Abstract
We investigated the effects of dietary supplementation of difructose anhydride III (DFA III), raffinose (Raf), and fructooligosaccharides (FOS) on diet-induced obesity development. Male rats were fed normal or high-fat and high-sucrose (HFS) diet, with or without supplementing (3%) DFA III, Raf, or FOS, for 8 or 5 weeks. Supplementing DFA III to the HFS diet decreased energy intake compared to the non-supplemented HFS diet. Accordingly, body weight gain and fat accumulation reduced in DFA III-fed rats. Cecal acetate production and plasma glucagon-like peptide-1 (GLP-1) and peptide-YY (PYY) were elevated in DFA III-fed rats, while Raf and FOS partially affected these parameters. These results demonstrate that DFA III has suppressive effect on excessive energy intake driven by the palatable obesogenic diet, possibly due to combined effects of increased anorexigenic factors such as cecal acetate production and GLP-1/PYY secretion.
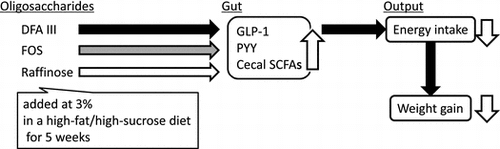
Overeating is one of the critical triggers of obesity that lead to metabolic diseases such as glucose intolerance, dyslipidemia, hypertension, diabetes, and artherogenesis, not only in adults but also in children.Citation1–4) Consuming excess amount of “normal” diet daily or frequent ingestion of “energy-dense diets” such as high-fat and high-sugar diet can be considered as overeating. Because of their high palatability, humans and animals ingest high-fat and high-sugar diets beyond the energy requirement.
Various dietary interventions that protect from diet-induced obesity have been tested. Fermentable dietary fibers and indigestible oligosaccharides are known to prevent diet-induced obesity. Anti-obesity effects of resistant maltodextrin and inulin-type fructans including fructooligosaccharides (FOS or OFS) have been demonstrated in animal and human studies.Citation5–11) Fermentable fibers promote production of short chain fatty acids (SCFAs), and SCFAs stimulate secretion and production of gut hormones such as glucagon like peptide-1 (GLP-1) and peptide YY (PYY) in the distal gut. These factors affect glucose, lipid, and energy metabolisms.Citation12) Therefore, it is possible that other fermentable oligosaccharides also exert beneficial effects against diet-induced obesity.
Raffinose (β-D-fructofuranosyl-O-α-D-galactopyranosyl- (1,6)-α-D-glucopyranoside) is an indigestible trisaccharide composed of glucose, fructose, and galactose. Previous studies have demonstrated various effects of dietary raffinose on immunity,Citation13,14) allergy,Citation15,16) and calcium absorption,Citation17), mainly through enhanced gut fermentation. However, it remains unclear whether dietary raffinose has any impact on obesity, glucose, or lipid metabolism.
Difructose anhydride III (di-D-fructofuranosyl 1,2′:2, 3′ anhydride, DFA III) is an indigestible disaccharide composed of two fructose molecules. DFA III is produced from inulin,Citation18) and it promotes intestinal mineral absorptionCitation19–21) and shows prebiotic effects.Citation22,23) Although DFA III lowers cholesterolCitation24) in isoflavone-fed rats and suppresses energy intake in female rats with or without ovariectomy,Citation25) its effect in diet-induced obesity in animal models has not been demonstrated.
In the present study, we first examined the effects of dietary DFA III and raffinose on glycemia and lipid accumulation in rats fed with an obesogenic high-fat and high-sucrose (HFS) diet under isocaloric feeding conditions. The experiment showed that DFA III supplementation resulted in reduced energy intake. Therefore, we further examined the effect of DFA III, as compared with FOS, on energy intake, glycemia, plasma gut hormone concentrations, and cecal fermentation in rats fed the HFS diet supplemented with or without DFA III or FOS, ad libitum.
Materials and methods
Animals and diets
Male Sprague–Dawley rats (5-week-old) were purchased from Japan SLC, Inc. (Shizuoka, Japan), and were fed an American Institute of Nutrition (AIN)-93G-based dietCitation26) for a one-week acclimation period. Each rat was housed in a separate cage and had free access to food and water, except for the days preceding the glucose tolerance test and sacrifice. The experiment was performed in a temperature-controlled room maintained at 22 ± 2 °C with a 12 h light/12 h dark cycle (08:00–20:00 light period). The study was approved by the Hokkaido University Animal Committee, and the animals were handled in accordance with the Hokkaido University guidelines for care and use of laboratory animals. After one week of acclimation, rats were fed individual test-diets, as shown in Table . The HFS diet contained 30% wt/wt fat and 40% wt/wt sucrose.Citation11,27) All diets contained 65 g/kg of fiber. DFA III, raffinose (Raf), and FOS were supplemented in the diet at doses of 30 g/kg diet (3%) by replacing 15 g of cellulose and 15 g of sucrose. The energy density of DFA III, FOS, and Raf was equally estimated at 2 kcal/g as the common value for non-digestible fermentable oligosaccharides, and that of cellulose was estimated at 0 kcal/g, which have been authorized by the Ministry of Health, Labor, and Welfare of Japan.Citation28)
Table 1. Test diet composition.
