Abstract
We previously reported that galectin-9 (Gal-9), an immunomodulatory animal lectin, could bind to insoluble collagen preparations and exerted direct cytocidal effects on immune cells. In the present study, we found that mature insoluble elastin is capable of binding Gal-9 and other members of the human galectin family. Lectin blot analysis of a series of commercial water-soluble elastin preparations, PES-(A) ~ PES-(E), revealed that only PES-(E) contained substances recognized by Gal-9. Gal-9-interacting substances in PES-(E) were affinity-purified, digested with trypsin and then analyzed by reversed-phase HPLC. Peptide fragments derived from five members of the small leucine-rich repeat proteoglycan family, versican, lumican, osteoglycin/mimecan, prolargin, and fibromodulin, were identified by N-terminal amino acid sequence analysis. The results indicate that Gal-9 and possibly other galectins recognize glycans attached to small leucine-rich repeat proteoglycans associated with insoluble elastin and also indicate the possibility that mature insoluble elastin serves as an extracellular reservoir for galectins.
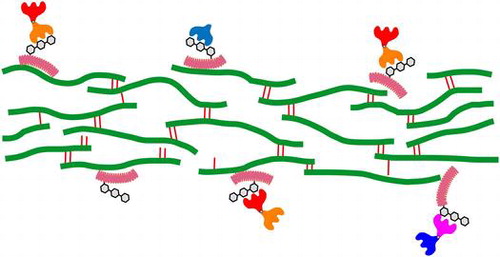
The members of galectin family, especially galectin-9, recognize small leucine-rich repeat.
Recent studies have established important intracellular roles of galectins, a family of soluble animal lectins with affinity for β-galactosides.Citation1–3) In addition to their intracellular functions, galectins have long been assumed to act extracellularly through interactions with cell membrane glycoconjugates. Although galectins lack a typical secretion signal sequence, several lines of evidence suggest that they are secreted via a non-classical secretory pathway and act in an autocrine/paracrine manner.Citation4) In addition, some galectins are known to bind extracellular matrix (ECM) components and modulate cellular functions: Gal-1 and Gal-3 bound to fibronectin and laminin affect adhesion, motility and proliferation of various types of cells.Citation5,6) Matrigel-bound Gal-1 is able to directly kill susceptible T cells more efficiently than soluble Gal-1.Citation7) Gal-3 exhibits affinity not only for fibronectin and laminin but also collagen,Citation8) tenascin,Citation9) and hensin.Citation10) Gal-3-dependent polymerization of hensin is required for the terminal differentiation of epithelial cells. Gal-7, -8, and -9 are known to modulate cell-ECM interactions, which results in maintenance of epidermal homeostasis,Citation11) modulation of cell adhesion/migration,Citation12) and inhibition of tumor metastasis.Citation13) These findings suggest that ECM-galectin interactions are important and sometimes indispensable for the extracellular functions of galectins.
We previously reported that Gal-9 exhibited lactose-sensitive binding activity toward collagen vitrigel membranes and insoluble collagen fibrils.Citation14) Gal-9-modified matrices could induce cell death in human immune cell lines, and the effect was dependent on direct cell-to-membrane contact. In the present study, we found that Gal-9 and other members of the galectin family exhibit affinity for mature insoluble elastin, another major component of ECM. In addition, analysis of water-soluble elastin prepared from insoluble elastin by organic acid hydrolysisCitation15) revealed that several members of the small leucine-rich repeat proteoglycan (SLRP) family incorporated into insoluble elastic fibers provide binding sites for Gal-9.
Materials and methods
Cloning and sequence analysis of porcine elastin and osteoglycin/mimecan cDNAs
Porcine skin total RNA (Zyagen, San Diego, CA, USA) and uterus total RNA (Unitech Co., Ltd., Chiba, Japan) were used to amplify cDNAs for elastin and osteoglycin, respectively. About 2 μg of total RNA was reverse transcribed using a GeneAmp RNA PCR kit with oligo(dT) primers (Perkin-Elmer, Norwalk, CT, USA). cDNAs for elastin and osteoglycin were amplified using gene-specific primer pairs ELN-1/-2 and OGN-1/-2, respectively.
