Abstract
Obesity is associated with systemic oxidative stress and leads to insulin resistance. Phenethyl isothiocyanate (PEITC), a natural dietary isothiocyanate, has been shown to have beneficial effects in improving cellular defense activities against oxidative stress through activation of nuclear factor erythroid-2 related factor 2 (Nrf2) pathway. However, little evidence exists if the antioxidative activity has beneficial effects on glucose metabolism. Here, we tested the preventive potential of PEITC for impaired insulin-induced glucose uptake by oxidative stress in 3T3-L1 adipocytes. Treatment with PEITC increased the expression of antioxidative enzymes regulated by Nrf2 such as γ-glutamylcysteine-synthetase, heme oxygenase 1, NAD(P)H:quinone oxidoreductase 1 and glutathione S-transferase, and reduced oxidative stress induced by H2O2. Furthermore, PEITC restored impaired insulin-stimulated glucose uptake, translocation of glucose transporter 4 and insulin signaling by H2O2. These results indicate that PEITC protected insulin-regulated glucose metabolism impaired by oxidative stress through the antioxidative activity in 3T3-L1 adipocytes.
Graphical abstract
Phenethyl isothiocyanate (PEITC) protects from insulin resistance induced by oxidative stress in 3T3-L1 adipocytes.
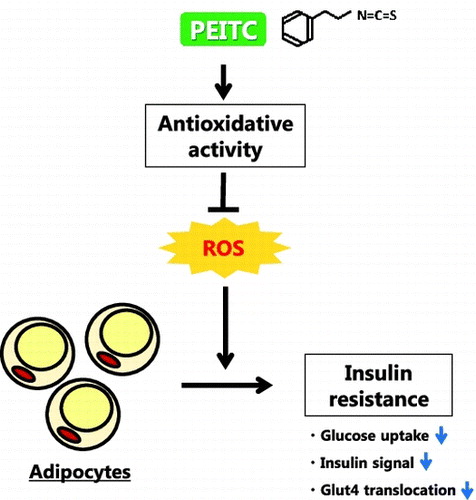
Obesity is associated with systemic oxidative stress.Citation1) The increase in reactive oxygen species (ROS) impairs glucose uptake in adipocytes,Citation2) which occurs through translocation of glucose transporter 4 (Glut4) to the plasma membrane upon insulin stimulation. Akt activation and its downstream signaling are necessary for the stimulation of Glut4 translocation by insulin.Citation3) Oxidative stress reduces insulin-dependent Akt activation and Glut4 translocation, thereby impairing glucose uptake.Citation2,4) Cyanidin 3-glucoside, a natural antioxidant, has been reported to provide protection from H2O2-induced insulin resistance in 3T3-L1 cells,Citation5) and suppression of ROS production by NADPH oxidase inhibitor improves glucose tolerance in obese mice.Citation1) Thus, increase in antioxidative potential can be a useful approach to protect insulin-regulated glucose metabolism from oxidative stress in adipocytes.
Isothiocyanates are plant bioactives found in cruciferous vegetables. Phenethyl isothiocyanate (PEITC) is an aromatic isothiocyanate and is found in watercress as its glucosinolate, gluconasturtiin.Citation6) PEITC and other isothiocyanates induce phase 2 and antioxidant enzymes via a nuclear factor erythroid-2-related factor (Nrf2) pathway,Citation7) thereby improving cellular defense activities against oxidative stress and detrimental substances. Nrf2 is a transcription factor belonging to Cap’n’collar-type basic leucine zipper proteins.Citation8) When exposed to oxidative/electrophilic stress, Nrf2 regulates phase 2 antioxidant enzyme genes having the antioxidant response element in the promoter region such as γ-glutamylcysteine-synthetase (γ-GCS), NAD(P)H:quinone oxidoreductase 1 (NQO1) and heme oxygenase-1 (HO-1).Citation9–11) In fact, PEITC activates Nrf2 pathway to attenuate lipopolysaccharide (LPS)-induced inflammation and to reduce oxidative stress using ultraviolet radiation.Citation12,13) Therefore, isothiocyanate possibly ameliorates impaired insulin signaling and glucose metabolism by oxidative stress through the antioxidative effect.
Among the isothiocyanates derived from dietary sources, sulforaphane, which is present in broccoli, cauliflower, and kale, has been extensively studied and shown to be beneficial for obesity and glucose metabolism. Sulforaphane attenuates obesity by inhibiting adipogenesis in high-fat diet-fed miceCitation14) and improves high-fat and high-sucrose diet-induced glucose intolerance in mice.Citation15) Furthermore, sulforaphane inhibits adipocyte differentiation by suppressing clonal expansion via cell cycle arrest in 3T3-L1 preadipocytes and induces lipolysis through hormone-sensitive lipase activation in 3T3-L1 adipocytes.Citation16,17) Therefore, the suppression of lipid accumulation may contribute to the ameliorative effect on glucose metabolism by isothiocyanates. However, little evidence exists if the antioxidative activity of isothiocyanate has beneficial effects on glucose metabolism and insulin signaling. Thus, the current study was undertaken to determine the preventive potential of PEITC, one of the major dietary isothiocyanates, for impaired insulin-induced glucose uptake by oxidative stress in 3T3-L1 adipocytes.