In experiment 1, rats were divided into four groups (n = 8–10, each group). The control group was fed the control diet ad libitum and other three groups (HFS, H-DFA, and H-Raf) were fed the HFS diet with or without DFA III/raffinose supplementation. In the first 2 days, rats were fed individual test-diets ad libitum. Then, the amount diets were adjusted to have the same energy intake among HFS, H-DFA, and H-Raf groups, based on the energy intake of the previous day. The amounts of test-diets provided to these three groups were adjusted for equal consumption, but not restricted in the lowest-consumption group, throughout the experimental period. After 2, 4, and 6 weeks of feeding the test-diet, tail vein blood was collected to measure fasting (overnight fasted) glucose and insulin concentrations in the plasma. Blood samples were immediately added with heparin (final concentration 50 IU/mL) and aprotinin (final concentration 500 KIU/mL). Plasma was separated by centrifugation at 2300 × g for 10 min at 4 °C and stored at −80 °C. After 8 weeks (Experiment 1) of test-diet feeding and overnight fasting, rats were anesthetized using sodium pentobarbital injection (50 mg/kg of body weight, Somnopentyl injection; Kyoritsu Seiyaku Corporation, Tokyo, Japan). Blood samples were collected from the portal vein using a syringe containing heparin (final concentration 50 IU/mL), aprotinin (final concentration 500 KIU/mL), and DPP-IV inhibitor (final concentration 50 μmol/L; DPP4-010; Merck Millipore). Plasma was collected and stored, as described above. Adipose tissues (mesenteric, epididymal, retroperitoneal), cecal tissue, and cecal content were collected after exsanguination. The weights of the contents were calculated by subtracting tissue weight from total cecal weight. The cecal contents were homogenized with deionized water (300 mg of cecal content in 1.5 mL water). The pH values of homogenates were measured using a compact pH meter (B-212, Horiba, Ltd., Kyoto, Japan).
In experiment 2, rats were divided into six groups (n = 8, each group), and fed the respective test-diet (Table ) ad libitum as follows: control diet, control diet supplemented with 3% DFA III (C-DFA) or 3% FOS (C-FOS), HFS diet, HFS diet supplemented with 3% DFA III (H-DFA) or 3% FOS (H-FOS). After 2 and 4 weeks of test-diet feeding, tail vein blood was collected to measure fasting (overnight fasted) glucose and insulin concentrations in the plasma. Blood samples were treated as described above. After 5 weeks of test-diet feeding and overnight fasting, rats were anesthetized and blood and tissue samples were collected as described above.
Measurement of plasma samples
Glucose and insulin concentrations were measured using a Glucose CII Test Kit (Wako Pure Chemical Industries, Ltd., Osaka, Japan) and a rat insulin enzyme-linked immunosorbent assay (ELISA) kit (AKRIN-010T; Shibayagi Co., Ltd., Gunma, Japan; inter-assay coefficient of variability (CV): < 5%, intra-assay CV: < 5%), respectively. Total GLP-1, active GLP-1, and total PYY concentrations were measured using a multi-species GLP-1 total ELISA kit (EZGLP1T-36 K; Merck Millipore, Darmstadt, Germany; intra-assay CV: < 5%, inter-assay CV: < 12%), a GLP-1 (active) ELISA assay (EGLP-35 K; Merck Millipore; intra-assay CV: 7.4 ± 1.1%, inter-assay CV: 8 ± 4.8%), and a MILLIPLEX MAP rat metabolic hormone magnetic bead panel metabolism multiplex assay (Merck Millipore; intra-assay CV: 3%, inter-assay CV: 11%), respectively. To estimate the degree of insulin resistance, the homeostatic model assessment for insulin resistance (HOMA-IR) was calculated using the following formulaCitation29): HOMA-IR = {glucose (mg/dL) × insulin (μU/mL)}/2,430, where 1 ng of insulin is equivalent to 26 μunits.