ELN-1: 5’-CTCCCCGAGATGGCGGGTCTGA-3’
ELN-2: 5’-TCACTTTCTCTTCCGGCCACAGGA-3’
OGN-1: 5’-TTGTCCCACAGTCAGCAGGCCACT-3’
OGN-2: 5’-GAGACAGATTATAAGTATGTGTGTACTT-3’
The amplified cDNAs were cloned into the pGEM-T Easy vector using a TA cloning system (Promega Corp., Madison, WI, USA). The DNA sequence was determined using a BigDye Terminator Cycle Sequencing Kit (Applied Biosystems, Foster City, CA, USA) and an ABI Prism 3130 Genetic Analyzer (Applied Biosystems). The sequences were deposited in the DDBJ database under the accession numbers LC005979 (elastin, long form), LC005980 (elastin, short form), and LC060063 (osteoglycin). All the partial amino acid sequences of porcine elastin reported previouslyCitation16) were found in the amino acid sequence translated from LC005979. The sequences determined in the present study were used to interpret the results given in the text.
Expression and purification of recombinant proteins
Expression of tag-free recombinant proteins, a protease-resistant form of human Gal-9 (G9Null) and other members of human galectin family except for Gal-4, in Escherichia coli BL21(DE3) was carried out as described previously.Citation17) Recombinant proteins were purified by affinity chromatography on a lactose-agarose column (Seikagaku Corp., Tokyo, Japan). Gal-4 was expressed as a glutathione S-transferase (GST)-fusion protein and purified by affinity chromatography on a glutathione-Sepharose column (GE Healthcare, Piscataway, NJ, USA). The GST tag was removed by thrombin cleavage. The purified proteins were dialyzed against PBS and then sterilized by filtration. The protein concentration was determined using BCA protein assay reagent (Pierce, Rockford, IL, USA) and bovine serum albumin as a standard. SDS-polyacrylamide gel electrophoresis (SDS-PAGE) patterns of purified recombinant galectins are shown in supplementary Fig. S1.
Elastin binding assay
Elastin-binding activity was assayed using porcine aorta insoluble elastin (PEI; Extra Cellular Matrix Laboratories, Mie, Japan), as described previously.Citation14) Insoluble elastin placed in the inner filter cup of a Nanosep MF centrifugal device (10 mg/cup) was washed twice with 0.3 mL of Tris-buffered saline (10 mM Tris-HCl, pH 7.5, 0.15 M NaCl, TBS) (10 min × 2) before use. Galectins dissolved in TBS were added to the cup (240 μL/cup), followed by incubation for 1 h. Protein solutions of at least five different concentrations (2.5, 5.0, 7.5, 10.0, and 12.5 μM) were used for each galectin. Bovine serum albumin (12.5 μM) was used as a control protein. After incubation, the device was centrifuged to obtain a filtrate containing the unbound protein. The filtrate and the original protein solution were subjected to SDS-PAGE (see below) together with a series of different amounts of the protein as quantitation standards. After electrophoresis, the gels were stained with Coomassie brilliant blue R-250. The stained gels were then scanned and quantitated using ImageJ software.Citation18) Extraction of elastin with SDS and 2-mercaptoethanol was carried out as follows. Insoluble elastin was washed twice with 0.3 mL of TBS as described above. TBS containing 2% SDS and 5% 2-mercaptoethanol was added to the washed elastin (0.3 mL/cup), followed by incubation at 60 °C for 10 min. After being cooled to room temperature, the device was centrifuged to remove the extraction solution, followed by washing twice with TBS. The extracted elastin was incubated with TBS, 1% Triton X-100 (0.3 mL/cup) for 30 min at room temperature to remove residual SDS. After washing three times with TBS, the extracted elastin was used for the binding assay.
SDS-PAGE and lectin blot analysis
Samples and standards (10 μL/lane) were electrophoretically separated in SDS/12.6% polyacrylamide gels under reducing conditions. The gels were stained with Coomassie Brilliant Blue R-250. Two protein ladder markers (P7701 and P7703, New England Biolabs, Inc., Beverly, MA, USA) were used in this study. Lectin blot analysis was carried out as described by Matsumura et al.Citation19) with modifications. Five water-soluble elastin preparations, PES-(A) ~ PES-(E), and biotinylated concanavalin A were purchase from Extra Cellular Matrix Laboratories and J-oil Mills (Tokyo, Japan), respectively. G9Null was biotinylated using EZ-Link NHS-Biotin (Pierce) according to the manufactures instructions. Samples were electrophoretically separated in SDS/12.6% polyacrylamide gels and then transferred to polyvinylidene difluoride membranes (Millipore, Billerica, MA, USA). The membranes were incubated with 2% bovine serum albumin in TBS for 1 h, and then overnight with 1 μg/mL of biotinylated G9Null in TBS-T (TBS containing 0.05% Tween 20). When biotinylated concanavalin A (0.5 μg/mL) was used as a lectin probe, the membranes were blocked with Carbo-Free Blocking Solution (Vector Laboratories, Burlingame, CA, USA). After washing with TBS-T, the membranes were incubated with streptavidin-biotinylated horseradish peroxidase complex (GE Healthcare) for 1 h, and then washed with TBS-T. Chemiluminescent detection was carried out using ECL reagent (GE Healthcare).