Materials and methods
Reagents
Dulbecco’s modified Eagles’ medium (DMEM with 25 mM glucose), bovine insulin, and 3-isobutyl-1-methylxanthine (IBMX) were purchased from Sigma (St. Louis, MO, USA). Dexamethasone (DEX) was obtained from Wako Pure Chemicals (Osaka, Japan). Bovine calf serum (CS) and fetal bovine serum (FBS) were purchased from Invitrogen (Grand Island, NY, USA) and BioWest (Nuaillé, France), respectively. PEITC was obtained from LKT laboratories (St. Paul, MN, USA). Anti-NQO1, anti-lamin B, anti-Akt, anti-p-Akt1/2/3 (Ser473), and anti-insulin receptor β (C-19) antibodies were purchased from Santa Cruz Biotechnology, Inc. (Santa Cruz, CA, USA). Anti-Nrf2 (D1Z9C), anti-Glut4 (1F8), anti-pAkt (Thr308, #9275) antibodies were obtained from Cell Signaling Technology (Danvers, MA, USA). Anti-HO-1 antibody was obtained from Enzo Life Sciences (Plymouth Meeting, PA, USA). Anti-rat glutathione S-transferase (GST) Ya antibody (GS09) was obtained from Oxford Biomedical Research (Oxford, MI, USA). Anti-γ-GCS(GCLC, ab190685) antibody was obtained from Abcam plc (Cambridge, UK). Anti-phospho insulin receptor (Y1162/Y1163) antibody was obtained from R&D systems (Minneapolis, MN, USA). Anti-β actin antibody (AC-15) was obtained from Novus Biologicals (Littleton, CO, USA). All other reagents were of analytical grade.
Cell culture and differentiation
Mouse 3T3-L1 preadipocytes (Human Science Research Resources Bank, Osaka, Japan) were cultured in DMEM containing 10% (v/v) bovine CS and antibiotics (100 U/mL penicillin and 100 μg/mL streptomycin) in a humidified atmosphere of 5% CO2 at 37 °C until confluent and then maintained in the same medium for an additional 2 days. Differentiation was induced at 2 days post-confluence by incubation in DMEM containing 10 μg/mL human recombinant insulin, 250 nM DEX, 0.5 mM IBMX, and 10% FBS for 3–4 days. The medium was replaced with DMEM containing 5 μg/mL insulin and 10% FBS and changed every 2 days. After 4 days, the cells were treated with or without 15 μM PEITC in DMEM containing 5 μg/mL insulin and 10% FBS for 48 h.
Oil Red O staining for lipids assessment
After incubation in DMEM containing 10 μg/mL insulin, 250 nM DEX, 0.5 mM IBMX, and 10% FBS for 3–4 days, the medium was replaced with DMEM containing 5 μg/mL insulin and 10% FBS with PEITC (0–20 μM) and was changed every 2 days. After 5 days of treatment with various PEITC concentrations, the same volume of 10% neutral formalin as the medium was added to the culture and the cells were mildly fixed for 20 min at room temperature. The formalin and medium were replaced with 10% neutral formalin. After another 1 h fixation, the cells were washed twice with PBS and stained with Oil Red O solution for 1 h at room temperature. The Oil Red O solution was prepared by mixing a stock solution (0.15 g of Oil Red O in 30 mL of isopropanol) with 20 mL of water, followed by filtration. After staining, the cells were washed twice with water and dried in air at room temperature. Oil Red O dye was eluted with isopropanol, and the extracted dye was quantified by measuring the absorbance at 490 nm to analyze the level of lipid content.
Determination of ROS production
The level of intracellular ROS was assessed by measuring the oxidation of 2′,7′-dichlorofluorescin diacetate (DCFH-DA) according to a previously described method with some modifications.Citation18) DCFH-DA diffuses through the cell membrane and is enzymatically hydrolyzed by intracellular esterase to non-fluorescent DCFH. Intracellular ROS oxidize DCFH to fluorescent 2′,7′-dichlorofluorescein (DCF), whose intensity of fluorescence is directly proportional to the levels of intracellular ROS. Briefly, the cells were labeled with 10 μM of DCFH-DA (Sigma) in DMEM containing 0.1% BSA in a 24-well culture plate (5 × 104 cells/well, Assist, Tokyo, Japan) for 45 min, followed by pretreatment with 15 μM of PEITC for 48 h. The cells were then exposed to H2O2 (0, 200 μM) in DMEM containing 0.1% BSA for 30 min. Fluorescence was measured at an excitation wavelength of 485 nm and an emission wavelength of 535 nm using an Infinite F200PRO plate reader (TECAN, Grödig, Austria).
Measurement of glucose uptake
We evaluated glucose uptake from the rate of glucose consumption in medium according to previously described method with some modifications.Citation19) The differentiated 3T3-L1 cells (5 × 104 cells/well in a 24-well plate) were cultured with 15 μM PEITC in DMEM containing 10% FBS and insulin. After 48 h, the cells were washed once with PBS and incubated with H2O2 (0–200 μM) in DMEM containing 0.1% BSA for 24 h. The cells were then incubated in phenol red and glucose-free DMEM (Sigma) containing 25 mM glucose with or without 100 nM insulin for 3 h. Glucose concentrations in the medium were determined using a glucose assay kit (Glucose CII-Test, Wako, Osaka, Japan), and the amounts of glucose uptake were calculated from the differences in glucose concentrations between before and after incubation.