Measurement of organic acids in the cecal content
The concentration of organic acids, such as succinate, acetate, propionate, and butyrate in the rat cecal contents were measured using high performance liquid chromatography (HPLC).Citation14) Briefly, the cecal content homogenates were mixed with equal volumes of 0.4 N HCl. After overnight incubation at 4 °C, the supernatant was filtered through a membrane filter and loaded onto an HPLC column (RSpak KC-811; Showa Denko K.K., Tokyo, Japan), and we monitored the wavelength at 445 nm using the post-column method with bromothymol blue as a pH indicator.
Statistical analyses
Data were expressed as means and standard errors (SE) of the mean. Statistical analyses were performed using the JMP Pro version 12.0 software (SAS Institute, Inc., Cary, NC, USA). Statistical significance was assessed using a one-way (Experiment 1) or two-way (Experiment 2) analysis of variance (ANOVA), as appropriate. Significant differences (p < 0.05) between treatments were determined using the Tukey’s HSD test, as appropriate.
Results
Experiment 1
Among the three HFS-fed groups, H-DFA group consumed less amount of diet from the beginning of test-diet feeding. To adjust the energy intake among the three HFS-fed groups, HFS and H-Raf groups were fed reduced amounts of the diet, while H-DFA group was fed ad libitum. As shown in Table , the total energy intake did not differ among the three groups. Notably, the total energy intake of HFS-fed group was not significantly higher than that of the control group which was fed the control diet ad libitum. Final body weights also did not differ significantly between the treatments. Adipose tissue weights were slightly higher in three HFS-fed groups, but not significantly different compared to that of the control group.
Table 2. Body weight, energy intake, and visceral adipose tissue weights of rats fed with test-diets for 8 weeks (Experiment 1).
We monitored fasting glucose and insulin concentrations in the plasma, taken from the tail vein after 2, 4, and 6 weeks of test-diet feeding. However, no significant differences were observed in these parameters between the treatments (data not shown).
The tissue and content weights of cecum (Table ) decreased in the HFS group compared to the control group, but these were maintained or increased by supplementation of DFA III or raffinose. Acetate concentration (μmol/g content) and amount (μmol/cecum) were highest in the H-DFA group, and the amount of acetate was lowest in the HFS group. Similar trends were observed in case of propionate and butyrate concentrations and amounts. Overall, short chain fatty acids productions were relatively reduced in the HFS group, restored or increased in the H-DFA group, and partially restored in the H-Raf group, as compared to the control group. In contrast, succinate concentration was highest in the HFS group.
Table 3. Cecal tissue, content weights, pH, and short chain fatty acid concentrations of rats fed test-diets for 8 weeks (Experiment 1).
Total GLP-1 concentrations (Fig. ) in the portal plasma after 8 weeks of test-diet feeding were measured. The GLP-1 concentration in the HFS group tended to be lower than that in the control group. In contrast, GLP-1 concentrations in H-DFA and H-Raf groups did not decrease, as compared to the control group. Moreover, concentration in the H-Raf group was significantly higher than that in the HFS group.
Fig. 1. GLP-1 concentration in the portal plasma after 8 weeks of test-diet feeding (Experiment 1).
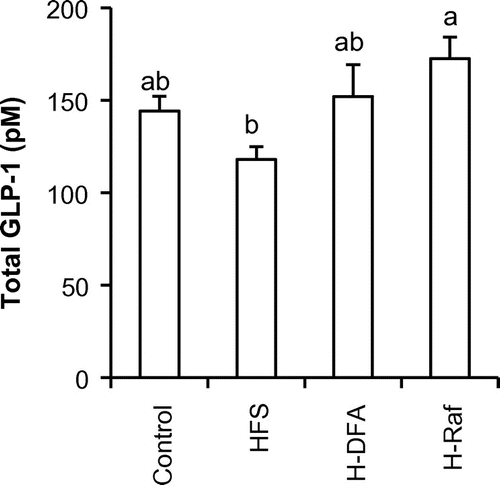
Although supplementation of DFA III or Raf to the HFS diet did not clearly affect adiposity and glucose tolerance under isocaloric feeding condition, DFA III showed suppressive effect on energy intake. In experiment 2, we examined effects of supplementing DFA III and FOS to the HFS diet and to the control diet under ad libitum feeding condition on energy intake, adiposity, glucose tolerance, cecal fermentation, and gut hormones involved in satiety induction.
