Abstract
In Corynebacterium glutamicum, the activity of the 2-oxoglutarate dehydrogenase (ODH) complex is negatively regulated by the unphosphorylated form of OdhI protein, which is critical for L-glutamate overproduction. We examined the potential impact of protein acylation at lysine (K)-132 of OdhI in C. glutamicum ATCC13032. The K132E succinylation-mimic mutation reduced the ability of OdhI to bind OdhA, the catalytic subunit of the ODH complex, which reduced the inhibition of ODH activity. In vitro succinylation of OdhI protein also reduced the ability to inhibit ODH, and the K132R mutation blocked the effect. These results suggest that succinylation at K132 may attenuate the OdhI function. Consistent with these results, the C. glutamicum mutant strain with OdhI-K132E showed decreased L-glutamate production. Our results indicated that not only phosphorylation but also succinylation of OdhI protein may regulate L-glutamate production in C. glutamicum.
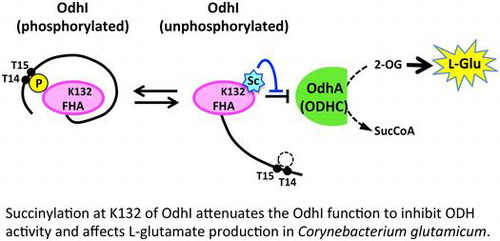
Succinylation at K132 of OdhI attenuates the OdhI function to inhibit ODH activity and affects L-glutamate production in Corynebacterium glutamicum.
Protein Nε-acylation targets lysine residues and is a widely distributed post-translational modification in the three domains of life. Since protein acylation is regulated by metabolites, such as acyl-CoA and NAD+, this modification could play a role in the regulation of protein function in response to nutrient signals and/or metabolism.Citation1–4) Corynebacterium glutamicum is an excellent glutamate producer that is utilized for industrial production of amino acids including L-glutamate and L-lysine.Citation5) We reported that the acetylation and succinylation profiles of many enzymes are drastically changed in response to L-glutamate overproduction in C. glutamicum, including enzymes involved in central metabolic pathways.Citation6) We also showed that phosphoenolpyruvate carboxylase, which is an anaplerotic enzyme catalyzing the supply of oxaloacetate and which is essential for L-glutamate production, is regulated by protein acetylation.Citation7) However, the functions of acylation of most other proteins remain to be elucidated.
The carbon flux change at the branch point of 2-oxoglutarate is pivotal for L-glutamate overproduction. The carbon flux redistribution has been explained by the decrease in the activity of the 2-oxoglutarate dehydrogenase (ODH) complex, which is a well-known phenomenon during L-glutamate overproduction.Citation8–10) The decrease in ODH activity decreases the flux of the citrate cycle and increases the flux toward L-glutamate synthesis via glutamate dehydrogenase.Citation10) The ODH complex is composed of three subunits: E1o (α-ketoglutarate decarboxylase, OdhA or Kgd [NCgl1084 in KEGG]), E2 (dihydrolipoamide acetyl/succinyl-transferase, AceF [NCgl2126 in KEGG]), and E3 (dihydrolipoamide dehydrogenase, Lpd [NCgl0355 in KEGG]). The E2 subunit is shared with the pyruvate dehydrogenase complex in C. glutamicum.Citation11) OdhI regulates ODH activity via its phosphorylation status.Citation11) OdhI is composed of an N-terminal domain containing two phosphorylation sites (Thr14 and Thr15) and a C-terminal forkhead-associated (FHA) domainCitation12) (Fig. (A)). The FHA domain in the unphosphorylated form is bound to the E1 (OdhA) subunit of the ODH complex to inhibit the ODH activity.Citation13,14) Phosphorylation of Thr14 and Thr15 induces a conformational change of OdhI to prevent it from inhibiting ODH activity.Citation15) The phosphorylation status of OdhI is regulated by the serine/threonine kinases PknG, PknA, PknB, and PknL and the serine/threonine phosphatase Ppp.Citation16) Deletion and overexpression of the odhI gene abolishes and increases L-glutamate production, respectively, indicating that OdhI is a critical factor in glutamate production.Citation17,18)
Fig. 1. Schematic positions of acetylation and succinylation sites in OdhI. (A) The primary structure of OdhI. Phosphorylation, acetylation, and succinylation sites are denoted as “P”, “Ac”, and “Sc”, respectively. (B) A structural model of the OdhA and OdhI complex.Citation6) The modeled structure was constructed based on the structure of the Mycobacterium smegmatis Kgd/GarA complex (PDB ID 2XT9). OdhA is indicated in green. The FHA domain of OdhI is indicated in magenta.
