Abstract
In red wheat, reddish-brown pigments accumulate in testa of mature seeds. Half-cut wheat seeds were immersed in p-dimethylaminocinnamaldehyde (DMACA) reagent that stains flavanol structures blue. Testa of 10–40 days after flowering (DAF) in red wheat (“Norin 61” and “Satonosora”) seeds were stained blue and the reagent color changed to blue with 10–25 DAF seeds. No blue staining was observed in white wheat (“Tamaizumi”) seeds during maturation. “Norin 61” seed coats at 10 DAF contained dihydroquercetin, dihydromyricetin, (+)-catechin, procyanidin B3, and prodelphinidin B3, which were identified by HPLC-diode array detector and LC-MS/MS analyses. These five components began accumulating 7 DAF, reached maxima at 10 or 15 DAF, and then decreased in red wheat seeds, but were not detected in white wheat seeds. These results suggest that flavanol and proanthocyanidins are possible precursors of the reddish-brown pigments of red wheat seeds, and are converted to insoluble compounds as the seeds mature.
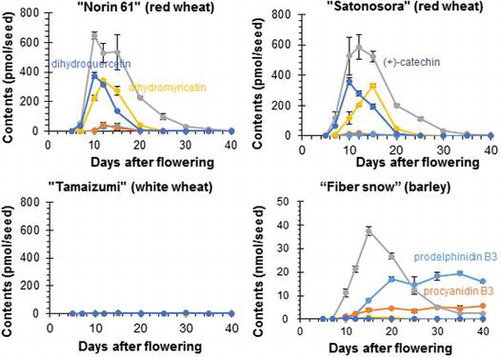
Dihydroflavonols, flavanol, and proanthocyanidin dimers accumulated in immature red wheat seeds, whose mature seed coat has reddish-brown pigments, but not in white wheat seeds.
Wheat (Triticum aestivum L.) is divided into two groups based on seed coat color: red wheat and white wheat. Red seed coats have been associated with the development of seed dormancy and contamination of reddish-brown pigment reduces the brightness of wheat flour during milling.Citation1–3) Although the reaction mechanism has not been determined, red and white wheat seeds can be easily distinguished after treatment with sodium hydroxide, with the former turning a brownish-orange color and the latter turning yellow.Citation4) The red seed coat color in wheat is a highly heritable traitCitation5) that is determined by R genes located on the distal end of the long arms of chromosome 3A, 3B, and 3D.Citation6) The three R genes controlling seed coat color in hexaploid wheat act additively, such that each additional gene results in a darker red color.Citation7) The R genes were identified as three Myb-type transcription factors (Tamyb10-A1 on 3A, Tamyb10-B1 on 3B, and Tamyb10-D1 on 3D) that activated the expression of four flavonoid synthesis genes (chalcone synthase, chalcone isomerase, flavanone 3-hydroxylase, and dihydroflavonol 4-reductase) in developing wheat seedsCitation8) (Fig. ). In barley (Hordeum vulgare L.) seeds, (+)-catechin and proanthocyanidin oligomers, such as procyanidin B3 (PCB3) and prodelphinidin B3 (PDB3), are major phenolic constituents that accumulate during seed maturation.Citation9,10) A proanthocyanidin-free barley mutant, ant28, is Hvmyb10-deficient, which is the barley ortholog of Tamyb10 in red wheat.Citation11) Therefore, red wheat, with a functional Tamyb10 protein, may synthesize (+)-catechin and proanthocyanidins like wild-type barley, which has a functional Hvmyb10 protein.
Fig. 1. Depiction of the flavonoid biosynthesis pathway modified from previous reports.Citation15,24) The scheme is simplified to show the steps leading to proanthocyanidins, flavones, and flavonols. CHS, CHI, F3H, and DFR are chalcone synthase, chalcone isomelase, flavanone 3-hydroxylase, and dihydroflavonol reductase, respectively. Compounds quantified in this study are described in parentheses below the corresponding category name.
