Abstract
The naturally occurring anthraquinone emodin has been serving primarily as an anti-bacterial and anti-inflammatory agent. However, little is known about its potential on anti-aging. This investigation examined the effect of emodin on lifespan and focused on its physiological molecular mechanisms in vivo. Using Caenorhabditis elegans (C. elegans) as an animal model, we found emodin could extend lifespan of worms and improve their antioxidant capacity. Our mechanistic studies revealed that emodin might function via insulin/IGF-1 signaling (IIS) pathway involving, specifically the core transcription factor DAF-16. Quantitative RT-PCR results illustrated that emodin up-regulated transcription of DAF-16 target genes which express antioxidants to promote antioxidant capacity and lifespan of worms. In addition, attenuated effect in sir-2.1 mutants suggests that emodin likely functioned in a SIR-2.1-dependent manner. Our study uncovers a novel role of emodin in prolonging lifespan and supports the understanding of emodin being a beneficial dietary supplement.
Emodin promotes lifespan and antioxidant capacity of C. elegans through insulin/IGF-1 signaling pathway depending on DAF-16 and SIR-2.1.
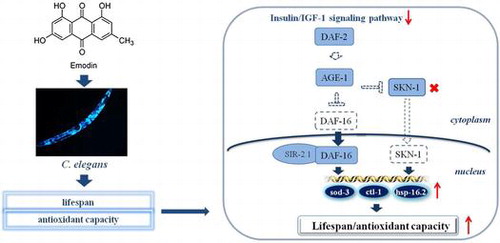
Aging is a natural process that can be hastened with accumulations of oxidative damage at molecular and cellular levels.Citation1,2) In recent decades, our environment and the abuse of it have contributed significantly to this hastening process.Citation3,4) Diseases induced by oxidative damage, and particularly those related to aging, have increasingly become a major health concern in everyday life. Valiant efforts from pharmaceutical industries around the world have yielded clinically viable therapeutics, but they are of high cost or with high health risk. The naturally occurring herbs and herbal-based products have been known for centuries for their multiple health benefits, and posing minimal health risks to animals and humans as well as low impact to our environment.Citation5) Therefore, developing therapeutics based on these herbal origins to combat aging and age-related diseases represents a promising endeavor.
Emodin, a naturally occurring anthraquinone isolated from roots and barks of a wide range of medicinal herbs, has long been recognized clinically as a useful anti-bacterial and anti-inflammatory agent.Citation6,7) Emodin also functions in a variety of important physiological processes that would include regulations of redox balance.Citation8) It has been noted that emodin’s role as an antioxidant is concentration-dependent.Citation9) In grass carp hepatic cell experiments, emodin predominantly acts as an antioxidant at low dosages (≤1 μg/mL), but behaves as a pro-oxidant at higher dose range of 1–25 μg/mL.Citation10) Nevertheless, studies have confirmed that at concentrations of which it is considered as an antioxidant, emodin shows potential to protect organisms from damage inflicted by reactive oxygen species (ROS), thereby revealing a protective effect on oxidation stress-related diseases, such as smoke-induced lung injury and high fat-induced obesity.Citation11,12) ROS has been implicated as a major culprit in the aging process because they can induce damage at molecular and cellular levels. Multiple studies have indicated that either reducing ROS production or increasing antioxidant capacity could extend lifespan of animals.Citation13,14)
The ability of emodin to act as an antioxidant led us to believe that it could influence lifespan. Given our longstanding interest in the invertebrate animal model Caenorhabditis elegans (C. elegans), we envisioned such potential effect on longevity could be assessed using C. elegans. Tissenbaum et al. have revealed that longevity genes and signaling pathways between these nematodes and human are highly conserved.Citation15) Recent reports have also linked the onset of diabetes to oxidative stresses and ROS, and restricting the insulin/IGF-1 signaling (IIS) pathway has been found to improve resistance to oxidative stress.Citation16) More intriguingly, reducing IIS not only elevated insulin resistance but also extended lifespan of C. elegans.Citation17) Although the underpinning mechanism remains unclear, the observed extension of lifespan is likely related to the reduced oxidative injuries. In particular, under reduced IIS conditions, it is associated with the conserved core transcription factors, such as the forkhead box O transcription factor DAF-16/FoxO and the Nrf-like xenobiotic-response factor SKN-1/Nrf-2.Citation18,19) In concert with the IIS pathway, DAF-16/FoxO is also regulated by overexpressed SIR-2.1/SirT1 in the nucleus. As the cofactor of DAF-16, SIR-2.1/SirT1 forms a complex with DAF-16 in nucleus, and such activation of DAF-16 then promotes lifespan and stress resistance of C. elegans.Citation20) Therefore, enhancing the activity of transcription factor DAF-16 could be a key to improving oxidative stress resistance and extending lifespan of C. elegans.