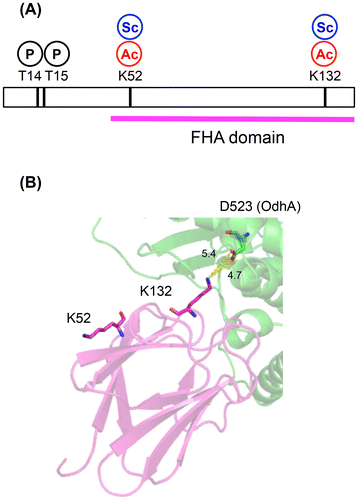
Fig. 2. The binding ability of OdhI to OdhA. (A) Purified His-tagged OdhA (0.5 μM) and Strep-tagged OdhI (2.5 μM) proteins were incubated in 0.5 mL of TESGN buffer at 30 °C for 30 min and then subjected to affinity purification using a Ni-NTA resin. Eluted samples were separated by 15% SDS-PAGE followed by Coomassie Brilliant Blue staining (upper panel). “ + ” and “−” indicate incubation of Strep-tagged OdhI with and without His-tagged OdhA before Ni-NTA purification, respectively. Protein bands of OdhA and OdhI were analyzed by densitometry. After normalizing of the amount of OdhI to that of OdhA, the relative binding of OdhI to the wild type (WT) variant was determined (lower panel). Data are the mean and standard deviation of two analytical replicates. (B) Pull-down assay was performed using purified His-tagged OdhA (0.5 μM) and various concentrations of Strep-tagged OdhI (1, 4, and 8 μM). After normalizing the amount of OdhI to that of OdhA, the relative binding of OdhI to OdhA was normalized to the 1 μM point of the wild type, and is shown here. Open circles, closed circles, closed triangles, and closed squares represent the wild-type OdhI, OdhI-K132R, OdhI-K132Q, and OdhI-K132E, respectively.
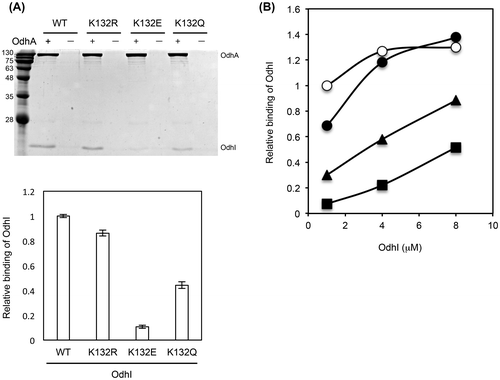
Fig. 3. ODH activity assay with recombinant OdhI proteins. Cell-free extract of C. glutamicum KSY22 (∆odhI) (1 mg) was preincubated with purified Strep-tagged OdhI protein (15.4 μg) at 30 °C for 10 min and subjected to the ODH activity assay. Data are the mean and standard deviation of three independent assays. The difference between the ODH activities with and without OdhI represents the inhibitory effect of OdhI on ODH activity. The inhibitory activity relative to the wild type (WT) variant is shown.
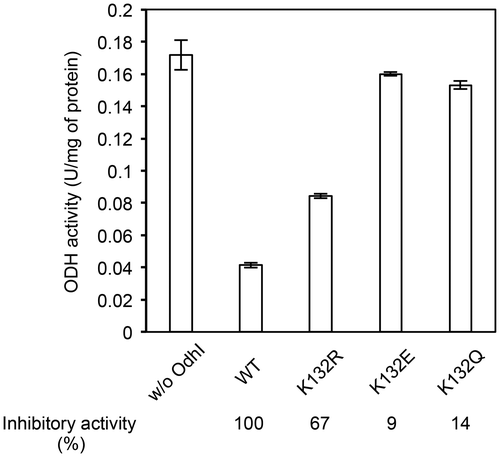
Fig. 4. ODH activity assay with in vitro succinylated OdhI protein. (A) Western blot and MS analyses of in vitro succinylated OdhI protein. Strep-tagged OdhI protein was incubated with 0, 0.1, 1, and 10 mM succinyl-CoA at 30 °C for 30 min. An aliquot containing 0.5 μg protein was subjected to 15% SDS-PAGE followed by western blot analysis using anti-succinyllysine or anti-OdhI antibody. Relative amount of K24-, K52-, and K132-succinylated peptides in each condition is shown below. K24Succ, 20-ADLLKSuccEMESSTGTAPASTGAENLPAGSALLVVK-52; K52Succ, 25-EMESSTGTAPASTGAENLPAGSALLVVKSuccR-53; and K132Succ, 118-NAQVMoxQTGDEIQIGKSuccFR-134. Semi-quantification of the succinylated peptides was based on the ratio of the peak areas of the peptides, after normalization with the amount of OdhI. (B) MS/MS ion spectrum of the K132-succinylated peptide (118-NAQVMoxQTGDEIQIGKSuccFR-134). (C) Succinylated Strep-tagged OdhI proteins (2 μg) with 0, 0.1, 1, and 10 mM succinyl-CoA were preincubated with cell-free extract of KSY22 (∆odhI) (0.4 mg) at 30 °C for 10 min and subjected to the ODH activity assay. Data are the mean and standard deviation of three independent assays. The ratio of OdhI to cell free extract was lower than that given in Figure , and this is used to detect the effect of succinylation on OdhI.