Analysis of insulin signaling and western blot analysis
After 48 h treatment with 15 μM PEITC, the cells (2.5 × 105 cells/35 mm dish) were washed once with PBS and incubated with H2O2 (0, 200 μM) in DMEM containing 0.1% BSA for 24 h. The medium was replaced with fresh DMEM containing 0.1% BSA, and the cells were stimulated with 100 nM insulin for 10 min. The medium was removed and the cells were lysed in lysis buffer solution (lysis buffer A: 50 mM Tris-HCl (pH 7.4), 150 mM NaCl, 0.1% (w/v) SDS, 1% (v/v) Triton-X, 1% (w/v) deoxycholic acid, 0.5 mM Na3VO4, and 5 mM EDTA). Cell extracts were prepared by centrifugation for 10 min at 20,000 × g at 4 °C to remove the cell debris. Protein concentrations were measured as described previously.Citation20) Equal amounts of proteins were separated on SDS-PAGE gels and then transferred onto a PVDF membrane (Millipore, Bedford, MA, USA). The membrane was immersed in blocking buffer (5% BSA in 20 mM Tris-HCl (pH 7.6), 137 mM NaCl, 1 mM EDTA, 0.1% Tween 20 [TBS-T]) at room temperature for 1 h, and then incubated overnight at 4 °C with primary antibodies, followed by incubation with the appropriate secondary antibody, i.e., anti-rabbit, anti-goat, or anti-mouse IgG antibody conjugated with horseradish peroxidase at room temperature for 1 h. Immunoreactive signals were detected using ECL Western Blotting Substrate (GE Healthcare, Tokyo, Japan) and a luminoimage analyzer (ImageQuant LAS 4000, GE Healthcare), and then analyzed with a software (NIH Image).
Measurement of nuclear translocation of Nrf2
To assess the translocation of Nrf2 into the nucleus upon PEITC treatment, the amount of Nrf2 in the nuclear fraction was determined by Western blotting with anti-Nrf2 antibody as described previously.Citation21) After 48 h treatment with PEITC, the cells were harvested with a 10 mM HEPES-NaOH (pH 7.4) buffer containing 10 mM KCl, 2 mM MgCl2, 1 mM EDTA, and 0.5% NP-40. The homogenates were centrifuged at 20,000 × g for 10 min at 4 °C. The precipitate was resuspended in the lysis buffer A and centrifuged at 20,000 × g for 10 min at 4 °C. The supernatant was collected and used as the nuclear fraction.
Measurement of Glut4 translocation
To assess the translocation of Glut4 to the cell surface, the amount of Glut4 in the plasma membrane fraction was determined by Western blotting with anti-Glut4 antibody, according to the method previously described with some modifications.Citation22) Briefly, 3T3-L1 cells were incubated with or without 100 nm insulin for 15 min and then harvested with a lysis buffer (50 mM Tris-HCl (pH 7.4), 150 mM NaCl, 0.5 mM Na3VO4, 5 mM EDTA, and 0.1% NP-40). The homogenates were centrifuged at 20,000 × g for 10 min at 4 °C. The precipitate was lysed using a lysis buffer (50 mM Tris-HCl (pH 7.4), 150 mM NaCl, 0.5 mM Na3VO4, 5 mM EDTA, and 1.0% NP-40) and centrifuged at 20,000 × g for 10 min at 4 °C. The supernatant was collected and used as the plasma membrane fraction.
Statistical analysis
The data are expressed as the mean ± SE. For comparison of groups with comparable variances, one-way ANOVA and a Tukey’s post hoc test were carried out. The p values below 0.05 were considered significant.
Results
PEITC did not affect the level of lipid accumulation and stimulated the expression of detoxification and antioxidant proteins in 3T3-L1 cells
We first tested the effect of PEITC on lipid accumulation in 3T3-L1 adipocytes. As shown in Fig. , the lipid accumulation did not show any difference by treatment with PEITC until the concentration of 20 μM at the later stage of the culture, a stage after clonal expansion induced by treatment with differentiation inducer mixture of IBMX, DEX and insulin.
Fig. 1. Effect of PEITC on lipid accumulation.
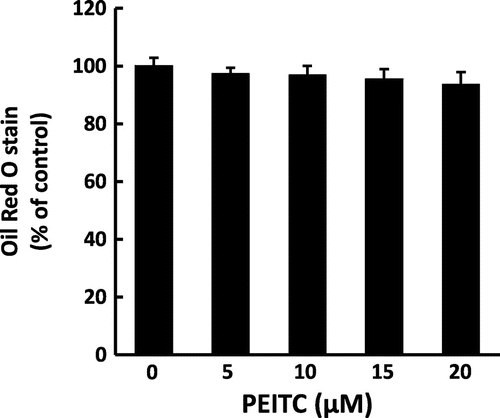
It has been reported that isothiocyanates induce the expression of detoxification and antioxidant genes including γ-GCS, NQO1, HO-1, and GST through Nrf2 activation.Citation23,24) We examined the effect of PEITC on the translocation of Nrf2 into the nucleus and on the induction of antioxidative enzymes regulated by Nrf2 in 3T3-L1 adipocytes. As shown in Fig. , the amount of Nrf2 in the nuclear fraction was increased in a dose-dependent manner of PEITC treatment. Concomitantly, the amount of γ-GCS, HO-1, NQO1, and GST proteins was significantly increased at the concentration of 15 μM and more of PEITC (Fig. ). Isothiocyanates including PEITC are reported to have a cytotoxic effect in cancer cells.Citation25,26) Thus, we treated with 15 μM of PEITC in further studies, although no obvious changes were observed in 3T3-L1 cells treated even with 20 μM of PEITC.