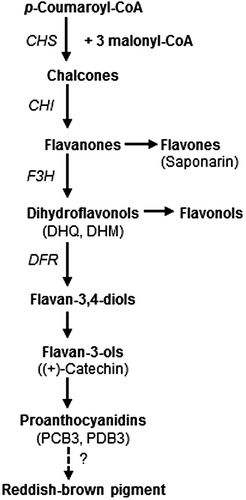
In early studies involving precipitation with lead acetate, Miyamoto and EversonCitation12) reported that the contents of catechin and catechin tannin in premature seed coats of red wheat were about twice as much as those of white wheat, suggesting that catechin tannin was a precursor of a reddish-brown pigment in the seed coat. McCallum and WalkerCitation13) suggested that wheat bran contained (+)-catechin, PCB3, and PDB3. On the contrary, GordonCitation14) reported that there was no clear association between seed coat color and flavanol concentration determined by a vanillin–sulfuric acid assay. Recently, Matus-Cádiz et al.Citation15) reported that red wheat but not white wheat, contained high levels of red pigments throughout their seed coat following vanillin–HCl staining, which suggested the presence of proanthocyanidins in red wheat seed coats. Using Fourier transform ion cyclotron resonance mass spectrometry, the researchers also detected three metabolite groups (flavonol, flavanone, and stilbene) that were abundant in red wheat bran relative to white wheat bran.Citation15)
The precursors of these reddish-brown pigments that accumulate in the seed coats of red wheat have not been identified, and no consistent difference in metabolites between red and white wheat has been conclusively detected. The objective of this study was to identify flavonoid metabolites contained in red, but not white, wheat to determine if they are possible precursors of the reddish-brown pigments. Vanillin–HCl staining is commonly used to detect catechins and proanthocyanidins in seeds,Citation11,15–17) and p-dimethylaminocinnamaldehyde (DMACA) staining is also used for the same purpose.Citation18) Although both vanillin–HCl and DMACA react with the flavanol (catechin) structure, which are present either as monomers or as terminal subunits of proanthocyanidins, the former stains it red and the latter stains it blue. Therefore, DMACA staining has several advantages over vanillin–HCl staining, that include the following: (i) greater DMACA sensitivity; (ii) DMACA makes distinguishing flavanols from co-occurring pink anthocyanin pigments possible in tissues; and (iii) DMACA makes distinguishing flavanols from lignin in plant tissues that can sometimes be stained reddish in the strong HCl of DMACA and vanillin reagents.Citation18) In this study, we used DMACA staining to detect flavanols in immature and mature wheat and barley seeds, and observed stain localization and solubility to acidified methanol (MeOH) during seed maturation. Furthermore, we compared flavonoid compounds among red and white wheat, as well as barley, in mature and developing seeds and identified them using an HPLC-diode array detector (DAD) and LC-MS/MS analyses.
Materials and methods
Plant materials
Wheat cvs. “Norin 61” (red wheat), “Satonosora” (red wheat), and “Tamaizumi” (white wheat) and the six-rowed barley cv. “Fiber snow” were grown in an experimental field in Tsukuba during the 2014–2015 and 2015–2016 growing season. The spikes of three wheat cultivars and barley were tagged at the anthesis and harvested at 2, 5, 7, 10, 12, 15, 20, 25, 30, 35, and 40 days after flowering (DAF). Wheat seeds were collected from the primary and secondary florets of the central spike parts, and barley seeds were collected from the central row of spikes. Collected seeds were stored at −20 °C until use. Mature wheat seeds from a collection of 324 varieties previously describedCitation19) were also used for DMACA staining.
Chemicals
Authentic samples of (+)-catechin and dihydromyricetin (DHM) were purchased from Sigma Chemicals Co. (St. Louis, MO, USA), and dihydroquercetin (DHQ), PCB3, and saponarin were obtained from Funakoshi Co. (Tokyo, Japan). PDB3 was isolated from barley bran and checked for purity before use, according to methods previously described.Citation20) HPLC and LC/MS grade reagents and DMACA were obtained from Wako Pure Chemicals Co (Osaka, Japan).
DMACA staining
Five immature and mature seeds of wheat and barley were longitudinally cut in half with a scalpel and immersed for 1.5 h in a 1 mL solution containing 0.1% (w/v) DMACA-0.12 N HCl in MeOHCitation21) at room temperature. Stained seeds were observed at low magnification.