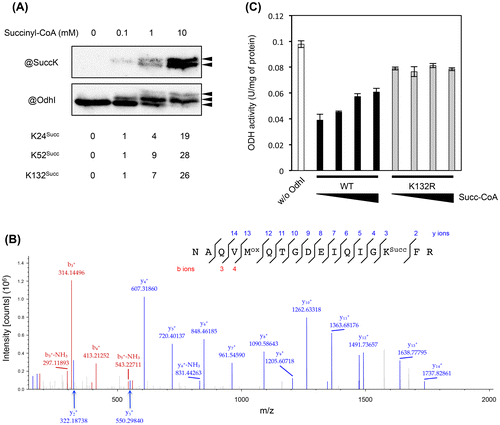
Fig. 5. Effect of succinylation on the phosphorylation-dependent OdhI function. (A) The three-dimensional structure of the phosphorylated form of OdhI (PDB ID 2KB3). The FHA domain is shown in magenta. Thr14 phosphorylation and Lys132 succinylation sites are shown. Lys132 is predicted to interact electrostatically with Glu25. Possible effect of K132-succinylation on phosphorylation-dependent conformational change of OdhI is schematically represented. (B) Succinylated Strep-tagged OdhI protein (with 10 mM succinyl-CoA) was phosphorylated by His-tagged PknG in vitro and subjected to the ODH activity assay. The assay condition was the same as described in Fig. 4. Data are the mean and standard deviation of three independent assays. Phosphorylation of OdhI was confirmed by Phos-tag SDS-PAGE.
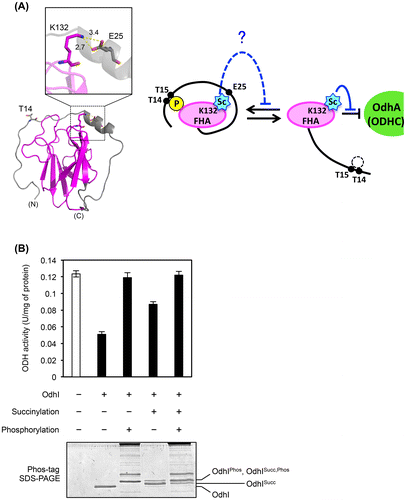
Fig. 6. Glutamate production of the OdhI-K132 mutant strains. C. glutamicum AA19 (OdhI_WT), KSY22 (ΔodhI), AA14 (OdhI_K132R), AA16 (OdhI_K132E), and AA18 (OdhI_K132Q) strains were cultivated in glutamate-production medium with and without Tween 40-triggered induction for 18 h. Diamonds and bars represent the OD660 and L-glutamate production, respectively. Reproducible results were obtained from experiments repeated three times, and a representative dataset is shown. A Student’s t test was performed to indicate statistical significance (*, p < 0.01; **, p < 0.05). Data of glutamate production are expressed as the mean of three independent cultures and its standard deviation.
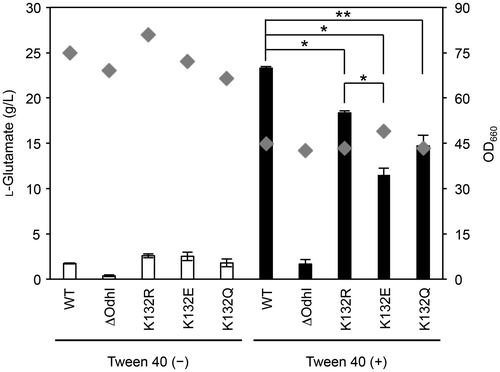
Our previous acylome study identified two lysine residues, Lys52 and Lys132, in the FHA domain of OdhI as sites of acetylation and succinylationCitation6) (Fig. ). Succinylation at the two lysine residues was detected only under Tween 40-triggered L-glutamate producing conditions.Citation6) In a modeled structure of the OdhI and OdhA complex, Lys132 was predicted to interact electrostatically with Asp523 of OdhA (Fig. (B)). This prompted us to determine the potential impact of protein acylation at Lys132 on OdhI function and L-glutamate production.
In the present study, we show that succinylation at Lys132 decreases the binding ability of OdhI to OdhA, which negatively regulates L-glutamate production. The findings indicate that not only phosphorylation and also acylation of OdhI may regulate L-glutamate production in C. glutamicum.
Materials and methods
Bacterial strains and culture conditions. C. glutamicum ATCC13032 (JCM1318) was used as the wild-type strain. All strains used in this study are shown in Table . A deletion mutant of odhI (KSY22) was constructed by a two-step homologous recombination method,Citation19) as described previously.Citation6) Briefly, upstream and downstream regions (approx. 1 kb) of odhI (NCgl1385) were amplified by PCR using the primer sets delodhI-f1 (AAGGGATCCGTGACCGTTTCCACTCAGATG)/delodhI-soe-r1 (TGTTTTTACTCAGCGTCGCTCATTAAACTTCCTCC) and delodhI-soe-f2 (GTTTAATGAGCGACGCTGAGTAAAAACACTTCCTA)/delodhI-r2 (AAGGGATCCAAGCCATCTGGTTCCATGGTG), respectively, and then joined by splicing by overall extension (SOE)-PCR using the delodhI-f1/delodhI-r2 primers. The resulting PCR products were cloned into the pK18mobsacB-based plasmidCitation19) and introduced into strain ATCC13032 by electroporation. Kanamycin resistance and sucrose tolerance were used as the positive and negative selection markers for the first and second recombination events, respectively. To construct the OdhI-K132 mutants, DNA fragments containing the upstream region, the coding region containing the desired point mutation (K132E, K132Q, or K132R), and the downstream region of odhI were amplified and cloned into the pK18mobsacB-based plasmid, and introduced into strain KSY22 (ΔodhI), followed by two-step homologous recombination. Selected mutants were screened by PCR amplification using the odhI-up1077-f (TCGAAGTAAGCGTTCTCAACG)/odhI-dn1053-r (AGGGCGTCATCAACGTCGAT) primers, which anneal to regions outside the homologous arm regions, and sequenced.