Experiment 2
Significant effects (increments) of the HFS diet were detected using the two-way ANOVA on body weight, weigh gain, and energy intake (Table ). Significant effects of fiber on body weight gain and total energy intake were also detected, as rats fed DFA III-supplemented diets showed lowest values compared with rats fed cellulose- or FOS-containing diets (both control and HFS diets). Adipose tissue weights also increased by the HFS diet, but rats fed DFA III-supplemented diets showed lower epididymal fat weights than rats fed cellulose- or FOS-containing diets. Significant effect of fiber was detected using the two-way ANOVA.
Table 4. Body weight, energy intake, and adipose tissue weights of rats fed the control or the HFS diet supplemented with DFA III or FOS for 5 weeks (Experiment 2).
Fig. shows daily changes in the difference in cumulative energy intake of test groups compared with that in the control group fed the control diet containing only cellulose as dietary fiber. The result clearly demonstrates that rats fed with the HFS diet had larger energy intake from beginning of the test period, and the cumulative difference continuously increased throughout the experimental period. H-FOS group showed incremental changes, similar to the HFS group, while H-DFA group showed only slight increment until end of the experiment. The C-DFA group had lower energy intake than the control group throughout the experiment.
Fig. 2. Differences in cumulative energy intake compared to energy intake of the control group (Experiment 2).
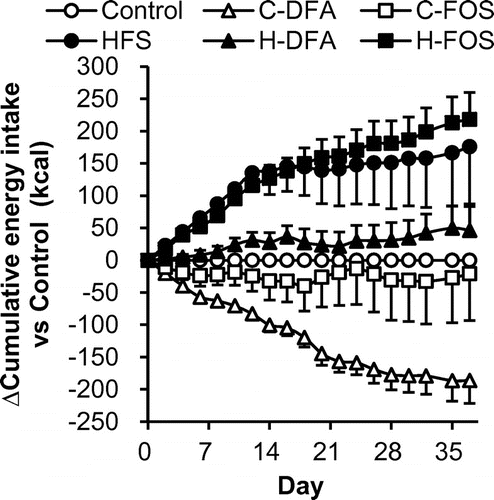
We monitored fasting glucose and insulin levels to assess glucose tolerance after 2 and 4 weeks of test-diet feeding (Fig. ). Significant effects were not detected using the two-way ANOVA, but HFS diet showed a tendency of increased glucose level after 2 weeks (p = 0.053). Plasma GLP-1 levels were significantly affected by replacing the fiber (p = 0.034 and 0.001, after 2 and 4 weeks, respectively). After 4 weeks, rats fed DFA-supplemented diets had highest GLP-1 levels among all treatments. When compared within the three HFS diet-fed groups, plasma GLP-1 concentrations in the H-DFA rats were significantly higher than those in the HFS-fed rats (Tukey’s test, p < 0.05).
Fig. 3. Plasma glucose, insulin, GLP-1 concentrations, and HOMA-IR under fasting condition 2 and 4 weeks after test-diet feeding (Experiment 2).
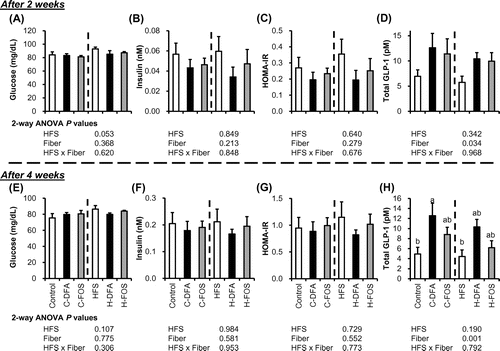
After 5 weeks of test-diet feeding, we collected portal plasma under anesthesia. Total GLP-1 and active GLP-1 concentrations in the portal plasma (Fig. ) were significantly affected by fiber (two-way ANOVA). Active and total GLP-1 concentrations in C-DFA and C-FOS groups were significantly higher than those in the control group, and GLP-1 concentrations in the H-DFA and H-FOS groups had higher tendency than those in the HFS group. The concentration of PYY, another L-cell-derived hormone, in the portal plasma of the C-DFA and C-FOS groups were significantly higher than that in the control group. Although the HFS diet significantly elevated PYY levels (p < 0.0001, two-way ANOVA), supplementing DFA III further increased plasma PYY concentration as it is significantly higher than that in HFS group.
Fig. 4. GLP-1 and PYY concentrations in the portal plasma of rats fed the control or the HFS diet supplemented with DFA III or FOS for 5 weeks (Experiment 2).