Extraction of the immature seed coat fraction of red wheat
The seed coat fraction was collected from 10 DAF seeds of 90 “Norin 61” spikes by removing the endosperm and was prepared by freezing with liquid nitrogen, lyophilizing, and grinding in a TI-100 vibrating sample mill (CMT, Tokyo, Japan). Ten grams of the seed coat fraction was extracted with 200 mL of 75% acetone, stirring at 6 °C for 1 h, and filtered through No. 2 filter paper (Advantec Toyo, Tokyo, Japan). The residue was extracted again with 200 ml of 75% acetone, and the combined filtrate was concentrated under vacuum at 35 °C and lyophilized. The extract was resolved in 50 mL of 2.5% acetic acid and applied onto a DPA-6S solid phase extraction cartridge (5 g/60 mL) (Supelco), which was consecutively pretreated with 15 mL of MeOH, 50 mL of ultrapure water, and 20 mL of 2.5% acetic acid, and washed with 2.5% acetic acid to obtain the unbound fraction. Bound constituents were sequentially eluted with 30 mL of MeOH (MeOH1 fraction), another 30 mL of MeOH (MeOH2 fraction), and 30 mL of acetone (acetone fraction). The unbound fraction was lyophilized and resuspended in 5.0 mL of ultrapure water. The other three fractions were evaporated at 35 °C and resuspended in 1.0 mL of MeOH.
Sample preparation for quantifications
The wheat and barley seeds collected 5–10 DAF were lyophilized and homogenized with a mortar and pestle under liquid nitrogen. Seeds collected 12–40 DAF were lyophilized and ground in a TI-100 vibrating sample mill. One hundred milligrams of whole grain flour was extracted with 1.0 mL of 75% acetone for 1 h at room temperature with mild shaking. Following centrifugation at 12,500 rpm for 10 min, the supernatant was collected and the residue was re-extracted twice, each time with 1.0 mL of 75% acetone. The combined extracts were evaporated at 30 °C and resuspended with 3.0 mL of 2.5% acetic acid. For the flavanol analysis of wheat samples, the extract was applied onto a DPA-6S cartridge (250 mg/3 mL), which was consecutively pretreated with 1.0 mL of MeOH, 3.0 mL of ultrapure water and 1.0 mL of 2.5% acetic acid. After washing with 2.5 mL of 2.5% acetic acid and 1.2 mL of MeOH, a crude flavanol fraction was eluted with 1.8 mL of MeOH, evaporated, and stored at −20 °C. The crude flavanol fraction was resuspended with 200 μL of MeOH and filtered through a 0.20 μm membrane filter (Millex LG) just before LC-MS/MS analysis. For the saponarin analysis of wheat samples and flavanol analysis of barley samples, the extract was applied onto an Autoprep EDS-1 cartridge (50 mg/3 mL) instead of a DPA-6S, washed with 3.0 mL of 2.5% acetic acid, and then eluted with 700 μL of MeOH. The crude phenolic fractions were then resuspended in 100 μL of MeOH. Barley samples were further diluted with MeOH (1:100) and filtered through a 0.20 μm filter (Millex LG) for LC-MS/MS analysis.
Analysis of HPLC-DAD
An HPLC equipped with an SPD-M20A DAD system (Shimadzu, Kyoto, Japan) was used to separate flavonoid compounds. Chromatographic separation was performed at 30 °C on a 150 mm × 4.6 mm i.d. TSKgel ODS-100 V column (Tosoh, Tokyo, Japan). It was eluted with a gradient mobile phase consisting of 20 mM sodium ammonium buffer, pH 3.0 (eluent A), and acetonitrile (eluent B) at 1.0 mL/min. For the analysis of seed coat fractions, the gradient was programmed as follows: 0–5 min, 100% A; 5–35 min, 0–30% B in A; 35–40 min, 30–50% B in A; 40–45 min, 50% B in A; 45–47 min, 50–0% B in A; and 47–57 min, 100% A. Elution was scanned from 240 to 440 nm and monitored for absorbance at 280 nm. Aliquots of the MeOH2 fraction were mixed with authentic sample mixtures of (+)-catechin, PCB3, PDB3, DHQ, and DHM, then co-eluted in the same conditions. For the quantification of saponarin, the gradient was programmed as follows: 0–30 min, 10–25% B in A; 30–35 min, 25–50% B in A; 35–40 min, 50% B in A; 40–42 min, 50–10% B in A; and 42–52 min, 10% B in A. Elution was scanned from 240 to 440 nm and monitored for absorbance at 336 nm. Authentic saponarin of 0.2–1.5 μg was analyzed under the same conditions, and the peak area was calculated at 336 nm.