Table 1. Bacterial strains and plasmids used in this study.
C. glutamicum cells were grown in 25 mL of L-glutamate production mediumCitation6) at 31.5 °C in 500 mL baffled flasks on a rotary shaker at 100 rpm. Tween 40 (1.5 g L−1) was added at 3 h to induce L-glutamate production. L-Glutamate level in the culture medium was measured with the L-glutamate assay kit YAMASA NEO (Yamasa Corporation).
Preparation of recombinant Strep-tagged OdhI, His-tagged OdhA, and His-tagged PknG proteins. Recombinant OdhI (wild type and mutant) proteins were expressed as N-terminal Strep-tagged proteins in Escherichia coli BL21-CodonPlus(DE3) (Agilent). The odhI-coding region was amplified from ATCC13032, AA14 (K132R), AA16 (K132E), and AA18 (K132Q) genomic DNA templates by PCR using the Nde-strepodhI-f (GGCATATGTGGAGCCATCCGCAGTTTGAAAAGGGTGGTATGAGCGACAACAACGGC) and Bam-odhI-r (CCGGATCCTTACTCAGCAGGGCCTGCGAGGAAAAC) primers. The resulting PCR products were cloned into an NdeI and BamHI-digested pET-21a vector. The resulting plasmids were named pAA77 (WT), pAA78 (K132R), pAA79 (K132E), and pAA80 (K132Q). E. coli cells were grown in 2 × YT medium (16 g L−1 Bacto tryptone, 10 g L−1 Bacto yeast extract, and 5 g L−1 NaCl) at 37 °C for 3 h and then cultivated at 25 °C for 18 h in the presence of 0.1 mM isopropyl β-D-1-thiogalactopyranoside (IPTG) to induce enzyme expression. The cells were harvested and lysed in buffer-D (100 mM Tris-HCl [pH 8.0] and 150 mM NaCl) containing 1 mM phenylmethylsulfonyl fluoride (PMSF) followed by affinity purification using StrepTactin Sepharose (Qiagen). Strep-tagged proteins were eluted with buffer-D containing 2.5 mM desthiobiotin. The eluted proteins were dialyzed against TESGN buffer (100 mM TES-NaOH [pH 7.6], 150 mM NaCl, and 30% glycerol), and utilized for pull-down and ODH activity assays.
Recombinant OdhA protein was expressed as a C-terminal His-tagged protein in E. coli BL21-CodonPlus(DE3) cells using the pET-21a vector. The odhA (NCgl1084 in KEGG)-coding region was amplified by PCR using the pET21::odhAslice-f (TTAAGAAGGAGATATACATATGAGCAGCGCTAGTA) and pET21::odhAslice-r (GTCGACGGAGCTCGAATTCTCAATGGTGATGGTGG) primers and cloned into pET-21a. E. coli cells were grown in 2 × YT medium at 37 °C for 3 h and then cultivated at 25 °C for 18 h in the presence of 0.1 mM IPTG to induce enzyme expression. The cells were harvested and lysed in buffer-A (20 mM Tris-HCl [pH 8.0] and 150 mM NaCl) containing 1 mM PMSF, followed by affinity purification using Ni-NTA His Bind resin (Millipore). His-tagged proteins were eluted with buffer-A containing 200 mM imidazole. The eluted proteins were dialyzed against TESGN buffer and used for pull-down assays.
Recombinant PknG protein was expressed as a C-terminal His-tagged protein in E. coli BL21-CodonPlus(DE3) cells using the pET-21a vector. The pknG (NCgl2655 in KEGG)-coding region was amplified by PCR using the NdeI-pknG-f (AAACATATGAAGGATAATGAAGAT) and XhoI-pknG-r (AGACTCGAGGAACCAACTCAGTGG) primers, and cloned into an NdeI and XhoI-digested pET-21a. The resulting plasmid was named pAA86. E. coli cells were grown in 2 × YT medium at 37 °C for 3 h and then cultivated at 25 °C for 18 h in the presence of 0.1 mM IPTG to induce enzyme expression. His-tagged PknG protein was purified as described with His-tagged OdhA.
Pull-down assay. Purified Strep-tagged OdhI and His-tagged OdhA proteins were incubated in 0.5 mL of TESGN buffer at 30 °C for 30 min and then subjected to affinity purification using Ni-NTA resin. His-tagged proteins were eluted with TESGN buffer containing 250 mM imidazole and separated by 15% SDS-PAGE followed by Coomassie Brilliant Blue staining. Densitometric analysis of protein bands was performed using ImageQuantTL ver. 8.1 software (GE Healthcare). The negative control lacked His-tagged OdhA protein.