Isolation of Gal-9-interacting substances by affinity chromatography and RP-HPLC
PES-(E) was reduced and pyridylethylated by the method of Fullmer.Citation20) About 20 mg of pyridylethylated PES-(E), PES-(E)·PE, dissolved in 3 mL of TBS was subjected to affinity purification on a G9Null-immobilized column (about 3 mg of G9Null immobilized on a 1-mL HiTrap NHS-activated HP column; GE Healthcare). The column was washed with 5 mL of TBS. G9Null-interacting substances were eluted with TBS, 0.2 M lactose. The eluate, PES-(E)·PE·AF, was acidified with TFA, and then applied to a μBondasphere C18 column (150 × 3.9 mm; Waters, Milford, MA, USA). After washing with 0.1% TFA, the column was developed with a linear gradient of 0–80% (v/v) acetonitrile in 0.1% (v/v) TFA. The eluate was fractionated into 13 fractions, which were then combined into three pools (designated as AF46, AF79 and AF1012) based on the result of lectin blot analysis with biotinylated G9Null as the probe.
Trypsin digestion of AF46, AF79, and AF1012, and subsequent purification of tryptic peptides by RP-HPLC
Lyophilized AF46, AF79, and AF1012 were individually dissolved in 3 mL of 50 mM Tris-HCl (pH 8.5), 1 mM CaCl2. After addition of 30 μg of trypsin (Roche Diagnostics, Mannheim, Germany), the reaction mixture was incubated for 16 h at 37 °C. The tryptic digests were acidified and then subjected to RP-HPLC as described above. Peak fractions were further purified by rechromatography under the same conditions.
Results and discussion
Elastin-binding activity of Gal-9 and other members of the galectin family
The elastin-binding activity of galectins was determined using a porcine insoluble elastin preparation. Initially, we attempted to analyze the binding data by means of Scatchard plots. However, a typical Scatchard plot was not obtained for CSGal-1 (a cysteine-less mutant of Gal-1),Citation21) Gal-3, Gal-4, Gal-7, or G9Null (a protease-resistant form of human Gal-9 with a truncated linker peptide)Citation17) under the experimental conditions used. This was probably due to the presence of multiple binding/interaction sites in the elastin preparation. Therefore, the binding data were plotted as “Bound vs. Free” not “Bound/Free vs. Bound” as in the case of the collagen-binding activity of Gal-9 reported previously.Citation14) The results indicate that the apparent binding affinities of galectins for insoluble elastin are in the following order: G9Null > Gal-8 > Gal-4 > Gal-3 >> CSGal-1 > Gal-7, although quantitative comparison is not possible, because Kd values are not available for all galectins (Fig. (A)). In the case of Gal-8, the apparent Kd and Bmax values were determined to be 3.81 × 10−6 M and 0.295 nmoles/mg elastin, respectively, by Scatchard analysis. To determine whether or not the elastin preparation contains contaminating substances that show affinity for G9Null, insoluble elastin was extracted with 2% SDS plus 5% 2-mercaptoethanol and then used for the assaying. G9Null showed slightly higher binding activity toward the extracted elastin than the untreated form (Fig. (B)). The results suggest that G9Null and probably other galectins directly bind to insoluble elastin and/or substance(s) associated with elastin via covalent bonds except for disulfide bonds. It is probable that the treatment with SDS and 2-mercaptoethanol removed minor contaminants, which interfere with Gal-9 binding, from the insoluble elastin preparation.
Fig. 1. Elastin-binding activities of galectins.
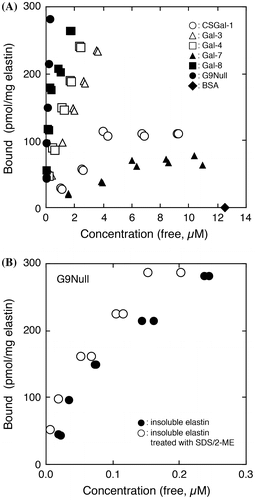
Among the members of the galectin family, Gal-3 has been shown to interact with elastin.Citation22) The authors suggested that Gal-3 directly interacted with peptide domain of elastin in a novel fashion based on experiments using hydrazine-treated elastin preparation. They also demonstrated that endogenous Gal-3 in a breast carcinoma cell line was associated with tropoelastin. Although the present study indicates that G9Null interacts with insoluble elastin in a glycan-dependent manner (see below), involvement of protein–protein interactions cannot be ruled out.