LC-MS/MS analysis
Identification and quantification were performed with a 4000 Q TRAP® LC-MS/MS system (Sciex, Redwood City, CA, USA) equipped with an electrospray ionization source (turbo V) and 1290 Infinity HPLC system (Agilent, Waldbronn, Germany). Chromatographic separation was performed at 40 °C on a 150 mm × 2.1 mm i.d. Acquity UPLC HSS T3 column (Waters, Milford, MA, USA). Eluents were composed of 0.5 mM ammonium acetate/acetic acid (99.9/0.1, v/v) (eluent A) and acetonitrile/acetic acid (99.9/0.1, v/v) (eluent B). Elution was conducted at a flow rate of 0.40 mL/min with a linear gradient as follows: 0–2 min, 1% B in A; 2–7 min, 1–30% B in A; 7–9 min, 30–90% B in A; 9–10 min, 90% B in A; 10–10.1 min, 90–1% B in A; and 10.1–14.0 min, 1% B in A. The injection volume was 5 μL. MS parameters were operated in a negative scheduled multiple reaction monitoring mode. The conditions of the interface were as follows: ion spray voltage, −4500 V; source temperature, 450 °C; curtain gas pressure, 10 psi; collision activated dissociation gas, 8 psi; nebulizing gas pressure, 60 psi; and turbo gas pressure, 70 psi. A summary of MS/MS detection parameters is shown in Table . Analyst® 1.6.2 software was used for data acquisition and processing. Standard mixtures of authentic samples were diluted to 10, 20, 50, 100, 200, 500, 1000, and 2000 ppb (μg/L) with MeOH.
Table 1. MS/MS detection parameters for five analytes.
Results and discussion
When longitudinally half-cut mature seeds were immersed in DMACA reagent, the orange color of the reagent did not change color for either red or white wheat (Fig. (A–C)). However, barley seeds immediately changed to a blue color (Fig. (D)). By contrast, the testa layer of red wheat cvs. “Norin 61” and “Satonosora” changed to a blue color, similar to that by the barley cv. “Fiber snow,” but in white wheat cv. “Tamaizumi,” the testa demonstrated no response to DMACA treatment (Fig. (E–H)). This blue staining of the testa was most evident around the embryo. Among 324 wheat varieties, the mature seeds of 309 varieties of red wheat also revealed a blue-stained testa, while the remaining white wheat varieties showed no staining of the testa (data not shown). Therefore, blue staining of the testa following DMACA reagent treatments provides conclusive evidence for a difference in flavanol content that is responsible for seed coat colors of wheat. No staining of white wheat seeds by DMACA reagent is consistent with a previous finding using vanillin–HCl staining in proanthocyanidin-free mutant barley seeds.Citation16) These results suggest that mature seeds of red wheat accumulate compounds with flavanol structures that are insoluble in acidified MeOH in the testa, whereas those of white wheat do not accumulate these compounds.
Fig. 2. Mature wheat and barley seeds treated with DMACA reagent.
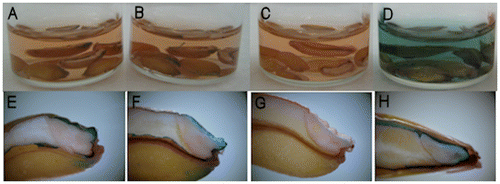
Wheat and barley seeds collected during maturation and harvested in 2016 revealed different patterns when stained with DMACA reagent (Fig. ). In barley seeds, soluble blue staining was observed immediately at 10–40 DAF. In red wheat (“Norin 61” and “Satonosora”) seeds, soluble blue staining was observed at 10–25 or 10–30 DAF, although there was faint staining compared to that in barley seeds. By contrast, no blue staining was observed in white wheat seeds during maturation. Therefore, a soluble flavanol that is subsequently converted to insoluble compounds as the seeds mature, appears to be present in immature red wheat seeds, and the total flavanol of red wheat was low compared to that of barley seeds.