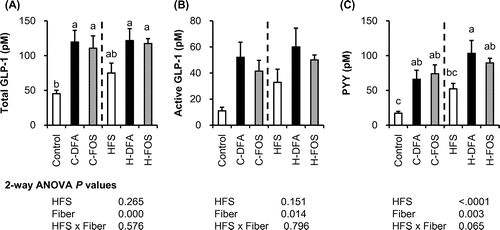
Cecal content weight (Table ) increased significantly in the C-DFA group, as compared to the control group, while the H-DFA group showed insignificant increments, compared to the HFS group, suggesting suppressive effect of the HFS diet on the DFA III-enhanced cecal fermentation. This is supported by lower concentrations of acetate and propionate in the H-DFA group compared to the C-DFA group. However, when compared within the three HFS-fed groups, acetate and propionate concentrations in the H-DFA group, but not in the H-FOS group, were significantly higher than those in the HFS group (p < 0.05, Tukey’s test). Succinate concentration was more than threefold higher in the HFS group compared to that in the control group, while H-DFA and H-FOS had modestly increased concentrations compared to the control group.
Table 5. Cecal tissue, content weights, pH, and short chain fatty acid concentrations of rats fed the control or the HFS diet supplemented with DFA III or FOS for 5 weeks (Experiment 2).
Discussion
The present study aimed to investigate the effects of indigestible oligosaccharides on development of diet-induced obesity. FOS is known to exert anti-obesity or anti-hyperglycemic effects, but effects of DFA III and Raf on diet-induced obesity remained unclear. In the first experiment comparing the effects of DFA III and Raf under isocaloric feeding conditions, DFA III seemed to prevent excess energy intake. The anorexigenic effect of DFA III was reproduced in the second experiment under ad libitum feeding conditions. FOS or Raf did not reduce the energy intake at identical doses (3% wt in the diet). Rats fed DFA III showed highest acetate content in the cecum and increased plasma GLP-1 and PYY concentrations compared to rats fed the diet containing only cellulose as fiber. These results suggest anti-obesity effect of dietary DFA III as one of fermentable oligosaccharides with relatively higher potency than FOS and raffinose.
In experiment 1, except for the control group, rats were fed the test-diets (HFS, H-DFA, H-Raf) under isocaloric conditions to examine the effects of DFA III and raffinose on adiposity and glucose metabolism, independent of energy intake. The results show no apparent effects of DFA III and raffinose on parameters related to adiposity and glucose metabolism. However, energy intake was lower in the H-DFA group than in the HFS and H-Raf groups, when rats were fed the diet ad libitum at the beginning of experimental period. The HFS group did not show significant increment in body weight, adipose tissue weights, and glycemia, compared to the control group because of adjustment of energy intake among the three HFS-fed groups. These results suggest that DFA III has preventive effect against diet-induced obesity and glucose intolerance through reduced consumption of the high-energy diet.
Experiment 2 was conducted to examine the effects of DFA III and FOS on energy intake and on the development of diet-induced obesity under ad libitum feeding conditions. Supplementation of DFA III reduced the energy intake when it was added either in the control diet or the HFS diet, while FOS had no effects on the energy intake. Accordingly, body weight gain and epididymal fat weight were also reduced by feeding DFA III-supplemented diets (C-DFA and H-DFA). As a result, the H-DFA group showed almost similar values of body weight, adipose tissue weight, and fasting glucose concentrations as those of the control group. FOS and inulin-type fructans have been reported to increase SCFA production, increase GLP-1 production, and decrease energy intake in rodent models, on adding these oligosaccharides in the diet at around 5–10% (wt/wt).Citation5–9,30–32) The dose of FOS at 3% in the present study seems insufficient to exert an anorexic effect in the diet-induced obesity model. In contrast, DFA III at 3% in the diet is effective in preventing excess energy intake. Although DFA III reduced energy intake when it was added to the control diet, the growth of rats was not impaired in the present study. The relatively higher efficacy of DFA III than FOS and raffinose on appetite suppression against the palatable obesogenic diet indicates the translational potential of DFA III in preventing excess energy intake in humans at a low dose and with low risk of unfavorable effects such as hyperosmotic diarrhea. In a human study, repeated ingestion of DFA III at 5 g/day for 12 days did not show any harmful side effects.Citation33)
Cecal SCFA (acetate, propionate, butyrate) contents (μmol/cecum) decreased after continuous feeding with HFS diet in both of the experiments. SCFA contents were restored overall by supplementing with DFA III, raffinose, and FOS, although these oligosaccharides had different impacts on individual SCFA levels. Acetate contents were increased by DFA III in both experiments, while raffinose and FOS had relatively smaller impact on the acetate production. These results indicate that oligosaccharides are utilized to produce SCFAs in the cecum, even if they are supplemented in the HFS diet that suppresses gut fermentation.