ODH activity assay. ODH activity was assayed at 30 °C as previously describedCitation20) with minor modifications. Briefly, C. glutamicum cells were lysed in TESG30 buffer (100 mM TES-NaOH [pH 7.6] and 30% glycerol) containing 1 mM dithiothreitol (DTT), 1 mM PMSF, 10 μg mL−1 DNase, and 10 μg mL−1 RNase by exposure to high pressure using EmulsiFlex-B15 (Avestin). After removal of cell debris by centrifugation (7,000 × g, 10 min, 4 °C), the cleared lysate was subjected to buffer exchange with TESG30 buffer using a PD10 column (GE Healthcare). Cell-free extracts containing 0.5 to 1.5 mg protein were incubated in a reaction mixture (1 mL) containing 100 mM TES-NaOH (pH 7.6), 0.2 mM coenzyme A, 0.3 mM thiamine pyrophosphate, 3 mM L-cysteine, 5 mM MgCl2, 1 mM of an oxidized form of 3-acetylpyridine adenine dinucleotide (APAD+; Oriental Yeast Co., Ltd.), and 1 mM 2-oxoglutarate. The reaction was started by the addition of the cell extract and the initial rate of APAD+ reduction was monitored spectrophotometrically at 365 nm. Endogenous APAD+ reduction in the absence of 2-oxoglutarate was subtracted from the APAD+ reduction in the presence of 2-oxoglutarate. One unit of ODH activity was defined as the reduction of 1 μmol of APAD+ per min.
Inhibitory assay of OdhI on ODH activity was performed as described previously.Citation11) Cell-free extracts of C. glutamicum KSY22 (∆odhI) was prepared from cells cultivated in L-glutamate production medium for 9 h without induction, and preincubated with purified Strep-tagged OdhI protein at 30 °C for 10 min before the reaction was started by addition of cofactors and substrates.
In vitro succinylation of OdhI protein. Purified Strep-tagged OdhI protein (60 μg) was incubated with indicated concentrations of succinyl-CoA in 120 μL of 100 mM TES buffer (pH 7.6) at 30 °C for 30 min. Succinylation of OdhI was detected by western blot using a home-made rabbit polyclonal anti-succinyllysine primary antibody (1:2000 dilution).Citation21) A rabbit polyclonal anti-OdhI antibody (1:3000 dilution)Citation22) was used to detect OdhI protein. Mass spectrometry (MS) analysis was performed to identify succinylation sites of OdhI as described previously.Citation6) Briefly, after in vitro succinylation, Strep-tagged OdhI protein was reduced with 20 mM DTT and subsequently alkylated with 30 mM iodoacetamide, and digested with sequence-grade trypsin (Promega). The proteolytic peptides were analyzed by reverse-phase nano HPLC-MS/MS using a Q Exactive mass spectrometer (Thermo Fisher Scientific). The acquired MS raw data were processed using Proteome Discoverer (ver. 1.4, Thermo Fisher Scientific). Only spectra with expectation values less than 1% of the false discovery rate were accepted for peptide identification and peptides with Mascot ion scores over 50 were used for semi-quantification analysis. Semi-quantification of succinylated peptides was based on the ratio of the peak areas of the peptides, after normalization with the amount of OdhI, which is given as the protein area by Proteome Discoverer.
In vitro phosphorylation of OdhI protein. In vitro phosphorylation of OdhI was performed as described previouslyCitation11,23) with minor modifications. Purified His-tagged OdhI protein (7 μg) was incubated with purified His-tagged PknG (40 μg) in a 100 μL reaction mixture containing 20 mM Tris-HCl (pH 7.5), 5 mM MgCl2, 2 mM MnCl2, 1 mM DTT, 0.2 mM orthovanadate, and 2 mM ATP at 37 °C for 30 min. An aliquot of the reaction mixture was used for the ODH activity assay. Phosphorylation of OdhI was confirmed by Phos-tag SDS-PAGE (Wako) according to the manufacturer’s protocol.
Results
Succinylation-mimic mutation of OdhI-K132 reduces binding to OdhA
To examine the effect of protein acylation at K132 of OdhI, we replaced this residue with glutamine (acetylation mimic), glutamate (succinylation mimic), and arginine (non-acylation mimic). We performed pull-down analysis with purified Strep-tagged OdhI and His-tagged OdhA proteins to determine the effect of these mutations on the binding ability of OdhI to OdhA. The K132E succinylation-mimic mutation substantially decreased the binding ability to OdhA (Fig. (A)). In contrast, the K132R non-acylation mimic mutation did not substantially affect the binding ability, and K132Q acetylation-mimic mutation had a partial effect on the binding ability. When we performed pull-down analysis with various concentrations of OdhI, the binding of the wild type and K132R variants to OdhA was saturated at a concentration of 4 μM. On the other hand, less K132E as well as K132Q variants bound to OdhA at 1 μM and binding was not saturated even at 8 μM (Fig. (B)), suggesting the decreased affinity of the mutant proteins to OdhA. The results with the K132E succinylation-mimic mutation suggested that succinylation at K132 of OdhI decreased the interaction of OdhI with OdhA.