Fig. 3. DMACA staining of wheat and barley seeds at developing stages (2–40 DAF) harvested in 2016.
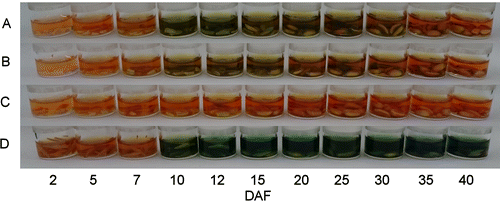
To identify the flavanol compounds present in immature red wheat seeds, the seed coat fraction of 10 DAF “Norin 61” was collected and extracted. The 75% acetone extract from 10 g of the seed coat fraction was separated into the unbound fraction (4264 mg), MeOH1 fraction (121 mg), MeOH2 fraction (22 mg), and acetone fraction (2 mg) by a solid phase extraction. Among the 36 peaks detected at 280 nm in MeOH2 fraction by HPLC–DAD analysis (Fig. ), 9 peaks with a flavanol-like absorption spectrum (λmax = 278 nm) and 2 peaks with a dihydroflavonol-like absorption spectrum (λmax = 290 nm) were observed, although they could not be detected before DPA-6S separation. Based on the comparisons of retention times and ultraviolet absorption spectra to authentic samples as well as co-elution, five peaks (1–5) in the MeOH2 fraction corresponded with PDB3, PCB3, (+)-catechin, DHM, and DHQ, respectively (Fig. ). When the MeOH2 fraction was analyzed by LC-MS/MS, these five compounds were detected at the same retention time as the authentic samples, confirming their identities. In a previous report, (+)-catechin, PCB3, and PDB3 were reported to be isolated from wheat bran,Citation13) but they were separated by thin layer chromatography and determined without structure analyses or reference to authentic proanthocyanidin standards. The consistency of our results with authentic samples provides the first evidence for the presence of PDB3 and PCB3 in red wheat.
Fig. 4. Chromatogram of the MeOH2 fraction from a “Norin 61” seed coat at 10 DAF.
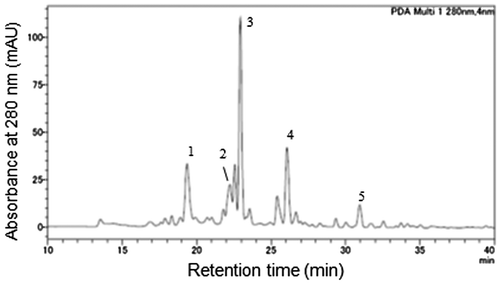
To determine how dihydroflavonol, flavanol, and proanthocyanidin accumulated during seed maturation, contents of DHQ, DHM, (+)-catechin, PCB3, and PDB3 in seeds at each maturing stage (5–40 DAF) were quantified by LC-MS/MS. Quantification results in wheat and barley seeds are shown in Fig. . In white wheat “Tamaizumi” seeds, the five compounds were rare or not detected at any stage of maturation (Fig. (C)). In red wheat “Norin 61” seeds, DHQ and DHM were detected at 7–20 DAF (max. 375 ± 20 pmol/seed at 10 DAF) and 7–25 DAF (max. 343 ± 10 pmol/seed at 12 DAF), respectively (Fig. (A)), and they were similarly detected in “Satonosora” (Fig. (B)). They were also detected in barley seeds at 10–15 DAF (max. 0.4 ± 0.0 nmol/seed at 10 DAF) and at 10–25 DAF (max. 0.8 ± 0.1 nmol/seed at 15 DAF), respectively (Fig. (D)). The contents and changes of both dihydroflavonols in red wheat seeds were approximately similar to those in barley seeds. The temporary accumulation of DHQ and DHM in wheat and barley seeds supports