Fig. 2. Effect of PEITC on Nrf2 nuclear translocation.
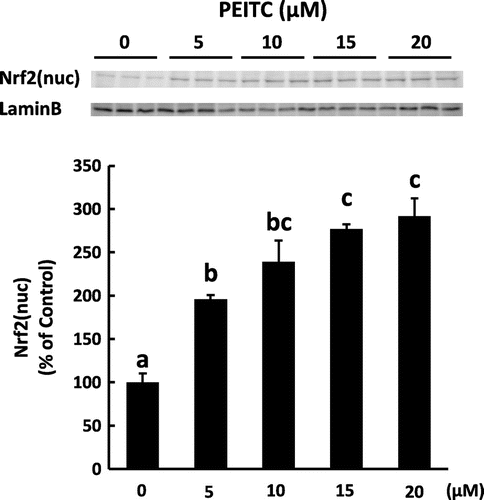
Fig. 3. Effect of PEITC on the protein level of Nrf2-regulating genes.
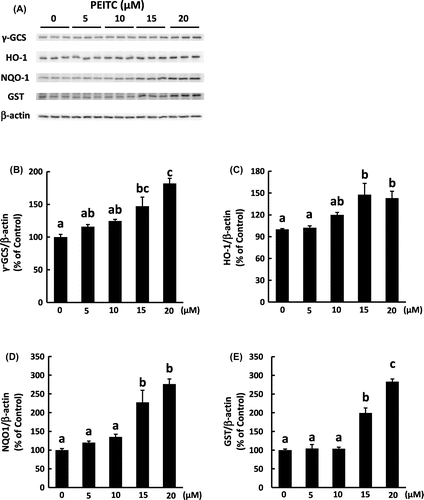
PEITC reduced oxidative stress by H2O2 treatment
To investigate the protective effect of PEITC against oxidative stress, we next assessed the level of DCFH-DA oxidation upon treatment with H2O2 in 3T3-L1 cells pretreated with PEITC. As shown in Fig. , pretreatment with 15 μM PEITC clearly reduced the oxidative stress induced by H2O2 in 3T3-L1 cells.
Fig. 4. Effect of PEITC on oxidative stress in H2O2-exposed 3T3-L1 cells.
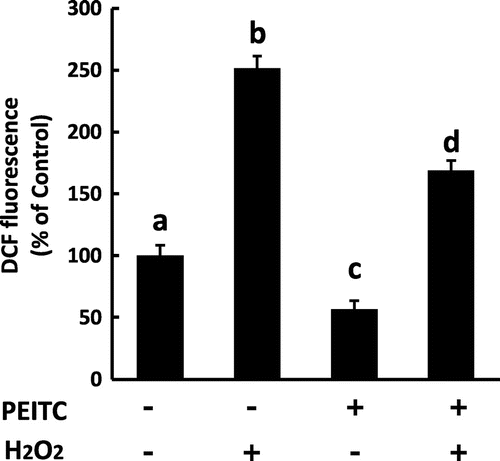
PEITC protected against downregulation of glucose uptake and insulin-stimulated translocation of Glut4 in H2O2-exposed 3T3-L1 adipocytes
Insulin increases cell surface Glut4, and thereby, induces glucose uptake in 3T3-L1 adipocytes. Thus, we next examined the protective effect of PEITC on glucose uptake and the translocation of Glut4 to the plasma membrane by insulin in H2O2-exposed cells. As shown in Fig. (A), treatment with H2O2 decreased insulin-induced glucose uptake, which was restored by pretreatment with PEITC. Consistent with the glucose uptake, insulin-induced translocation of Glut4 was decreased with H2O2 and the impairment of insulin-induced Glut4 translocation by H2O2 was recovered by pretreatment with PEITC (Fig. (B)). Further, we analyzed whether the treatment with PEITC and H2O2 affects the total cellular protein level of Glut4. As shown in Fig. (A), pretreatment with PEITC slightly increased the total cellular amount of Glut4 protein, while that with H2O2 did not affect it.
Fig. 5. Effect of PEITC on insulin-dependent glucose uptake.
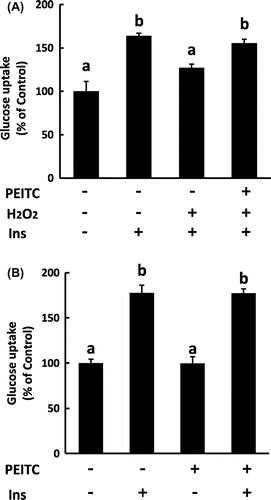
Fig. 6. Effect of PEITC on the amount of Glut4 protein and insulin-dependent translocation of Glut4 protein to plasma membrane.