In C. elegans, gene daf-2 encodes an insulin/IGF-1 receptor-like protein, and its mutation leads to extension of lifespan. Gene age-1 encodes phosphoinositide 3-kinase, which is downstream of DAF-2, and its mutation extends lifespan. Gene daf-16 encodes the FoxO transcription factor downstream of IIS, while gene skn-1 encodes the Nrf-2 transcription factor. A single mutation of either daf-16 or skn-1 shortens lifespan. Gene sir-2.1 encodes NAD-dependent protein deacetylase, and its mutation leads to decreased stress tolerance and shortened lifespan.Citation21) Gene eat-2 encodes neuronal acetylcholine receptor, and its mutation leads to defective of pharyngeal pumping and extension of lifespan.Citation22) For studies regarding molecular mechanism, loss-of-function mutants were utilized. We report herein our data in determining the ability of emodin to extend lifespan of C. elegans and its molecular mechanisms.
Materials and methods
Materials, genes and growth condition
Emodin (98%, Guangrun Biotechnology Co., Ltd., Nanjing, China) was dissolved in DMSO (Sigma-Aldrich, St. Louis, Missouri, USA). 0.01% (v/v) DMSO was used in all assays as the solvent control. Fluorescence probe H2DCF-DA (2′,7′-dichlorofluorescein diacetate) was purchased from Beyotime Biotechnology Co., Ltd., Jiangsu, China. RNA prep Pure Kit was purchased from Tiangen Biotechnology Co., Ltd., Beijing, China. Strains used in this study were Bristol N2 (wild type), CB1370 daf-2 (e1370) III, TJ1052 age-1 (hx546) II, GR1307 daf-16 (mgDf50) I, EU35 skn-1(zu169) IV/nT1 [unc-?(n754) let-?] (IV;V), TJ356 zIs356 [daf-16p::daf-16a/b::gfp; rol-6], LD1 ldIs7 [skn-1b/c::gfp; rol-6], VC199 sir-2.1(ok434) IV, DA1116 eat-2(ad1116) II. All strains and E. coli OP50 were purchased from Caenorhabditis Genetics Center at University of Minnesota, Minneapolis, MN, USA. Nematodes were cultured and assayed (except for the stress assay) at 20 °C on NGM with a lawn of E. coli OP50 as the food source according to standard procedures reported by Harper.Citation23) Synchronized L4 stage worms were utilized in all assays, and they were all cultured from synchronized L1 larvae obtain by bleaching worms. The synchronized L1 larvae were obtained through sodium hypochlorite treatment of gravid adults, and were subsequently cultured at 20 °C on NGM plates for 36 h (hours) to obtain a population of mainly L4 larvae.
Lifespan assay
Synchronized L4 worms were transferred to NGM plates containing different concentrations of emodin (concn = 0, 50, 150, and 300 μM). 5-FUdR (12.5 mg/L) was added into solid NGM plates for all lifespan assays to block progeny development. NGM plates were covered with heat-killed OP50 (inactivated at 65 °C for 30 min) mixed with emodin (0, 50,150, and 300 μM) as the food source. The first day C. elegans exposed to emodin was counted as day-1. Dead worms were counted daily, while the living worms were transferred to new plates every four d (days). Worms were considered as dead when they did not respond to the gentle touching with a flexible picker tip. The accidental dead, internal hatched or lost animals were excluded. Approximately, 50 worms were used in each lifespan assay, and each assay was performed at least three times independently.
Stress tolerance
Synchronized L4 larvae were treated with or without 150 μM of emodin on NGM plates for three d. For the oxidative resistance assay, the three-day-old worms were transferred to NGM plates containing 30 mM of paraquat, and the mortality rate was scored at 24, 48, 72, 96, and 120 h. For the acute thermal tolerance test, three-day-old worms were transferred and incubated at 37 °C, and their mortality rate was scored each hour after the experiment commenced. With regards to scoring for oxidative and thermal resistance capacity, we considered paralyzed worms as dead. This assay was performed at least three times independently.