their role as precursors of flavanols and other metabolites (Fig. ). During seed maturation, (+)-catechin started accumulating at 7 DAF and reached a maximum at 10 DAF (646 ± 24 pmol/seed) in “Norin 61” seeds or at 12 DAF (586 ± 83 pmol/seed) in “Satonosora” seeds. In mature red wheat seeds (40 DAF), the (+)-catechin content was only about 5 pmol/seed in both cultivars. In “Fiber snow” barley seeds, (+)-catechin started accumulating at 10 DAF, reached a maximum at 15 DAF (37.5 ± 1.9 nmol/seed), and remained 2.4 ± 0.2 nmol/seed at 40 DAF (matured) (Fig. (D)). The presence of (+)-catechin contents in “Norin 61” and “Satonosora” was less than 2% of what was present in “Fiber snow” at the maximum time, and was less than 0.15% of what was present in “Fiber snow” when mature. In addition, PCB3 and PDB3 were detected at 7–25 DAF (max. 44.3 ± 18.2 pmol/seed at 12 DAF) and 10–20 DAF (35.1 ± 20.1 pmol/seed at 15DAF), respectively, in “Norin 61” seeds, and at 7–20 DAF (max. 18.2 ± 7.0 pmol/seed at 12 DAF) and 7–20 DAF (max. 13.5 ± 3.3 pmol/seed at 15 DAF), respectively, in “Satonosora” seeds. The contents of both proanthocyanidin dimers were low compared to those of dihydroflavonols and catechin in red wheat seeds (Fig. (A–B)). A temporary and small accumulation of PCB3 and PDB3 could suggest that they were quickly metabolized into other compounds in red wheat seeds. On the contrary, both proanthocyanidin dimers started accumulating at 10 DAF and remained at almost maximum levels (5.69 ± 0.10 and 16.04 ± 0.42 nmol/seed, respectively) at 40 DAF in “Fiber snow” seeds (Fig. (D)). Because “Fiber snow” is a relatively low flavanol-accumulating cultivar among barley,Citation22) the observed levels of (+)-catechin and proanthocyanidins found in red wheat seeds indicate that their flavanol content is much lower compared to that in most barley seeds.
Fig. 5. Contents of five flavonoid constituents in seeds during maturation.
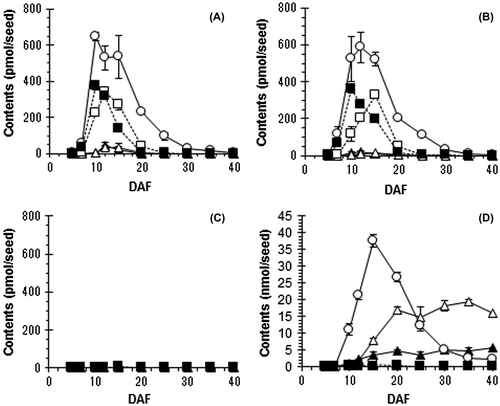
Consistent with a previous report,Citation23) several peaks with a flavone-like absorption spectrum were detected in mature wheat seeds. One such peak was identified as saponarin (apigenin-6-C-glucoside-7-O-glucoside) based on co-elution and comparison with an authentic sample in HPLC–DAD analyses. Mature wheat seeds contained copious amounts of saponarin (>2.5 nmol/seed) compared to (+)-catechin, regardless of seed coat colors (Fig. ). Although the R genes controlling seed coat color are transcription factors of flavonoid synthesis enzymes,Citation8) saponarin may be synthesized by regulating other transcription factors in white wheat seeds or it is transported from other organs.
Fig. 6. Saponarin content in the mature wheat seeds harvested in 2016.