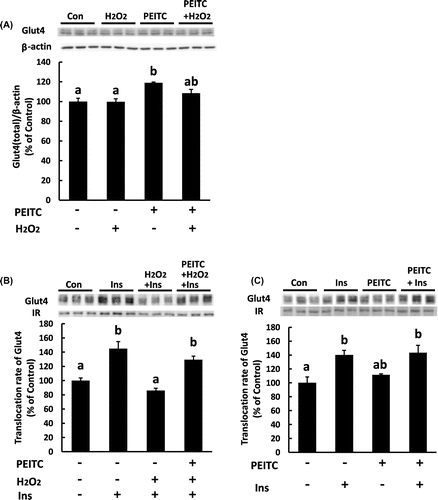
To investigate the possibility that the increased level of Glut4 protein by PEITC contributes to restoration from the impairment of insulin-induced glucose uptake and translocation of Glut4 by H2O2, we next examined the effect of PEITC on glucose uptake and Glut4 translocation in 3T3-L1 cells without H2O2 treatment. The differentiated cells were incubated with 15 μM PEITC for 48 h, and then the medium was replaced with a fresh DMEM + 0.1% BSA. The cells were stimulated with 100 nM insulin for 3 h for glucose uptake assay and for 15 min in order to analyze translocation of Glut4. As shown in Figs. (B) and (C), PEITC alone did not have any effect on glucose uptake and the amount of Glut4 in the membrane fraction. Furthermore, any synergic effect of PEITC on insulin-dependent glucose uptake and Glut4 translocation was not observed. These results suggest that the restoration of insulin-induced glucose uptake by PEITC in the cell with H2O2 treatment is not due to upregulation of the protein level of Glut4, but is likely due to recovering from the decreased rate of translocation of Glut4.
Pretreatment with PEITC restored impaired insulin signaling by H2O2-inducing oxidative stress
Activation of Akt is necessary for insulin-stimulated translocation of Glut4Citation3,27) and glucose uptake,Citation28,29) which is impaired by oxidative stress.Citation2) Thus, we examined changes in insulin signaling to investigate the molecular mechanism underlying the protective effect of PEITC on impaired insulin-induced glucose uptake and Glut4 translocation in H2O2-exposed 3T3-L1 cells. As shown in Fig. (left panels), treatment with H2O2 impaired tyrosine phosphorylation in the insulin receptor and Akt phosphorylation in response to insulin, which was restored by pretreatment with PEITC. Then, we examined the effect of PEITC on these signaling molecules of the insulin signal under the condition without H2O2 treatment. As a result in Fig. (right panels), PEITC alone did not induce activation of these insulin signal molecules and did not have any effect on insulin-induced activation at the level of insulin receptor. Activation of Akt induced by insulin was enhanced by pretreatment with PEITC. However, the effect on Akt activation was not observed in glucose uptake (Fig. (B)). These findings suggest that PEITC reduced oxidative stress through antioxidative enzymes and preserved insulin signaling from oxidative stress.
Fig. 7. Effect of PEITC on insulin-dependent signaling.
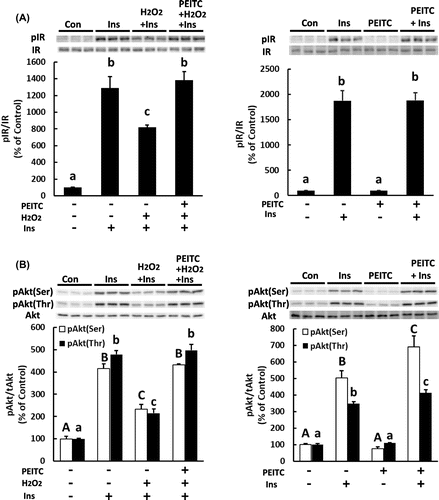
Discussion
ROS are generated from the cellular metabolic reaction. Oxidative stress is increased in obese subjects and the production of ROS increases in adipose tissue of obese mice. Increased oxidative stress affects the adipose tissue function, and thereby, leads to insulin resistance and impaired glucose uptake.Citation1,2,30) Numerous studies have shown that PEITC, one of the dietary isothiocyanates, induces cellular defense activity through the activation of Nrf2 pathway and exerts the cancer chemopreventive effect by upregulation of protective activity against oxidative stress and reactive metabolites of carcinogens.Citation7,31) However, little information is available about the ameliorative effect of PEITC on the metabolic dysregulation induced by oxidative stress. The current study has examined whether PEITC has preventive activity toward impaired insulin response and glucose uptake in 3T3-L1 adipocytes treated with H2O2.
To examine whether PEITC treatment induces the expression of antioxidant enzymes downstream of Nrf2 pathway, we observed the translocation of Nrf2 into the nucleus and the expression of antioxidative enzymes upon treatment with PEITC. We found that the amount of Nrf2 protein increased in the nuclear fraction when the cells were treated with PEITC (Fig. ). This result is consistent with those of previous studies using another isothiocyanate. Sulforaphane induces the accumulation of Nrf2 by inhibiting its degradation,Citation32,33) and sulforaphane and other isothiocyanates including PEITC increase the expression of antioxidative enzymes, while antioxidative effect of PEITC decreases in Nrf2 (–/–) macrophage cells.Citation7,12) In this study, PEITC induced several antioxidant enzymes downstream of Nrf2 pathway and reduced oxidative stress in 3T3-L1 adipocytes (Figs. and ). Thus antioxidative effect of PEITC in 3T3-L1 adipocytes treated with H2O2 in the present study would be mediated by increased expression of antioxidative enzymes downstream of Nrf2 pathway. In the present study, it is not defined whether induction of these antioxidative enzymes by PEITC is directly regulated by Nrf2 pathway in 3T3-L1 cells. Further studies are needed to clarify the precise mechanism.