Intracellular ROS level
ROS levels of C. elegans were measured using fluorescence probe H2DCF-DA (2′,7′-dichlorofluorescein diacetate) as the indicator. Synchronized L4 larvae were bred on NGM plates with or without emodin. At the adult day-three, five worms were washed by 0.1% PBST (1 × PBS/0.1% Tween 20), and then suspended with 80 μL of PBS in a black 96-well plate. Subsequently, 50 μM of H2DCF-DA was added to each well. While being kept at 37 °C, the changes in fluorescent intensity were measured by DCF fluorescence (Excitation wavelength: 485 nm; Emission wavelength: 535 nm) at 0, 15, 30, 60, 90, and 120 min. This test was performed at least three times.
Sub-cellular DAF-16 and SKN-1 distribution
Synchronized L4 stage TJ356 (DAF-16::GFP) and LD1 (SKN-1::GFP) were cultured on NGM plates with or without 150 μM of emodin for 2 h and three d. Subsequently, worms were mounted on microscope slides coated with 2% agarose and anesthetized with 10 μL of 1 mM imidazole. The resulting slides were covered with a slideglass and monitored by fluorescence microscopy (Nikon-eclipse 80i) at 200-fold and 400-fold magnification. According to sub-cellular distribution of green fluorescence, the worms were categorized as nuclear, intermediate, and cytosolic.Citation12,24) Worms with more than 20 nuclear fluorescence dots were categorized as nuclear, while without nuclear fluorescence dots were categorized as cytosolic. Worms in the mid state were counted as intermediate. The number of worms in each category was counted. Approximately, 30 worms were used in each trial, and this assay was repeated at least four times.
Quantitative real-time PCR (RT-PCR)
Synchronized L4 worms were treated with or without 150 μM of emodin for three d. The total RNA was extracted by RNA prep Pure Kit, and 1 μg of total RNA was used per reaction to produce cDNA using Quantscript RT Kit. cDNA products were stored at –20 °C. For RT-PCR to detect the levels of mRNA, ~200 ng of cDNA was utilized per group on the Light Cycler (Roche) system with SYBR green as the detection method. The expression data were analyzed by the comparative 2-ΔΔCt method with act-1 as the internal control. This test was performed for more than three independent trials. Primers used in RT-PCR are as follows: daf-16: forward 5′-CCAGACGGAAGGCTTAAACT-3′, and reverse 5′-ATTCGCATGAAACGAGAATG-3′; sod-3: forward 5′-CGAGCTCGAACCTGTAATCAGCCATG-3′, and reverse 5′-GGGGTACCGCTGATATTCTTCCAGTTG -3′; hsp-16.2: forward 5′-CTGCAGAATCTCTCCATCTGAGTC-3′, and reverse 5′-AGATTCGAAGCAACTGCACC-3′; ctl-1: forward 5′-CGGATACCGTACTCGTGATGAT-3′, and reverse 5′-CCAAACAGCCACCCAAATCA-3′; act-1: forward 5′-CCAGGAATTGCTGATCGTATGCAGAA-3′, and reverse 5′-TGGAGAGGGAAGCGAGGATAGA-3′.
Statistical analysis
All data were expressed as lifespan ± SEM. Statistical analyses for lifespan and stress resistance assays were performed by Kaplan–Meier survival analysis. Statistical calculations were performed using SPSS 18.0 and Origin 8.0 software. Significant differences between the experimental and control groups were calculated by log-rank test, one-way ANOVA or Student’s t-test. Differences with *p < 0.05 and **p < 0.01 were considered statistically significant.
Results
Emodin extended the lifespan of wild-type C. elegans
To determine the effect of emodin on the lifespan of C. elegans, viabilities of worms with 0, 50, 150, and 300 μM emodin treatment were assessed under normal culture condition. Results showed that emodin extended the lifespan of nematodes at concentrations of 50 and 150 μM (Fig. (A) and Table ). Specially, worms treated with 150 μM of emodin exhibited the maximal effect on the lifespan extension. However, emodin had little effect on the lifespan at 300 μM. Based on this result, all subsequent assays were performed with 150 μM of emodin.
Fig. 1. Lifespan and antioxidant capacity of C. elegans treated with or without emodin.