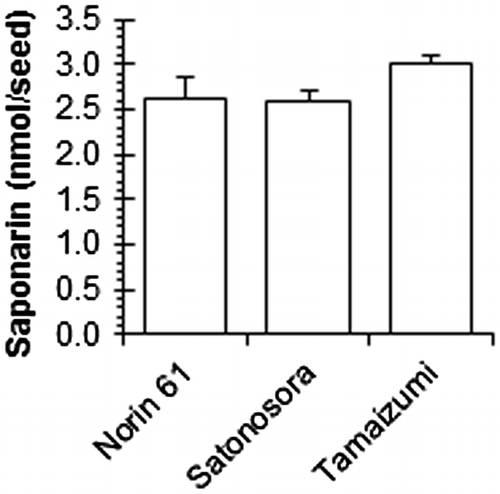
In Fig. , DMACA reagent color in 10–25 DAF “Norin 61” seeds were deeper than those of “Satonosora”. Immature “Norin 61” seeds (10–25 DAF) contained more PCB3 and PDB3 than that in “Satonossora,” although (+)-catechin levels in both cultivars were much the same (Fig. ). Therefore, blue staining by DMACA reagent might be affected by soluble proanthocyanidins. The results of DMACA staining and quantification of proanthocyanidins corresponded with each other. In white wheat seeds, no blue staining was observed by DMACA reagent (Fig. ), and no proanthocyanidin was detected by LC-MS/MS analysis during seed maturation (Fig. ). In red wheat seeds, soluble compounds stained blue with DMACA were observed at 10–25 DAF (Fig. ), and proanthocyanidins (PCB3 and PDB3) that accumulated early were not detected in later stages of seed maturation (Fig. ). The observation that the insoluble blue staining of mature red wheat seed testa suggests that the reduction of PCB3 and PDB3 in later stages could be caused by their conversion into insoluble and unidentified polymers rather than by degradation (Fig. ). Because both “Norin 61” and “Satonosora” have three R genes, the difference in proanthocyanidin content in 12–15 DAF seeds between these cultivars should not be caused by the number of R genes. Further studies are necessary to elucidate the relationship between soluble flavanols in developing seeds and reddish-brown pigments in mature seeds. Investigation of quantitative regulation mechanism of flavanols in developing red wheat seeds is also needed.
In summary, we identified a clear difference in flavonoid constituents between red and white wheat seeds. Dihydroflavonols (DHQ, DHM), flavanol [(+)-catechin], and proanthocyanidins (PCB3, PDB3) accumulated in developing seeds of red but not white wheat. This should be consistent with the gene expression of Tamyb10 in developing seeds, which encodes a transcription factor of flavonoid synthesis enzymes.Citation8) On the contrary, flavones such as saponarin were observed in mature seeds of both red and white wheat, suggesting that not all flavonoid metabolites are related to seed coat color. Therefore, DHQ, DHM, (+)-catechin, PCB3, and PDB3 are strongly implicated as precursors of reddish-brown pigments in the seed coat of red wheat.
Author contributions
N.K., M.C., and H.M. conceived and designed the experiments. H.N., Y.M., and H.O. designed and performed the LC-MS/MS analyses. N.K. performed the experiment, analyzed data, and wrote the manuscript. All authors approved the manuscript.
Disclosure statement
No potential conflict of interest was reported by the authors.
Supplemental data
Supplemental data for this article can be accessed at https://doi.org/10.1080/09168451.2017.1373589.
Supplimental_Table_1.pdf
Download PDF (207.8 KB)Fig._S1.pdf
Download PDF (145.2 KB)Supplimental_Table_2.pdf
Download PDF (28.2 KB)Fig._S2.pdf
Download PDF (98.4 KB)Notes
Abbreviations: DMACA, p-dimethylaminocinnamaldehyde; DAF, days after flowering; DAD, diode array detector; PCB3, procyanidin B3; PDB3, prodelphinidin B3; DHM, dihydromyricetin; DHQ, dihydroquercetin; MeOH, methanol.
References
- Flintham JE. Different genetic components control coat-imposed and embryo-imposed dormancy in wheat. Seed Sci. Res. 2000;10:43–50.10.1017/S0960258500000052
- Warner RL, Kudrna DA, Spaeth SC, et al. Dormancy in white-grain mutants of Chinese Spring wheat (Triticum aestivum L.). Seed Sci Res. 2000;10:51–60.
- Himi E, Mares DJ, Yanagisawa A, et al. Effect of grain colour gene (R) on grain dormancy and sensitivity of the embryo to abscisic acid (ABA) in wheat. J Exp Bot. 2002;53:1569–1574.10.1093/jxb/erf005
- Lamkin WM, Miller BS. Note on the use of sodium hydroxide to distinguish red wheats from white common, club, and durum cultivars. Cereal Chem. 1980;57:293–294.