Succinylation-mimic mutation of OdhI-K132 reduces the ability to inhibit ODH in vitro
We next examined the effect of the acylation mimic mutations on the inhibitory activity of OdhI for ODH. We added the wild type and K132-mutated variants of Strep-tagged OdhI to cell-free extracts of ΔodhI strain and measured the ODH activity (Fig. ). The activity was reduced by addition of the wild-type OdhI protein, as reported previously.Citation11) K132E as well as K132Q variant of OdhI produced no substantial change in the ODH activity. The inhibitory activities of the K132E and K132Q variants were 9% and 14% of that of the wild type, respectively (Fig. ). The K132R variant showed a partial inhibitory activity (67% of the wild type). These results were consistent with those of the pull-down assay (Fig. ), and indicated that the K132E succinylation-mimic as well as K132Q acetylation-mimic mutations decreased the ability of OdhI to inhibit ODH due to the decreased binding affinity to OdhA.
In vitro succinylation of OdhI decreases the inhibitory effect on ODH activity
Analysis of the K132 acylation-mimic and non-acylation mimic mutants suggested that succinylation at K132 might negatively affect the ability of OdhI to inhibit the ODH activity. To obtain evidence to support this hypothesis, we performed the ODH inhibition assay using in vitro succinylated OdhI protein. Since several proteins are succinylated non-enzymatically by succinyl-CoA,Citation24) Strep-tagged OdhI protein was incubated with succinyl-CoA at concentrations of 0, 0.1, 1, or 10 mM. Increasing concentration of succinyl-CoA produced an increased amount of three slow migrating bands that were immunoreactive with anti-OdhI antibody (Fig. (A)). Among the three bands, the two upper bands were immunoreactive to anti-succinyllysine antibody. These results indicated that the lowest band corresponded to the unsuccinylated OdhI and the shifted bands to the succinylated OdhI. This was similar to phosphorylated OdhI, as reported previously.Citation17,22) Mass spectrometry analysis confirmed succinylation at K24, K52, and K132 of OdhI by this non-enzymatic reaction, and the succinylation levels increased with the increase in the concentration of succinyl-CoA (Fig. (A) and (B)). The two shifted bands in western blot analysis were considered to reflect multiple succinylation of OdhI, and were observed when the OdhI-K132R variant was incubated with succinyl-CoA (data not shown). We added the in vitro succinylated OdhI proteins to the ΔodhI lysate and measured the ODH activity. The inhibitory activity of the wild-type OdhI on ODH was attenuated with increased succinylation of OdhI, while this effect of succinylation was not observed with the K132R variant (Fig. (C)). These results strongly suggested that succinylation at K132 was responsible for negative regulation of the OdhI function.
The effect of succinylation on the phosphorylation-dependent OdhI function
Lys132 was predicted to interact electrostatically with Glu52 in the three-dimensuional structure of the phosphorylated form of OdhI (Fig. (A)). We considered a possibility that succinylation at K132 might inhibit the phosphorylation-dependent conformational change in OdhI by electrostatic repulsion, which may interfere with the inhibitory effect of OdhI on ODH activity. To understand the relationship between the succinylation and phosphorylation as post-translational modifications, we prepared phosphorylated OdhI proteins using purified PknG protein kinase in vitro and performed the ODH inhibition assay. PknG-dependent phosphorylation completely abolished the ability of non-succinylated OdhI to inhibit ODH, while succinylation had a partial inhibitory effect on the OdhI ability (Fig. (B)), second and third columns from the left). A similar effect of phosphorylation was observed with the succinylated OdhI (Fig. (B)), fourth and fifth columns from the left). These results suggested that succinylation did not interfere with the phosphorylation-dependent conformational change and OdhI function to inhibit ODH activity.
Succinylation inhibits glutamate production by the OdhI-K132 mutants
The observation that the K132E succinylation-mimic mutation reduced the ability to inhibit the ODH activity suggested that this mutation may negatively affect L-glutamate production. We examined L-glutamate production of the wild type and the K132 mutant strains under Tween 40-induced conditions (Fig. ). The OdhI-deleted strain displayed substantially decreased L-glutamate production (1.7 g L-1) compared to the wild type (23.3 g L−1), as reported previously.Citation17) Consistent with the results of pull-down and ODH inhibition assays in vitro, the K132E mutant strain showed a significantly decreased L-glutamate production (11.5 g L−1) compared to the wild type and K132R non-acylation mimic mutant (18.4 g L−1) strains. The K132Q acetylation-mimic mutant strain also showed a significantly decreased L-glutamate production (14.7 g L−1) compared to the wild type strain. The defect was more moderate than the K132E mutant, which was consistent with the result of pull-down assay (Fig. ). The collective results indicate that succinylation as well as acetylation at K132 had a negative effect on L-glutamate production due to inhibition of the binding of OdhI to OdhA.
Discussion
The present data are the first evidence that acylation (especially succinylation) at K132 inhibits the binding of OdhI to OdhA, which likely has a negative effect on L-glutamate production. Mycobacterium tuberculosis GarA, a homologous protein of C. glutamicum OdhI, negatively regulates α-ketoglutarate dehydrogenase, glutamate dehydrogenase, and glutamate synthase.Citation25) The substitution of glutamate for Lys144 on GarA, which corresponds to the OdhI-K132E mutation, abolishes the ability to inhibit these enzymes.Citation26) Our result with the OdhI-K132E mutant agrees well with the result with GarA-K144E, suggesting that Lys132 has a critical role in the binding to OdhA.