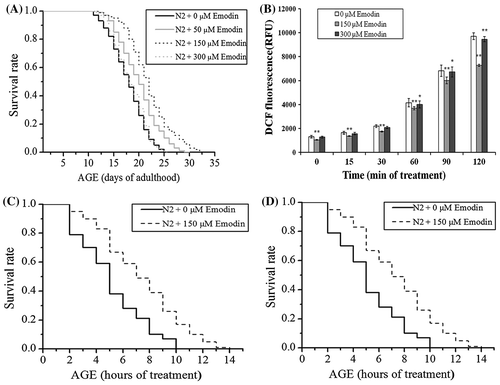
Table 1. Effect of emdoin on the lifespan of wild-type C. elegans.
Emodin improved antioxidant capacity of C. elegans
High levels of ROS generation can lead to oxidative stress and accelerate the aging process. The ROS scavenging capability of 150 μM emodin in the cells was quantified using ex-vivo DCFH-DA assay. To figure out whether high concentration of emodin at 300 μM is pro-oxidant, the ROS levels in worms treated with 300 μM emodin were also measured. As shown in Fig. (B), there was a significant reduction of ROS in N2 worms treated with 150 μM of emodin, while the ROS were slightly reduced in worms with 300 μM of emodin treatment. To explore the effect of emodin on oxidative stress resistance of worms, we then assessed the ability of emodin to recover the thrashing movement of worms under oxidative conditions. Paraquat is a free-radical-generating compound that can induce acute oxidative stress in the cells, while heat shock can also increase intracellular oxidative injuries. As shown in Fig. (C) and (D), the emodin treatment improved the survival rate of C. elegans under either paraquat or heat shock stress conditions (Table ).
Table 2. Effect of emodin on the stress resistance of wild-type C. elegans.
Emodin promoted lifespan and daf-16 transcription via IIS pathway
It’s reported that IIS pathway plays a dominant role in aging-related signaling pathways, and the reduction of IIS by various methods has been found to extend lifespan and enhance stress tolerance both in mammals and C. elegans. Single mutations of daf-2, age-1, or daf-16 lead to inhibition of the IIS pathway, and mutant strains of daf-2 (e1370), age-1 (hx546), and daf-16 (mgDf50) are IIS-inhibited, and thus, possess longevity capability. To uncover the role of IIS pathway in the emodin-induced lifespan extension, lifespan of IIS-inhibited daf-2 (e1370), age-1 (hx546), and daf-16 (mgDf50) mutant strains were assessed with or without emodin treatment. Under reduced IIS conditions, emodin was unable to promote the lifespan of these mutant worms in comparison with the control group (Figs. (A)–(C), and Table ). To further investigate the functional relationship between emodin and IIS pathway, we examined the daf-16 transcription changes in N2 worms and daf-2 (e1370) mutants with act-1 as internal control. Through RT-PCR analysis, there was an up-regulation of daf-16 mRNA in the emodin-treated N2 worms, whereas it showed an equal level of daf-16 mRNA in the emodin-treated and -untreated daf-2 (e1370) mutants (Fig. (D)). These data suggested that emodin promoted the lifespan and daf-16 transcription through IIS pathway.
Fig. 2. Emodin’s effect on lifespan of IIS-inhibited worms and daf-16 transcription.
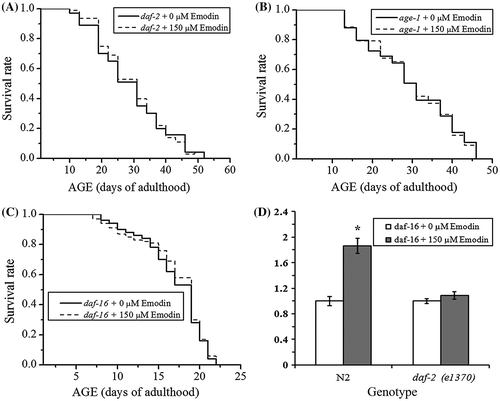
Table 3. Effect of emodin on the lifespan of mutant worms.
DAF-16 was involved in emodin-modulated longevity
It’s reported that transcription factors DAF-16 and SKN-1 function primarily downstream of the IIS pathway to modulate lifespan of C. elegans. To determine the effect of emodin on DAF-16 and SKN-1, transgenic strains TJ356 and LD1 were utilized to observe DAF-16 and SKN-1 nuclear translocation activities. Worms were categorized as nuclear, intermediate, and cytosolic according to DAF-16::GFP and SKN-1::GFP sub-cellular distribution. As in Fig. (C), emodin treatment increased the nuclear localization percentage of TJ356 at 2 h (control: 18.94%; emodin: 48.36%) and three d (control: 31.63%; emodin: 61.22%). However, the nuclear and intermediate localization percentage of LD1 showed no statistical significance difference between emodin treatment and control group (Fig. (C)). Subsequently, effect of emodin on the lifespan of skn-1 (zu169) mutants was assessed. In contrast to the abolished longevity in daf-16 (mgDf50) mutants (Fig. (C) and Table ), prolonged lifespan was detected in emodin-treated skn-1 (zu169) mutants (Fig. (D) and Table ). These results revealed that DAF-16 could be responsible for the emodin-mediated lifespan extension in wild-type nematodes, while emodin did not appear to activate SKN-1 in vivo.