SCFAs are known to promote secretion and production of GLP-1 in the large intestine.Citation34,35) Elevated plasma GLP-1 levels on supplementation of DFA III, raffinose, and FOS were demonstrated in the present study. Although changes in SCFA production and plasma GLP-1 levels are not simply parallel among all the diet-treatments, increased SCFAs probably enhanced GLP-1 release in the large intestine. GLP-1 has an anorexic effect, along with an incretin effect.Citation36) Moreover, acetate also shows an anorexic effect.Citation37,38) Acetate production was largely increased by DFA III in the present study. A previous study showed similar effects of DFA III, FOS, and raffinose on cecal SCFA production in rats,Citation39) while several following studies demonstrated higher impacts of DFA III in rats on cecal SCFA production than FOS, especially on acetate content.Citation40–42) Although experimental conditions vary among studies, the latter studies support the finding that DFA III has potent effect on gut fermentation compared to FOS. Thus, the suppressive effect of DFA III on energy intake could be attributed to combined effects of increased acetate and GLP-1 levels. Plasma GLP-1 concentrations increased rapidly (within 2 weeks) by DFA III and FOS, and the effect sustained by end of the experimental period, suggesting that cecal fermentation was already modified after 2 weeks of feeding these oligosaccharides. Another anorexic gut hormone, PYY,Citation43) was significantly elevated by DFA III, but not by FOS, when added to the HFS diet. These results suggest that additive or synergistic effects of increased plasma GLP-1, PYY, and acetate are involved in suppressive effects of DFA III on excess energy intake. There may be other mechanisms also involved. Although DFA III failed to stimulate GLP-1 secretion from a murine GLP-1-producing enteroendocrine cell line (GLUTag) in our preliminary study (data not shown), postprandial GLP-1/PYY secretions might be transiently potentiated by the supplementation of DFA III to the diet, through direct/indirect action of DFA III on enteroendocrine L cells in vivo. Further studies would be need in the future to assess the hypothesis.
Interestingly, increased production of succinate by the HFS diet was attenuated by supplementation of DFA-III, raffinose, and FOS. Luminal succinate has been reported to have unfavorable effects such as inhibition of colonic epithelial proliferation, inflammatory, and hypoxic effects.Citation44–46) Therefore, these oligosaccharides may protect the host from any harmful effects by reducing succinate production. Clarifying the underlying mechanism will be an attractive issue for future studies.
In summary, rats were fed the HFS diet with or without supplementation of DFA III, raffinose, and FOS for 5 or 8 weeks. Rats fed DFA III-containing diet showed lower energy intake compared to rats fed with the HFS diet, resulting in reduced fat accumulation in DFA III-fed rats. Cecal SCFA productions were increased by these oligosaccharides, but acetate production was largely increased by DFA III. Plasma GLP-1 concentrations were elevated in DFA III and FOS-fed groups after 2 weeks of the diet intervention. In addition, plasma PYY concentration was increased in the DFA III fed group. These results demonstrate that DFA III has suppressive effect on energy intake against palatable obesogenic diet, possibly through combined effects of increased anorexigenic factors, including cecal acetate, plasma GLP-1, and PYY concentrations.
Disclosure statement
Keisuke Takahashi, Taizo Nagura, and Hirokatsu Uchino are employee of Nippon Beet Sugar Mfg., Co., Ltd.
Funding
This work was supported by the Japan Society for the Promotion of Science [grant number JP16K07725], [grant number 16K14918].
References
- English L, Lasschuijt M, Keller KL. Mechanisms of the portion size effect. What is known and where do we go from here? Appetite. 2015;88:39–49.
- Livingstone MB, Pourshahidi LK. Portion size and obesity. Adv Nutr. 2014;5(6):829–834.
- Tulloch AJ, Murray S, Vaicekonyte R, et al. Neural responses to macronutrients: hedonic and homeostatic mechanisms. Gastroenterology. 2015;148(6):1205–1218.
- Zhang Y, Liu J, Yao J, et al. Obesity: pathophysiology and intervention. Nutrients. 2014;6(11):5153–5183.
- Cani PD, Dewever C, Delzenne NM. Inulin-type fructans modulate gastrointestinal peptides involved in appetite regulation (glucagon-like peptide-1 and ghrelin) in rats. Br J Nutr. 2004;92(3):521–526.
- Cani PD, Daubioul CA, Reusens B, et al. Involvement of endogenous glucagon-like peptide-1(7-36) amide on glycaemia-lowering effect of oligofructose in streptozotocin-treated rats. J Endocrinol. 2005;185(3):457–465.
