail C. retropictus (~500 mg body weight). When pn4c dissolved in water was injected into the foot of the snail at a dose of 0.2–2 nmol/snail (0.35–3.5 μg/snail), more than 80% of tested snails dropped from the wall after 5 min, and their foot muscles shrank. This result is consistent with that obtained using natural pn4c, which induced paralysis at a dose of approximately 1 μg/snail. The paralysis was not significantly observed (10%) when 0.1 nmol of pn4c was injected, indicating that the 50% paralytic dose (PD50) of pn4c against C. retropictus is approximately 0.2–0.4 nmol/g. This toxicity is comparable to that of other anti-mollusk conotoxins, such as PnIVA, which has the PD50 of 0.34 nmol/g, although different mollusk species (Mytilus edulis) was used for the bioassay in that studyCitation8). Since the conotoxin PnIVA is known to act on sodium channels of freshwater snails (Lymnaea stagnalis), it is likely that the toxicity of pn4c is caused by the same mechanisms. In contrast, pn3a was completely inactive even after injection of 2 nmol of the sample, as expected. This indicates that pn3a has biological functions other than anti-mollusk toxicity in the venom. The toxins identified in this study will not only serve as a novel pharmacological tool for functional studies on ion channels, but also help understanding biological roles of each peptide in the Conus venom.
Author contributions
M.A.-W. performed the experiments; M.M., M.A.-R., and Y.N. designed the research; M.M., and R.O. analyzed the mass spectrometric data; H.J., and Y.O. assisted the experiments; M.S. assisted the interpretation of data; M.F. supported the collection of cone snails; S.S. supervised the research; M.A.-W, M.M., and Y.N. wrote and revised the manuscript.
Disclosure statement
No potential conflict of interest was reported by the authors.
Funding
This study was supported in part by a scholarship from the Egyptian Ministry of Higher Education, Cultural Affairs and Missions sector according to a joint supervision program between Egypt and Japan.
Supplemental material
The supplemental material for this article is available at https://doi.10.1080/09168451.2017.1364966.
Supplement_Fig.pdf
Download PDF (264.3 KB)Acknowledgments
The authors are grateful to Mr. A. Yassein in National Institute of Oceanography and Fisheries (NIOF) for his assistance in cone snail collection.
References
- Akondi KB, Muttenthaler M, Dutertre S, et al. Discovery, synthesis, and structure–activity relationships of conotoxins. Chem Rev. 2014;114:5815–5847.10.1021/cr400401e
- Lewis RJ, Dutertre S, Vetter I, et al. Conus venom peptide pharmacology. Pharmacol Rev. 2012;64:259–298.10.1124/pr.111.005322
- Mir R, Karim S, Kamal MA, et al. Conotoxins: structure, therapeutic potential and pharmacological applications. Curr Pharm Des. 2016;22:582–589.10.2174/1381612822666151124234715
- Fainzilber M, Gordon D, Hasson A, et al. Mollusc-specific toxins from the venom of Conus textile neovicarius. Eur J Biochem. 1991;202:589–595.10.1111/ejb.1991.202.issue-2
- Aman JW, Imperial JS, Ueberheide B, et al. Insights into the origins of fish hunting in venomous cone snails from studies of Conus tessulatus. Proc Natl Acad Sci USA. 2015;112:5087–5092.10.1073/pnas.1424435112
- Conticello SG, Gilad Y, Avidan N, et al. Mechanisms for evolving hypervariability: the case of conopeptides. Mol Biol Evol. 2001;18:120–131.10.1093/oxfordjournals.molbev.a003786
- Fainzilber M, Nakamura T, Gaathon A, et al. A new cysteine framework in sodium channel blocking conotoxins. Biochemistry 1995;34:8649–8656.10.1021/bi00027a014
- Fainzilber M, van der Schors R, Lodder JC, et al. New sodium channel-blocking conotoxins also affect calcium currents in lymnaea neurons. Biochemistry 1995;34:5364–5371.10.1021/bi00016a007