Fig. 3. Influence of emodin on DAF-16 and SKN-1.
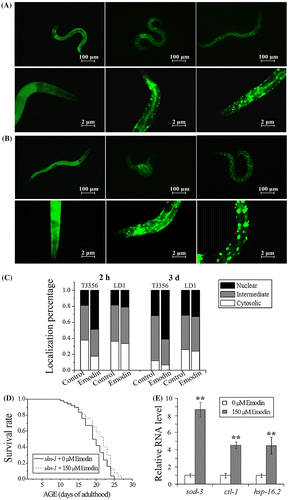
Emodin up-regulated transcription of aging-related genes
Genes sod-3, ctl-1, and hsp-16.2 are the longevity related and downstream of DAF-16, which express anti-oxidative and anti-thermal enzymes to enhance the stress tolerance of C. elegans. Through RT-PCR analysis, it showed that emodin up-regulated mRNA levels of sod-3, ctl-1, and hsp-16.2 by 8.75, 4.53, and 4.50-fold, respectively, with act-1 as the internal control (Fig. (E)). Up-regulations of these downstream genes implied that emodin treatment indeed activated the transcription factor DAF-16.
Emodin extends lifespan and enhances stress resistance in SIR-2.1-dependent manner
To determine whether SIR-2.1 was involved in the emodin-modulated longevity of C. elegans, effect of emodin on the lifespan of sir-2.1 (ok434) mutants was measured under normal culture condition. As shown in Fig. (A) and Table , the lifespan extension effect of emodin was significantly reduced in the sir-2.1 (ok434) mutants (68.67% reduced).
Fig. 4. Effect of emodin on survival of sir-2.1 (ok434) and eat-2 (ad1116).
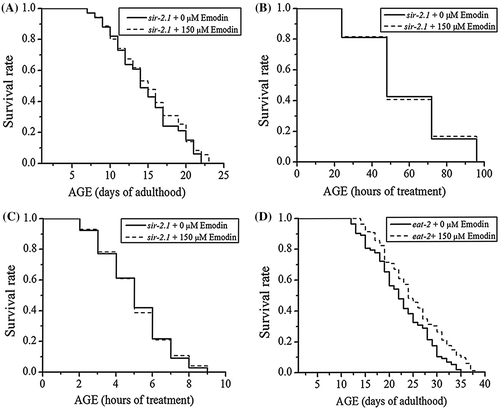
Since the ability of emodin extending lifespan appeared to be dependent upon SIR-2.1, we anticipated that the promoted stress resistance by emodin could be similarly associated with SIR-2.1. As expected, emodin did not improve survival of sir-2.1 (ok434) mutant worms under the paraquat-induced acute oxidative stress condition (Fig. (B) and Table ). Similarly, emodin also failed to improve the resistance to thermal stress in sir-2.1 (ok434) mutants (Fig. (C) and Table ). Therefore, SIR-2.1 appeared to be involved both in the emodin-promoted lifespan and stress tolerance.
Table 4. Effect of emodin on the stress resistance of sir-2.1 (ok434).
Longevity effect of emodin was not based on Calorie Restriction (CR) mechanism
To understand whether emodin extended lifespan through CR, emodin’s effect on the lifespan of pharyngeal pumping defective eat-2 (ad1116) mutants were measured. As shown in Fig. (D), eat-2 (ad1116) mutants with emodin treatment showed significant lifespan extension compared to the controls. Therefore, the CR could be excluded from the molecular mechanism of emodin-induced longevity.
Discussion
High levels of ROS and imbalance of redox system have been implicated as major contributors to aging and degenerative disease. Citation25) With air pollution becoming increasingly worse, damage due to exposure to oxidative free radicals has become a chronic issue for all aerobic organisms under any physiological conditions. Recent reports suggested that the increased expression of anti-aging genes could significantly enhance stress resistance and increase survival of animals.Citation26,27) Therefore, developing products that improve antioxidant genes expression has received much attention in recent years. The natural products, especially those with antioxidant properties, have become invaluable resources to combat oxidative injuries because they are environmentally benign and minimize health risks to animals and humans.