Affinity purification of Gal-9-interacting substances from a water-soluble elastin preparation
In order to identify the Gal-9-interacting entity in mature insoluble elastin, five commercial water-soluble elastin preparations prepared by oxalic acid hydrolysis of insoluble elastin, PES-(A/B/C/D/E), were tested for the presence of Gal-9-interacting substance(s). All the water-soluble elastin preparations retained coacervation ability, an intrinsic property of elastin, with ascending coacervation temperature (at 1% concentration): 20–22.5 °C, 22.5–25 °C, 25–30 °C, 30–35 °C, and 35–50 °C for PES-(A) ~ PES-(E), respectively (Extra Cellular Matrix Laboratories, https://www.ecm-labo.co.jp/product/pdf/1503-shiyaku-price.pdf). SDS-PAGE patterns of PES-(A) ~ PES-(E) are shown in supplementary Fig. S2. Lectin blot analysis of the elastin preparations using biotinylated G9Null as a probe revealed that only PES-(E), which consisted of peptide fragments released at the earliest stage of acid hydrolysis of insoluble elastin, contained substances recognized by G9Null (Fig. (A)). On the other hand, concanavalin A, a plant lectin with preferential specificity for high-mannose type N-glycans, detected multiple bands in whole cells lysates of PC-3 and Jurkat cells but did not react with any of the water-soluble elastin preparations (Fig. (B)). The results indicate that high-mannose type N-glycans are scarcely present in the water-soluble elastin preparations.
Fig. 2. Lectin blot analysis.
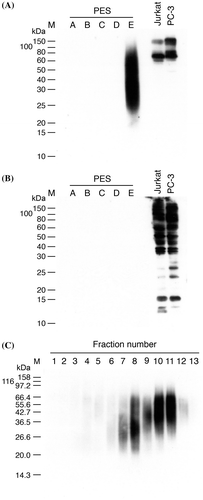
PES-(E) was pyridylethylated and then subjected to affinity chromatography using G9Null immobilized on a NHS-activated HiTrap Sepharose column. About 4.5% (4.5 ± 1.0, n = 5) of the input material was recovered in the lactose eluate (affinity-purified fraction), PES-(E)·PE·AF, as judged from the absorbance at 280 nm. Gal-9-interacting substances were detected only in PES-(E)·PE·AF, i.e. not in the flow-through fraction by lectin blot analysis (data not shown). PES-(E)·PE·AF was further fractionated by reversed-phase HPLC (RP-HPLC) on a C18 column. PES-(E)·PE·AF was eluted as two broad peaks and the eluate was fractionated into thirteen fractions (AF1 ~ AF13) (supplementary Fig. S3). Lectin blot analysis of the RP-HPLC fractions showed that Gal-9-interacting substances were distributed mainly in AF7 ~ AF12 (Fig. (C)). AF4 ~ AF6 also contained lower amounts of Gal-9-interacting substances (see supplementary Fig. S4).
Identification of Gal-9-interacting substances by trypsin digestion, RP-HPLC and N-terminal amino acid sequencing
RP-HPLC fractions containing Gal-9-interacting substances were combined into three pools, AF4 ~ AF6 (AF46), AF7 ~ AF9 (AF79), and AF10 ~ AF12 (AF1012). AF46, AF79, and AF1012 were digested with trypsin and then individually analyzed by RP-HPLC (Fig. ). The thirteen, twelve, and eight peak components derived from AF46, AF79, and AF1012, respectively, were subjected to N-terminal amino acid sequence analysis after rechromatography under the same conditions. Only the results for the peak components for which clear N-terminal amino acid sequences were obtained are summarized in Table . Although no clear sequence corresponding to porcine elastin was detected in the peak components subjected to sequence analysis, peptide fragments derived from five members of the SLRP family, versican, lumican, osteoglycin/mimecan, prolargin and fibromodulin, were identified. The peptides derived from lumican and prolargin were most frequently found (eight and seven peptides, respectively). Of note was that the lumican-derived peptides contained three different N-glycosylation sites. The peptides identified in AF46-T8 (GLVXLTFIHL) and AF79-T7 (LGSFDGLVXL) represent overlapping peptides containing the same N-glycosylation site. In the case of versican and osteoglycin/mimecan, three peptides were identified for each protein, and a fibromodulin-derived peptide was only detected in a single fraction.