- Cooper DC, Sorrells ME. Selection for white kernel color in the progeny of red/white wheat crosses. Euphytica. 1984;33:227–232.10.1007/BF00022770
- Metzger RJ, Silbaugh BA. Location of genes for seed coat color in hexaploid wheat. Triticum aestivum L. Crop Sci. 1970;10:495–496.10.2135/cropsci1970.0011183X001000050012x
- Baker RJ. Inheritance of seed coat color in eight spring wheat cultivars. Can J Plant Sci. 1981;61:719–721.10.4141/cjps81-100
- Himi E, Noda K. Red grain colour gene (R) of wheat is a Myb-type transcription factor. Euphytica. 2005;143:239–242.10.1007/s10681-005-7854-4
- Kristiansen KN. Biosyntheses of proanthocyanidins in barley: Genetic control of the conversion of dihydroquercetin to catechin and procyanidins. Carlesberg Res Commun. 1984;49:503–524.10.1007/BF02907552
- Kohyama N, Ono H, Yanagisawa T. Changes in anthocyanins in the grains of purple waxy hull-less barley during seed maturation and after harvest. J Agric Food Chem. 2008;56:5770–5774.10.1021/jf800626b
- Himi E, Yamashita Y, Haruyama N, et al. Ant28 gene for proanthocyanidin synthesis encoding the R2R3 MYB domain protein (Hvmyb10) highly affects grain dormancy in barley. Euphytica. 2012;188:141–151.10.1007/s10681-011-0552-5
- Miyamoto T, Everson EH. Biochemical and physiological studies of wheat seed pigmentation. Agron J. 1958;50:733–734.10.2134/agronj1958.00021962005000120005x
- McCallum JA, Walker JRL. Proanthocyanidins in wheat bran. Cereal Chem. 1990;67:282–285.
- Gordon IL. Selection against spouting damage in wheat. III Dormancy, germinative alpha-amylase, grain redness and flavanols. Aust J Agric Res. 1979;30:387–402.10.1071/AR9790387
- Matus-Cádiz MA, Daskalchuk TE, Verma B, et al. Phenolic compounds contribute to dark bran pigmentation in hard white wheat. J Agric Food Chem. 2008;56:1644–1653.10.1021/jf072970c
- Aastrup S, Outtrup H, Erdal K. Location of the proanthocyanidins in the barley grain. Carlesberg Res Commun. 1984;49:105–109.10.1007/BF02913969
- Debeaujon I, Léon-Kloosterziel KM, Koornneef M. Influence of the testa on seed dormancy, germination, and longevity in Arabidopsis. Plant Physiol. 2000;122:403–413.10.1104/pp.122.2.403
- Abrahams S, Tanner GJ, Larkin PJ, et al. Identification and biochemical characterization of mutants in the proanthocyanidin pathway in Arabidopsis. Plant Physiol. 2002;130:561–576.10.1104/pp.006189
- Chono M, Matsunaka H, Seki M, et al. Molecular and genealogical analysis of grain dormancy in Japanese wheat varieties, with specific focus on MOTHER OF FT AND TFL1 on chromosome 3A. Breeding Sci. 2015;65:103–109.10.1270/jsbbs.65.103
- Kohyama N, Fujita M, Ono H, et al. Effects of phenolic compounds on the browning of ooked barley. J Agric Food Chem. 2009;57:6402–6407.10.1021/jf901944 m
- Nagel CW, Glories Y. Use of a modified dimethylaminocinnamaldehyde reagent for analysis of flavanols. Am J Enol Vitic. 1991;42:364–366.
- Kohyama N, Nagamine T, Murata M. Relationship between browning and changes in flavanols of cooked barley after incubation. J Jpn Soc Food Sci Technol. 2010;57:372–379. Japanese.10.3136/nskkk.57.372
- Michael HN, Guergues SN, Sandak RN. Some polyphenolic constituents of Triticum aestivum (Wheat bran, Sakha 69) and their antibacterial effect. Asian J Chem. 1998;10:256–263.
- Winkel-Shirley B. Flavonoid biosynthesis. A colorful model for genetics, biochemistry, cell biology, and biotechnology. Plant Physiol. 2001;126:485–493.10.1104/pp.126.2.485