The naturally occurring anthraquinone emodin has been known for its anti-bacterial and anti-inflammatory activities. It is also capable of moderating oxidative damage through regulating redox balance when administered at an optimal concentration. In this study, we investigated the potential longevity effect of emodin in C. elegans and explored possible underpinning mechanisms contributing to this effect. Our data suggested that emodin up-regulated transcription of antioxidant genes and extended lifespan of C. elegans, and that such activity was associated with the IIS pathway and depended upon DAF-16 and SIR-2.1.
The potency of emodin on lifespan extension was measured in N2 worms, and the mean lifespan of worms was extended at the optimal concentration of 150 μM. For emodin’s ability to regulate redox balance, such lifespan promotion effect was likely a result of improved anti-oxidant capacity. With 150 μΜ emodin treatment, ROS production of worms was significantly decreased. It previously demonstrated that emodin functioned as pro-oxidant at high levels.Citation9) However, there was not a pro-oxidant state shift of emodin at 300 μM. Under paraquat and heat shock-induced oxidative stress conditions, a remarkable increase in the stress tolerance was observed in emodin treatment group. For the molecular damage occurred under oxidative stress was consistent with the injuries seen with aging, the emodin-reduced ROS and the improved stress tolerance were closely associated with the extended lifespan of C. elegans.
IIS pathway was not only responsible for extending the lifespan but also related to enhanced stress resistance.Citation28) However, emodin was unable to increase lifespan of reduced IIS daf-2 (e1370), age-1 (hx546) and daf-16 (mgDf50) mutants. Reports have shown that DAF-16/FoxO is necessary for lifespan extension in response to reduced IIS and that its overexpression extends lifespan.Citation29,30) Through RT-PCR analysis, emodin increased the mRNA level of daf-16 in wild-type N2 worms, whereas such effect was diminished in daf-2 (e1370) mutants with reduced IIS. Our data implied the involvement of IIS pathway in emodin-promoted lifespan and daf-16 transcription.
Under reduced IIS conditions, nuclear localized DAF-16 and SKN-1 have overlapping as well as independent functions in modulating lifespan of worms.Citation31,32) With emodin treatment, there was increased nuclear translocation of DAF-16::GFP instead of SKN-1::GFP. Consistent with this result, mutation of skn-1 gene did not attenuate the longevity effect of emodin in vivo, while this longevity effect was completely abolished in daf-16 (mgDf50). This contrast demonstrated that the longevity effect of emodin was modulated only by DAF-16. Downstream from DAF-16, genes sod-3, ctl-1 and hsp-16.2 express antioxidants which protect cells from oxidative and thermal injuries.Citation33) With emodin treatment, the genes transcription was up-regulated, which further supported the role of emodin in activating DAF-16. In addition, the increased antioxidant genes transcription was consistent with the improved viability under stress and normal conditions.
As cofactor of DAF-16, SIR-2.1 plays vital roles in promoting lifespan and stress resistance of worms.Citation34,35) It is therefore significant to note that the mutation of sir-2.1 attenuated the longevity effect of emodin, and the same mutation almost completely abolished the emodin-enhanced stress resistance. Thus, emodin promoted the lifespan and antioxidant capacity in a SIR-2.1-dependent manner.
CR has been implicated in reducing oxidative damage and delaying the aging process for a range of organisms.Citation36) However, emodin extended the lifespan of CR-constitutive eat-2 (ad1116) mutants, thereby excluding the CR mechanism in the emodin-induced longevity.
Conclusion
In summary, we have reported here that emodin up-regulated antioxidant genes transcription, promoted the antioxidant capacity and lifespan of C. elegans. Such effect of emodin was associated with the IIS pathway and depended upon DAF-16 as well as SIR-2.1. In addition, the CR mechanism study illustrated that emodin could protect C. elegans from oxidation induced damage and senescence in a relatively healthy and safe manner. This work provides strong evidence that emodin could serve as a novel and viable anti-aging agent, and suggest it as a beneficial dietary supplement.
Authors’ contributions
This study was conceived and designed by all authors. Lab works was carried out by Xuan Zhao, Lulu Lu, and Miao Li. Data were analyzed by Xuan Zhao and Yonghao Qi. The manuscript was written by Xuan Zhao in consultation with all authors. All authors accepted the final version of the manuscript.