Several studies support the preventive effect of the downstream enzymes of Nrf2 pathway against oxidative stress. PEITC induced enzymes related to glutathione metabolism, GST, and γ-GCS (Fig. ). GST catalyzes the conjugation of glutathione with electrophilic substrates, and γ-GCS is a limiting enzyme in the synthesis of glutathione, thereby increasing the redox capacity against oxidative stress. Some isothiocyanates are shown to protect against oxidative stress by upregulation of γ-GCS and the increased level of glutathione.Citation24) Elevated expression of NQO1 by sulforaphane is also shown to exert cytoprotective activity against cisplatin-induced oxidative stress.Citation34) Furthermore, in 3T3-L1 cells, expression of NQO1 decreases lipid accumulation which is associated with oxidative stress.Citation1,35) Thus, NQO1 might be involved in the reduction of oxidative stress by PEITC. Several reports support that HO-1 is another potential protein for the protective effect of PEITC against oxidative stress.Citation36–38) Omega-3 polyunsaturated fatty acids, eicosapentaenoic acid, and docosahexaenoic acid, reduce H2O2-induced oxidative stress through HO-1 induction in 3T3-L1 adipocytes,Citation36) and HO-1 protects human melanocytes from H2O2-induced oxidative stress.Citation38) In addition to the antioxidant effect of HO-1, modulation of insulin sensitivity by HO-1 might be involved in the effect of PEITC. It is reported that the administration of the HO-1 inducer, cobalt protoporphyrin, reduces adiposity and improves insulin resistance in obese diabetic animals, although the physiologic role of HO-1 in adipocytes has not been established.Citation39,40) Taken together, PEITC would reduce oxidative stress and improve insulin resistance in adipocytes exposed to H2O2 by induction of the above antioxidative enzymes, although further studies are needed to clarify the precise role of these enzymes in the effect of PEITC.
Besides the mechanism through the upregulation of antioxidative enzymes in the antioxidative effect of PEITC, possible mechanisms may occur through lipid metabolism and mitochondrial biogenesis. It is reported that lipid accumulation parallels ROS production in 3T3-L1 adipocytes,Citation1) and the chronic activation of Nrf2 by administering agonists, CDDO-ImCitation41) or oltipraz,Citation42) prevents high-fat diet-induced obesity in mice. Therefore, treatment with PEITC may reduce oxidative stress through suppression of lipid accumulation. In fact, Zhang et al. demonstrated that sulforaphane increased mitochondrial biogenesis and activated glucose aerobic oxidation pathway, thereby resulting in reduction of lipid accumulation.Citation33) However, we did not observe the reduction of lipid accumulation by treatment with PEITC during the latter stage of differentiation (Fig. ). This result is consistent with that of a previous report that treatment of sulforaphane inhibits the accumulation of triglyceride in adipocytes during the early stage of the differentiation (a stage of clonal expansion treated with IBMX, DEX, and insulin), but does not have any effect on lipid accumulation during the late stage after clonal expansion.Citation16) Taken together, it is unlikely that the reduction of oxidative stress by PEITC in this study is relevant to the effect on lipid metabolism by PEITC.
In conclusion, we demonstrated that PEITC can induce the expression of antioxidative enzymes in 3T3-L1 adipocytes and exerts protective effect against downregulation of insulin-induced glucose uptake by H2O2 treatment. PEITC is found in ordinary food materials, watercress, radish, and turnip,Citation6,31) which may act as potential tools for the prevention and treatment of dysregulation of glucose metabolism associated with oxidative stress, although its efficacy in vivo needs be proved by further study.
Author contributions
Y.I. designed the study, M.N. and Y.I. performed the experiments, M.N., Y.I., and T.N. analyzed the data and discussed results, M.N. and Y.I. wrote the manuscript. All authors read and approved the manuscript.
Disclosure statement
No potential conflict of interest was reported by the authors.
Funding
This work was supported by the Grant-in-Aid for Scientific Research from Japan Society for the Promotion of Science [grant number 22580125], [grant number 25450161].