In our acylome analysis, succinylation at K132 was detected only under Tween 40-triggered L-glutamate-producing conditions, while K132 acetylation was unchanged between L-glutamate-producing and non-producing conditions.Citation6) It is likely that in the L-glutamate-producing conditions, a large portion of the carbon flux is shifted toward the anaplerotic pathway mediated by phosphoenolpyruvate carboxylase, rather than the acetyl phosphate and acetate-producing Pta-AckA pathways, with the resulting depletion of acetylation substrates.Citation6,7) In this condition, succinylation utilizing succinyl-CoA would readily occur. Although we have not identified an enzyme responsible for K132 succinylation, a non-enzymatic mechanism might be considered, as suggested in mitochondria.Citation24,27) Under Tween 40 and penicillin-induced L-glutamate-producing conditions, most synthesized OdhI is unphosphorylated, which results in decreased ODH activity.Citation22) Presently, succinylation at K132 of OdhI resulted in retention of the ODH activity and had a negative effect on L-glutamate production (Figs. and ). Non-enzymatic succinylation at K132 of OdhI might represent carbon stress, as mentioned elsewhere.Citation28)
Regulation of the OdhI function by phosphorylation has been well studied.Citation11,16,22) The results of the present study indicated that OdhI is also regulated by protein succinylation, and suggested the potential effect of OdhI succinylation on L-glutamate production. As phosphorylation and acylation often interfere and/or cooperate with each other in the regulation of protein function,Citation29,30) it would be interesting to understand the impact of the two major post-translational modifications on protein regulation related to metabolism and biology in C. glutamicum.
Author contributions
SKo designed and supervised this study. AKA, MNS, and SKu performed the experiments and data analysis. SKo wrote the manuscript with the assistance of HK, MY, and MK. AKA and MNS equally contributed to this work.
Disclosure statement
The authors declare no conflict of interest associated with this manuscript.
Funding
This work was supported by JSPS KAKENHI [grant number 21580105]; the Institute for Fermentation, Osaka to SKo.
Acknowledgements
Strain JCM 1318 was provided by the Japan Collection of Microorganisms, RIKEN-BRC, which is a participant in the National Bioresource Project of MEXT, Japan. We thank Takashi Hirasawa and Hiroshi Shimizu for providing an anti-OdhI antibody. We also thank Kaori Ohtsuki, Masaya Usui, and Aya Abe at the RRC center of RIKEN-BSI for their technical assistance with the mass spectrometry analysis. Some of the authors, including the corresponding author, are members of a laboratory that is financially supported by an endowment from Kyowa Hakko Bio Co., Ltd. to the Biotechnology Research Center of The University of Tokyo. The corresponding author is not paid directly by the company, and do not have shares of the company. The company did not have any role in the study design, data collection and analysis, or the preparation of or decision to publish the article.
References
- Choudhary C, Weinert BT, Nishida Y, et al. The growing landscape of lysine acetylation links metabolism and cell signalling. Nat Rev Mol Cell Biol. 2014;15:536–550.10.1038/nrm3841
- Hentchel KL, Escalante-Semerena JC. Acylation of biomolecules in prokaryotes: a widespread strategy for the control of biological function and metabolic stress. Microbiol Mol Biol Rev. 2015;79:321–346.10.1128/MMBR.00020-15
- Menzies KJ, Zhang H, Katsyuba E, et al. Protein acetylation in metabolism — metabolites and cofactors. Nat Rev Endocrinol. 2015;12:43–60.10.1038/nrendo.2015.181
- Yoshida M, Kudo N, Kosono S, et al. Chemical and structural biology of protein lysine deacetylases. Proc Jpn Acad Ser B. 2017;93:297–321.10.2183/pjab.93.019
- Eggeling L, Bott M, editors. Handbook of Corynebacterium glutamicum. CRC Press; 2005.