Funding
This study was supported by the National Natural Science Foundation of China [grant number 81641132].
Disclosure statement
The authors declare that they have no conflict of interest.
Supplemental materials
The supplemental material for this paper is available at https://doi.org/10.1080/09168451.2017.1365592.
Supporting_Information.docx
Download MS Word (213.5 KB)Acknowledgments
Authors would like to thank Professor Richard P. Hsung of University of Wisconsin–Madison for invaluable discussions and the preparation of this manuscript. Authors would like to thank The National Natural Science Foundation of China (81641132) for invaluable financial support of this work.
References
- Ghosh D, LeVault KR, Barnett AJ, et al. A reversible early oxidized redox state that precedes macromolecular ROS Damage in aging nontransgenic and 3xTg-AD mouse neurons. J Neurosci. 2012;32:5821–5832.10.1523/JNEUROSCI.6192-11.2012
- Harman D. Aging: a theory based on free radical and radiation chemistry. J Gerontol. 1956;11:298–300.10.1093/geronj/11.3.298
- Finch CE, Beltrán-Sánchez H, Crimmins EM. Uneven futures of human lifespans: reckonings from Gompertz mortality rates, climate change, and air pollution. Gerontology. 2014;60:183–188.10.1159/000357672
- Risom L, Møller P, Loft S. Oxidative stress-induced DNA damage by particulate air pollution. Mutat Res. 2005;592:119–137.10.1016/j.mrfmmm.2005.06.012
- Katiki LM, Ferreira JF, Gonzalez JM, et al. Anthelmintic effect of plant extracts containing condensed and hydrolyzable tannins on Caenorhabditis elegans, and their antioxidant capacity. Vet Parasitol. 2013;192:218–227.10.1016/j.vetpar.2012.09.030
- Alisi A, Pastore A, Ceccarelli S, et al. Emodin prevents intrahepatic fat accumulation, inflammation and redox status imbalance during diet-induced hepatosteatosis in rats. Int J Mol Sci. 2012;13:2276–2289.10.3390/ijms13022276
- Dong X, Fu J, Yin X, et al. Emodin: a review of its pharmacology, toxicity and pharmacokinetics. Phytother Res. 2016;30:1207–1218.10.1002/ptr.v30.8
- Yen GC, Duh PD, Chuang DY. Antioxidant activity of anthraquinones and anthrone. Food Chem. 2012;70:437–441.
- Tian B, Hua Y. Concentration-dependence of prooxidant and antioxidant effects of aloin and aloe-emodin on DNA. Food Chem. 2005;91:413–418.10.1016/j.foodchem.2004.06.018
- Cui YT, Liu B, Xie J, et al. The effect of emodin on cytotoxicity, apoptosis and antioxidant capacity in the hepatic cells of grass carp (Ctenopharyngodon idellus). Fish Shellfish Immunol. 2014;38:74–79.10.1016/j.fsi.2014.02.018
- Tzeng TF, Lu HJ, Liou SS, et al. Emodin protects against high-fat diet-induced obesity via regulation of AMP-activated protein kinase pathways in white adipose tissue. Plant Med. 2012;78:943–950.
- Wang X, Zhang J, Lu L, et al. The longevity effect of echinacoside in Caenorhabditis elegans mediated through daf-16. Biosci Biotechnol Biochem. 2015;79:1676–1683.10.1080/09168451.2015.1046364
- Lithgow GJ, Walker GA. Stress resistance as a determinate of C. elegans lifespan. Mech Ageing Dev. 2002;123:765–771.10.1016/S0047-6374(01)00422-5
- Zhang J, Lu L, Zhou L. Oleanolic acid activates daf-16 to increase lifespan in Caenorhabditis elegans. Biochem Biophys Res Commun. 2015;468:843–849.10.1016/j.bbrc.2015.11.042
- Tissenbaum HA. Using C. elegans for aging research. Invertebr Reprod Dev. 2015;59:59–63.10.1080/07924259.2014.940470
- Rains JL, Jain SK. Oxidative stress, insulin signaling, and diabetes. Free Radic Biol Med. 2011;50:567–575.10.1016/j.freeradbiomed.2010.12.006
- Altintas O, Park S, Lee SJ. The role of insulin/IGF-1 signaling in the longevity of model invertebrates, C. elegans and D. melanogaster. BMB Rep. 2016;49:81–92.10.5483/BMBRep.2016.49.2.261
- Tullet JM, Hertweck M, An JH, et al. Direct inhibition of the longevity-promoting factor SKN-1 by insulin-like signaling in C. elegans. Cell. 2008;132:1025–1038.10.1016/j.cell.2008.01.030
- Wu JZ, Huang JH, Khanabdali R, et al. Pyrroloquinoline quinone enhances the resistance to oxidative stress and extends lifespan upon DAF-16 and SKN-1 activities in C. elegans. Exp Gerontol. 2016;80:43–50.10.1016/j.exger.2016.04.008
- Berdichevsky A, Guarente L. A stress response pathway involving sirtuins, forkheads and 14-3-3 proteins. Cell Cycle. 2006;5:2588–2591.10.4161/cc.5.22.3513
- Murphy CT, Hu PJ. Insulin/insulin-like growth factor signaling in C. elegans. WormBook. 2013;1–43.