References
- Furukawa S, Fujita T, Shimabukuro M, et al. Increased oxidative stress in obesity and its impact on metabolic syndrome. J Clin Invest. 2004;114:1752–1761.10.1172/JCI21625
- Tirosh A, Potashnik R, Bashan N, et al. Oxidative stress disrupts insulin-induced cellular redistribution of insulin receptor substrates-1 and phosphatidylinositol 3-kinase in 3T3-L1 adipocytes. J Biol Chem. 1999;274:10595–10602.10.1074/jbc.274.15.10595
- Brewer PD, Romenskaia I, Kanow MA, et al. Loss of AS160 Akt substrate causes glut4 protein to accumulate in compartments that are primed for fusion in basal adipocytes. J Biol Chem. 2011;286:26287–26297.10.1074/jbc.M111.253880
- Shibata M, Hakuno F, Yamanaka D, et al. Paraquat-induced oxidative stress represses phosphatidylinositol 3-kinase activities leading to impaired glucose uptake in 3T3-L1 adipocytes. J Biol Chem. 2010;285:20915–20925.10.1074/jbc.M110.126482
- Guo H, Ling W, Wang Q, et al. Cyanidin 3-glucoside protects 3T3-L1 adipocytes against H2O2- or TNF-α-induced insulin resistance by inhibiting c-Jun NH2-terminal kinase activation. Biochem Pharmacol. 2008;75:1393–1401.10.1016/j.bcp.2007.11.016
- Palniswamy UR, McAvoy RJ, Bible BB, et al. Ontogenic variations of ascorbic acid and phenethyl isothiocyanate concentrations in watercress (Nasturtium officinate R.Br.) leaves. J Agric Food Chem. 2003;51:5504–5509.10.1021/jf034268w
- Ernst IMA, Wagner AE, Schuemann C, et al. Allyl-, butyl- and phenylethyl-isothiocyanate activate Nrf2 in cultured fibroblasts. Pharmacol Res. 2011;63:233–240.10.1016/j.phrs.2010.11.005
- Motohashi H, O’Conner T, Katsuka F, et al. Integration and diversity of the regulatory network composed of Maf and CNC families of transcription factors. Gene. 2002;294:1–12.10.1016/S0378-1119(02)00788-6
- Wild AC, Moinova HR, Mulcahy RT. Regulation of γ-glutamylcysteine synthetase subunit gene expression by the transcription factor Nrf2. J Biol Chem. 1999;274:33627–33636.10.1074/jbc.274.47.33627
- Favreau LV, Pickett CB. Transcriptional regulation of the rat NAD(P)H:quinone reductase gene. Identification of regulatory elements controlling basal level expression and inducible expression by planar aromatic compounds and phenolic antioxidants. J Biol Chem. 1991;266:4556–4561.
- Prestera T, Talalay P, Alam J, et al. Parallel induction of heme oxygenase-1 and chemoprotective phase 2 enzymes by electrophiles and antioxidants: regulation by upstream antioxidant responsive elements (ARE). Mol Med. 1995;1:827–837.
- Boyanapalli SS, Paredes-Gonzalez X, Fuentes F, et al. Nrf2 knockout attenuates the anti-inflammatory effects of phenethyl isothiocyanate and curcumin. Chem Res Toxicol. 2014;27:2036–2043.10.1021/tx500234 h
- Kleszczyński K, Ernst IM, Wagner AE, et al. Sulforaphane and phenethyl isothiocyanate protect human skin against UVR-induced oxidative stress and apoptosis: role of Nrf2-dependent gene expression and antioxidant enzymes. Pharmacol Res. 2013;78:28–40.10.1016/j.phrs.2013.09.009
- Choi KM, Lee YS, Kim W, et al. Sulforaphane attenuates obesity by inhibiting adipogenesis and activating the AMPK pathway in obese mice. J Nutr Biochem. 2014;25:201–207.10.1016/j.jnutbio.2013.10.007
- Shawky N, Pichavaram P, Shehatou GSG, et al. Sulforaphane improves dysregulated metabolic profile and inhibits leptin-induced VSMC proliferation: implications toward suppression of neointima formation after arterial injury in western diet-fed obese mice. J Nutr Biochem. 2016;32:73–84.10.1016/j.jnutbio.2016.01.009
- Choi KM, Lee YS, Sin DM, et al. Sulforaphane inhibits mitotic clonal expansion during adipogenesis through cell cycle arrest. Obesity. 2012;20:1365–1371.10.1038/oby.2011.388
- Lee JH, Moon MH, Jeong JK, et al. Sulforaphane induced adipolysis via hormone sensitive lipase activation, regulated by AMPK signaling pathway. Biochem Biophys Res Commun. 2012;426:492–497.10.1016/j.bbrc.2012.08.107
- Park SY, Je JY, Ahn CB. Phenolic composition and hepatoprotective activities of Allium hookeri against hydrogen-peroxide-induced oxidative stress in cultured hepatocytes. J Food Biochem. 2016;40:284–293.10.1111/jfbc.2016.40.issue-3
- Son MJ, Miura Y, Yagasaki K. Mechanisms for antidiabetic effect of gingerol in cultured cells and obese diabetic model mice. Cytotechnology. 2015;67:641–652.10.1007/s10616-014-9730-3
- Ito Y, Oumi S, Nagasawa T, et al. Oxidative stress induces phosphoenolpyruvate carboxykinase expression in H4IIE cells. Biosci Biotechnol Biochem. 2006;70:2191–2198.10.1271/bbb.60135
- Lee HS, Li L, Kim HK, et al. The protective effects of Curcuma longa Linn. Extract on carbon tetrachloride-induced hepatotoxicity in rats via upregulation of Nrf2. J Microbiol Biotechnol. 2010;20:1331–1339.10.4014/jmb
- Nishiumi S, Ashida H. Rapid preparation of a plasma membrane fraction from adipocytes and muscle cells: application to detection of translocated glucose transporter 4 on the plasma membrane. Biosci Biotechnol Biochem. 2007;71:2343–2346.10.1271/bbb.70342
- Klaassen CD, Reisman SA. Nrf2 the rescue: effects of the antioxidative/electrophilic response on the liver. Toxicol Appl Pharmacol. 2010;244:57–65.10.1016/j.taap.2010.01.013
- Mizuno K, Kume T, Muto C, et al. Glutathione biosynthesis via activation of the nuclear factor e2-related factor 2 (Nrf2) – antioxidant-response element (ARE) pathway is essential for neuroprotective effects of sulforaphane and 6-(methylsulfinyl) hexyl isothiocyanate. J Pharmacol Sci. 2011;115:320–328.10.1254/jphs.10257FP
- Satyam KS, Swamy N, Dizon DS, et al. Phenethyl isothiocyanate (PEITC) inhibits growth of ovarian cancer cells by inducing apoptosis: role of caspase and MAPK activation. Gynecol Oncol. 2006;103:261–270.10.1016/j.ygyno.2006.03.002
- Fimognari C, Lenzi M, Hrelia P. Apoptosis induction by sulfur-containing compounds in malignant and nonmalignant human cells. Environ Mol Mutagen. 2009;50:171–189.10.1002/em.v50:3
- Chang L, Chiang SH, Saltiel AR. Insulin signaling and the regulation of glucose transport. Mol Med. 2004;10:65–71.