- Mizuno Y, Nagano-Shoji M, Kubo S, et al. Altered acetylation and succinylation profiles in Corynebacterium glutamicumin response to conditions inducing glutamate overproduction. MicrobiologyOpen. 2016;5:152–173.10.1002/mbo3.2016.5.issue-1
- Nagano-Shoji M, Hamamoto Y, Mizuno Y, et al. Characterization of lysine acetylation of a phosphoenolpyruvate carboxylase involved in glutamate overproduction in Corynebacterium glutamicum. Mol Microbiol. 2017;104:677–689.10.1111/mmi.2017.104.issue-4
- Kawahara Y, Takahashi-Fuke K, Shimizu E, et al. Relationship between the glutamate production and the activity of 2-oxoglutarate dehydrogenase in brevibacterium lactofermentum. Biosci Biotechnol Biochem. 1997;61:1109–1112.10.1271/bbb.61.1109
- Shimizu H, Tanaka H, Nakato A, et al. Effects of the changes in enzyme activities on metabolic flux redistribution around the 2-oxoglutarate branch in glutamate productionby Corynebacterium glutamicum. Bioprocess Biosyst Eng. 2003;25:291–298.10.1007/s00449-002-0307-8
- Shirai T, Nakato A, Izutani N, et al. Comparative study of flux redistribution of metabolic pathway in glutamate production by two coryneform bacteria. Metab Eng. 2005;7:59–69.10.1016/j.ymben.2004.10.001
- Niebisch A, Kabus A, Schultz C, et al. Corynebacterial protein kinase G controls 2-oxoglutarate dehydrogenase activity via the phosphorylation status of the OdhI protein. J Biol Chem. 2006;281:12300–12307.10.1074/jbc.M512515200
- Almawi AW, Matthews LA, Guarné A. FHA domains: phosphopeptide binding and beyond. Prog Biophys Mol Biol. 2017;127:105–110.10.1016/j.pbiomolbio.2016.12.003
- Krawczyk S, Raasch K, Schultz C, et al. The FHA domain of OdhI interacts with the carboxyterminal 2-oxoglutarate dehydrogenase domain of OdhA in Corynebacterium glutamicum. FEBS Lett. 2010;584:1463–1468.10.1016/j.febslet.2010.03.028
- Raasch K, Bocola M, Labahn J, et al. Interaction of 2-oxoglutarate dehydrogenase OdhA with its inhibitor OdhI in Corynebacterium glutamicum: Mutants and a model. J Biotechnol. 2014;191:99–105.10.1016/j.jbiotec.2014.05.023
- Barthe P, Roumestand C, Canova MJ, et al. Dynamic and structural characterization of a bacterial FHA protein reveals a new autoinhibition mechanism. Structure 2009;17:568–578.10.1016/j.str.2009.02.012
- Schultz C, Niebisch A, Schwaiger A, et al. Genetic and biochemical analysis of the serine/threonine protein kinases PknA, PknB, PknG and PknL of Corynebacterium glutamicum: evidence for non-essentiality and for phosphorylation of OdhI and FtsZ by multiple kinases. Mol Microbiol. 2009;74:724–741.10.1111/mmi.2009.74.issue-3
- Schultz C, Niebisch A, Gebel L, et al. Glutamate production by Corynebacterium glutamicum: dependence on the oxoglutarate dehydrogenase inhibitor protein OdhI and protein kinase PknG. Appl Microbiol Biotechnol. 2007;76:691–700.10.1007/s00253-007-0933-9
- Kim J, Fukuda H, Hirasawa T, et al. Requirement of de novo synthesis of the OdhI protein in penicillin-induced glutamate production by Corynebacterium glutamicum. Appl Microbiol Biotechnol. 2010;86:911–920.10.1007/s00253-009-2360-6
- Schafer A, Tauch A, Jager W, et al. Small mobilizable multi-purpose cloning vectors derived from the Escherichia coli plasmids pK18 and pK19: selection of defined deletions in the chromosome of Corynebacterium glutumicum. Gene 1994;145:69–73.10.1016/0378-1119(94)90324-7
- Shiio I, Ujigawa-Takeda K. Presence and regulation of α-ketoglutarate dehydrogenase complex in a glutamate-producing bacterium. Brevibacterium flavum. Agric Biol Chem. 1980;44:1897–1904.
- Kosono S, Tamura M, Suzuki S, et al. Changes in the acetylome and succinylome of Bacillus subtilis in response to carbon source. PLoS ONE. 2015;10:e0131169.10.1371/journal.pone.0131169
- Kim J, Hirasawa T, Saito M, et al. Investigation of phosphorylation status of OdhI protein during penicillin- and Tween 40-triggered glutamate overproduction by Corynebacterium glutamicum. Appl Microbiol Biotechnol. 2011;91:143–151.10.1007/s00253-011-3275-6
- Koul A, Choidas A, Tyagi AK, et al. Serine/threonine protein kinases PknF and PknG of Mycobacterium tuberculosis: characterization and localization. Microbiology 2001;147:2307–2314.10.1099/00221287-147-8-2307
- Wagner GR, Payne RM. Widespread and enzyme-independent Nε-acetylation and Nε-succinylation of proteins in the chemical conditions of the mitochondrial matrix. J Biol Chem. 2013;288:29036–29045.10.1074/jbc.M113.486753
- Ventura M, Rieck B, Boldrin F, et al. GarA is an essential regulator of metabolism in Mycobacterium tuberculosis. Mol Microbiol. 2013;90:356–366.
- Nott TJ, Kelly G, Stach L, et al. An intramolecular switch regulates phosphoindependent FHA domain interactions in Mycobacterium tuberculosis. Sci Signal. 2009;2:ra12.
- Weinert BT, Schölz C, Wagner SA, et al. Lysine succinylation is a frequently occurring modification in prokaryotes and eukaryotes and extensively overlaps with acetylation. Cell Rep. 2013;4:842–851.10.1016/j.celrep.2013.07.024
- Wagner GR, Hirschey MD. Nonenzymatic protein acylationas a carbon stress regulated by sirtuin deacylases. Mol Cell. 2014;54:5–16.10.1016/j.molcel.2014.03.027
- Soufi B, Soares NC, Ravikumar V, et al. Proteomics reveals evidence of cross-talk between protein modifications in bacteria: focus on acetylation and phosphorylation. Curr Opin Microbiol. 2012;15:357–363.10.1016/j.mib.2012.05.003
- van Noort V, Seebacher J, Bader S, et al. Cross-talk between phosphorylation and lysine acetylation in a genome-reduced bacterium. Mol Syst Biol. 2012;8:571.