- McKay JP, Raizen DM, Gottschalk A, et al. eat-2 and eat-18 are required for nicotinic neurotransmission in the caenorhabditis elegans pharynx. Genetics. 2004;166:161–169.10.1534/genetics.166.1.161
- Harper JM, Salmon AB, Chang Y, et al. Stress resistance and aging: Influence of genes and nutrition. Mech Ageing Dev. 2006;127:687–694.10.1016/j.mad.2006.04.002
- Ray A, Martinez BA, Berkowitz LA, et al, Mitochondrial dysfunction, oxidative stress, and neurodegeneration elicited by a bacterial metabolite in a C. elegans Parkinson’s model. Cell Death Dis. 2014;5:e984.10.1038/cddis.2013.513
- Sohal RS, Orr WC. The redox stress hypothesis of aging. Free Radic Biol Med. 2012;52:539–555.10.1016/j.freeradbiomed.2011.10.445
- Ogawa T, Kodera Y, Hirata D, et al. Natural thioallyl compounds increase oxidative stress resistance and lifespan in Caenorhabditis elegans by modulating SKN-1/Nrf. Sci Rep. 2016;6:13.10.1038/srep21611
- Rathor L, Akhoon BA, Pandey S, et al, Folic acid supplementation at lower doses increases oxidative stress resistance and longevity in Caenorhabditis elegans. Age (Dordr). 2015;37:113.
- Lin K, Hsin H, Libina N, et al. Regulation of the Caenorhabditis elegans longevity protein DAF-16 by insulin/IGF-1 and germline signaling. Nat Genet. 2001;28:139–145.10.1038/88850
- Bansal A, Kwon ES, Conte D Jr, et al. Transcriptional regulation of Caenorhabditis elegans FOXO/DAF-16 modulates lifespan. Longev Healthspan. 2014;3:5.10.1186/2046-2395-3-5
- Henderson ST, Johnson TE. daf-16 integrates developmental and environmental inputs to mediate aging in the nematode Caenorhabditis elegans. Curr Biol. 2001;11:1975–1980.10.1016/S0960-9822(01)00594-2
- Havermann S, Humpf HU, Wätjen W. Baicalein modulates stress-resistance and life span in C. elegans via SKN-1 but not DAF-16. Fitoterapia. 2016;113:123–127.10.1016/j.fitote.2016.06.018
- Seo HW, Cheon SM, Lee MH, et al. Catalpol modulates lifespan via DAF-16/FOXO and SKN-1/Nrf2 activation in Caenorhabditis elegans. Evid Based Complement Alternat Med. 2015;2015:524878.
- Murphy CT, McCarroll SA, Bargmann CI, et al. Genes that act downstream of DAF-16 to influence the lifespan of Caenorhabditis elegans. Nature. 2003;424:277–283.10.1038/nature01789
- Lee J, Kwon G, Park J, et al. Brief Communication: SIR-2.1-dependent lifespan extension of Caenorhabditis elegans by oxyresveratrol and resveratrol. Exp Biol Med (Maywood), 2016;241:1757–1763.10.1177/1535370216650054
- Wang X, Wang X, Li L, et al. Lifespan extension in Caenorhabditis elegans by DMSO is dependent on sir-2.1 and daf-16. Biochem Biophys Res Commun. 2010;400:613–618.10.1016/j.bbrc.2010.08.113
- Mesquita A, Weinberger M, Silva A, et al. Caloric restriction or catalase inactivation extends yeast chronological lifespan by inducing H2O2 and superoxide dismutase activity. Proc Natl Acad Sci USA. 2010;107:15123–15128.10.1073/pnas.1004432107