- Karnieli E, Zarnowski MJ, Hissin PJ, et al. Insulin-stimulated translocation of glucose transport systems in the isolated rat adipose cell. J Biol Chem. 1981;256:4772–4777.
- Brewer PD, Habtemichael EN, Romenskaia I, et al. Insulin-regulated Glut4 translocation: membrane protein trafficking with six distinctive steps. J Biol Chem. 2014;289:17280–17298.10.1074/jbc.M114.555714
- Casteilla L, Rigoulet M, Pénicaud L. Mitochondrial ROS metabolism: modulation by uncoupling proteins. IUBMB Life. 2001;52:181–188.10.1080/15216540152845984
- Fuentes F, Paredes-Gonzalez X, Kong AT. Dietary glucosinolates sulforaphane, phenethyl isothiocyanate, indole-3-carbinol/3,3′-diindolylmethane: Anti-oxidative stress/inflammation, Nrf2, epigenetics/epigenomics and in vivo cancer chemopreventive efficacy. Curr Pharmacol Rep. 2015;1:179–196.10.1007/s40495-015-0017-y
- McMahon M, Itoh K, Yamamoto M, et al. Keap1-dependent proteasomal degradation of transcription factor Nrf2 contributes to the negative regulation of antioxidant response element-driven gene expression. J Biol Chem. 2003;278:21592–21600.10.1074/jbc.M300931200
- Zhang HQ, Chen YC, Wang AS, et al. Sulforaphane induces adipocyte browning and promotes glucose and lipid utilization. Mol Nutr Food Res. 2016;60:2185–2197.10.1002/mnfr.v60.10
- Guerrero-Beltrán CE, Calderón-Oliver M, Martínez-Abundis E, et al. Protective effect of sulforaphane against cisplatin-induced mitochondrial alterations and impairment in the activity of NAD(P)H: quinone oxidoreductase 1 and γ glutamyl cysteine ligase: Studies in mitochondria isolated from rat kidney and in LLC-PK1 cells. Toxicol Lett. 2010;199:80–92.10.1016/j.toxlet.2010.08.009
- Vomhof-DeKrey EE, Picklo MJ. NAD(P)H:quinone oxidoreductase 1 activity reduces hypertrophy in 3T3-L1 adipocytes. Free Radic Biol Med. 2012;53:690–700.10.1016/j.freeradbiomed.2012.05.047
- Kusunoki C, Yang L, Yoshizaki T, et al. Omega-3 polyunsaturated fatty acid has an anti-oxidant effect via the Nrf-2/HO-1 pathway in 3T3-L1 adipocytes. Biochem Biophys Res Commun. 2013;430:225–230.10.1016/j.bbrc.2012.10.115
- Clark JE, Foresti R, Green CJ, et al. Dynamics of haem oxygenase-1 expression and bilirubin production in cellular protection against oxidative stress. Biochem J. 2000;348:615–619.10.1042/bj3480615
- Jian Z, Li K, Liu L, et al. Heme oxygenase-1 protects human melanocytes from H2O2-induced oxidative stress via the Nrf2-ARE pathway. J Invest Dematol. 2011;131:1420–1427.10.1038/jid.2011.56
- Li M, Kim DH, Tsenovoy PL, et al. Treatment of obese diabetic mice with a heme oxygenase inducer reduces visceral and subcutaneous adiposity, increases adiponectin levels, and improves insulin sensitivity and glucose tolerance. Diabetes. 2008;57:1526–1535.10.2337/db07-1764
- Nicolai A, Li M, Kim DH, et al. Heme oxygenase-1 induction remodels adipose tissue and improves insulin sensitivity in obesity-induced diabetic rats. Hypertension. 2009;53:508–515.10.1161/HYPERTENSIONAHA.108.124701
- Shin S, Wakabayashi J, Yates MS, et al. Role of Nrf2 in prevention of high-fat diet-induced obesity by synthetic triterpenoid CDDO-imidazolide. Eur J Pharmacol. 2009;620:138–144.10.1016/j.ejphar.2009.08.022
- Yu Z, Shao W, Chiang Y, et al. Oltipraz upregulates the nuclear respiratory 2 alpha subunit (NRF2) antioxidant system and prevents insulin resistance and obesity induced by a high-fat diet in C57BL/6J mice. Diabetologia. 2011;54:922–934.10.1007/s00125-010-2001-8