- Delzenne NM, Cani PD, Daubioul C, et al. Impact of inulin and oligofructose on gastrointestinal peptides. Br J Nutr. 2005;93(Suppl 1):S157–161.
- Reimer RA, Russell JC. Glucose tolerance, lipids, and GLP-1 secretion in JCR:LA-cp rats fed a high protein fiber diet. Obesity (Silver Spring). 2008;16(1):40–46.
- Zhou J, Martin RJ, Tulley RT, et al. Dietary resistant starch upregulates total GLP-1 and PYY in a sustained day-long manner through fermentation in rodents. Am J Physiol Endocrinol Metab. 2008;295(5):E1160–E1166.
- Hashizume C, Kishimoto Y, Kanahori S, et al. Improvement effect of resistant maltodextrin in humans with metabolic syndrome by continuous administration. J Nutr Sci Vitaminol (Tokyo). 2012;58(6):423–430.
- Hira T, Suto R, Kishimoto Y, et al. Resistant maltodextrin or fructooligosaccharides promotes GLP-1 production in male rats fed a high-fat and high-sucrose diet, and partially reduces energy intake and adiposity. Eur J Nutr. Forthcoming. doi:10.1007/s00394-017-1381-7.
- van de Wouw M, Schellekens H, Dinan TG, et al. Microbiota-gut-brain axis: modulator of host metabolism and appetite. J Nutr. 2017;147(5):727–745.
- Nagura T, Hachimura S, Hashiguchi M, et al. Suppressive effect of dietary raffinose on T-helper 2 cell-mediated immunity. Br J Nutr. 2002;88(4):421–426.
- Nagura T, Hachimura S, Kaminogawa S, et al. Characteristic intestinal microflora of specific pathogen-free mice bred in two different colonies and their influence on postnatal murine immunocyte profiles. Exp Anim. 2005;54(2):143–148.
- Watanabe H, Sonoyama K, Watanabe J, et al. Reduction of allergic airway eosinophilia by dietary raffinose in Brown Norway rats. Br J Nutr. 2004;92(2):247–255.
- Sonoyama K, Watanabe H, Watanabe J, et al. Allergic airway eosinophilia is suppressed in ovalbumin-sensitized Brown Norway rats fed raffinose and alpha-linked galactooligosaccharide. J Nutr. 2005;135(3):538–543.
- Mitamura R, Hara H, Aoyama Y. Ingestion of raffinose promotes calcium absorption in the large intestine of rats. Biosci Biotechnol Biochem. 2004;68(2):384–389.
- Saito K, Tomita F. Difructose anhydrides: their mass-production and physiological functions. Biosci Biotechnol Biochem. 2000;64(7):1321–1327.
- Suzuki T, Hara H. Difructose anhydride III and sodium caprate activate paracellular transport via different intracellular events in Caco-2 cells. Life Sci. 2006;79(4):401–410.
- Tomita K, Shiomi T, Okuhara Y, et al. Ingestion of difructose anhydride III enhances absorption and retention of calcium in healthy men. Biosci Biotechnol Biochem. 2007;71(3):681–687.
- Hara H, Onoshima S, Nakagawa C. Difructose anhydride III promotes iron absorption in the rat large intestine. Nutrition. 2010;26(1):120–127.
- Minamida K, Asakawa C, Sujaya IN, et al. Effects of long-term ingestion of difructose anhydride III (DFA III) on intestinal bacteria and bile acid metabolism in humans. J Biosci Bioeng. 2006;101(2):149–156.
- Minamida K, Ohashi M, Hara H, et al. Effects of ingestion of difructose anhydride III (DFA III) and the DFA III-assimilating bacterium Ruminococcus productus on rat intestine. Biosci Biotechnol Biochem. 2006;70(2):332–339.
- Tamura A, Nishimukai M, Shigematsu N, et al. Supplementation of difructose anhydride III enhanced elevation of plasma equol concentrations and lowered plasma total cholesterol in isoflavone-fed rats. Br J Nutr. 2006;96(3):442–449.
- Fujitani M, Kishida T, Shimizu E, et al. Difructose anhydride III decreases body fat as a low-energy substitute or by decreasing energy intake in non-ovariectomized and ovariectomized female rats. Biosci Biotechnol Biochem. 2017;81(7):1425–1432.
- Reeves PG. Components of the AIN-93 diets as improvements in the AIN-76A diet. J Nutr. 1997;127(5 Suppl):838S–841S.
- Nakajima S, Hira T, Hara H. Postprandial glucagon-like peptide-1 secretion is increased during the progression of glucose intolerance and obesity in high-fat/high-sucrose diet-fed rats. Br J Nutr. 2015;113(9):1477–1488.
- Oku T, Nakamura S. Digestion, absorption, fermentation, and metabolism of functional sugar substitutes and their available energy. Pure Appl Chem. 2002;74(7):1253–1261.
- Cacho J, Sevillano J, de Castro J, et al. Validation of simple indexes to assess insulin sensitivity during pregnancy in Wistar and Sprague-Dawley rats. Am J Physiol Endocrinol Metab. 2008;295(5):E1269–E1276.
- den Besten G, Gerding A, van Dijk TH, et al. Protection against the metabolic syndrome by Guar gum-derived short-chain fatty acids depends on peroxisome proliferator-activated receptor γ and glucagon-like peptide-1. PLoS One. 2015;10(8):e0136364. doi:10.1371/journal.pone.0136364.
- Adam CL, Williams PA, Dalby MJ, et al. Different types of soluble fermentable dietary fibre decrease food intake, body weight gain and adiposity in young adult male rats. Nutr Metab (Lond). 2014;11:36. doi:10.1186/1743-7075-11-36.
- Adam CL, Gratz SW, Peinado DI, et al. Effects of dietary fibre (Pectin) and/or increased protein (Casein or Pea) on satiety, body weight, adiposity and Caecal fermentation in high fat diet-induced obese rats. PLoS one. 2016;11(5):e0155871. doi:10.1371/journal.pone.0155871.
- Tamura A, Shiomi T, Tamaki N, et al. Comparative effect of repeated ingestion of difructose anhydride III and palatinose on the induction of gastrointestinal symptoms in humans. Biosci Biotechnol Biochem. 2004;68(9):1882–1887.
- Psichas A, Sleeth ML, Murphy KG, et al. The short chain fatty acid propionate stimulates GLP-1 and PYY secretion via free fatty acid receptor 2 in rodents. Int J Obes (Lond). 2015;39(3):424–429.
- Tolhurst G, Heffron H, Lam YS, et al. Short-chain fatty acids stimulate glucagon-like peptide-1 secretion via the G-protein-coupled receptor FFAR2. Diabetes. 2012 Feb;61(2):364–371.
- Holst JJ. Incretin hormones and the satiation signal. Int J Obes (Lond). 2013;37(9):1161–1168.
- Frost G, Sleeth ML, Sahuri-Arisoylu M, et al. The short-chain fatty acid acetate reduces appetite via a central homeostatic mechanism. Nat Commun. 2014;5:3611. doi:10.1038/ncomms4611.
- Chambers ES, Morrison DJ, Frost G. Control of appetite and energy intake by SCFA: what are the potential underlying mechanisms? Proc Nutr Soc. 2015;74(3):328–336.
- Suzuki T, Hara H, Kasai T, et al. Effects of difructose anhydride III on calcium absorption in small and large intestines of rats. Biosci Biotechnol Biochem. 1998;62(5):837–841.
- Afsana K, Shiga K, Ishizuka S, et al. Ingestion of an indigestible saccharide, difructose anhydride III, partially prevents the tannic acid-induced suppression of iron absorption in rats. J Nutr. 2003;133(11):3553–3560.
- Asvarujanon P, Ishizuka S, Hara H. Promotive effects of non-digestible disaccharides on rat mineral absorption depend on the type of saccharide. Nutrition. 2005;21(10):1025–1035.
- Shiga K, Nishimukai M, Tomita F, et al. Ingestion of difructose anhydride III, a non-digestible disaccharide, prevents gastrectomy-induced iron malabsorption and anemia in rats. Nutrition. 2006;22(7–8):786–793.
- Price SL, Bloom SR. Protein PYY and its role in metabolism. Front Horm Res. 2014;42:147–154.
- Inagaki A, Ichikawa H, Sakata T. Inhibitory effect of succinic acid on epithelial cell proliferation of colonic mucosa in rats. J Nutr Sci Vitaminol (Tokyo). 2007;53(4):377–379.
- Jakobsdottir G, Xu J, Molin G, et al. High-fat diet reduces the formation of butyrate, but increases succinate, inflammation, liver fat and cholesterol in rats, while dietary fibre counteracts these effects. PLoS one. 2013;8(11):e80476.
- Yang Y, Sitanggang NV, Okazaki Y, et al. Supplemental fermented plant product (‘Manda Koso’) reduces succinate and deoxycholate, as well as elevates IgA and mucin levels, in rats fed a high-fat diet. Biomed Rep. 2015;3(6):787–791.