Fig. 3. RP-HPLC chromatograms of trypsin digests.
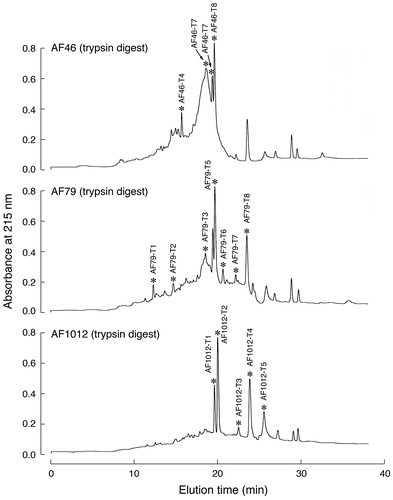
Table 1. N-terminal amino acid sequences of tryptic peptides derived from PES-(E)·PE·AF.
Tropoelastin deposits on microfibrils, which consisted of fibrillins, microfibril associated proteins, microfibril associated glycoproteins, and other peripheral proteins, in the formation of elastic fibers. In addition to microfibrillar components, a variety of ECM proteins, including SLRPs (biglycan and versican), are known to associate with elastic fibers.Citation23) The results shown in Fig. (B) and those obtained using water-soluble elastin preparations suggest that SLRPs are covalently linked to insoluble elastin via non-disulfide bonds. It is likely that the bonds between SLRPs and insoluble elastin are more susceptible to organic acid hydrolysis than desmosine and isodesmosine cross-links (unique constituents of elastin), and thus they were only present in PES-(E) fraction. On the other hand, an insoluble elastin core rich in the cross-links is relatively resistant to hydrolysis and thus needs longer time for solubilization. Currently, whether the interaction between Gal-9 (and other members of the galectin family) and SLRPs associated with insoluble elastin occurs in vivo is unclear. It is possible, however, that insoluble elastin fibers serve as an extracellular reservoir for galectins via attached SLRPs, because elastin is one of the major ECM components, especially in elastic tissues like the lungs, blood vessels, and skin.
There is accumulating evidence that a series of ECM molecules proteolytically released following tissue injury act as danger associated molecular patterns (DAMPs).Citation24) ECM-derived DAMPs interact with pattern recognition receptors, including Toll-like receptors (TLRs), and modulate innate immune responses. Biglycan and decorin, members of the SLRP family, are known to act as DAMPs upon proteolysis by interacting with TLR2/4.Citation25,26) Lumican and versican are not canonical DAMPs but known to modulate TLR signaling.Citation27,28) These findings suggest another function of the possible galectin-SLRP interaction on the surface of insoluble elastin fibers: the interaction modulates proteolytic release of SLRPs linked to elastin and/or the affinity of proteolytic fragments of SLRPs for TLRs. The immunomodulatory nature of the galectin family is consistent with this speculation.
Author contribution
NN designed the experiments and wrote the manuscript. AI and NN carried out major experiments. YM, TO, and TN provided valuable advice and helped to draft the manuscript.
Funding
This study was supported by the Ministry of Education, Culture, Sports, Science and Technology, Japan for Scientific Research (C) [grant number 15K06974].
Supplemental material
Supplemental material for this article can be accessed at https://doi.org/10.1080/09168451.2017.1374828.
Disclosure statement
No potential conflict of interest was reported by the authors.
supplementary_figureS4.jpg
Download JPEG Image (2.2 MB)supplementary_figureS3.jpg
Download JPEG Image (1.5 MB)supplementary_figureS2.jpg
Download JPEG Image (1.3 MB)supplementary_figureS1.jpg
Download JPEG Image (902 KB)Notes
Abbreviations: CSGal-1, a cysteine-less mutant of Gal-1; DAMP, danger associated molecular pattern; ECM, extracellular matrix; G9Null, a protease-resistant form of galectin-9; Gal, galectin; GST, glutathione S-transferase; RP, reversed phase; SDS-PAGE, SDS-polyacrylamide gel electrophoresis; SLRP, small leucine-rich repeat proteoglycan; TBS, Tris-buffered saline; TLR, toll-like receptor.
References
- Delacour D, Gouyer V, Zanetta JP, et al. Galectin-4 and sulfatides in apical membrane trafficking in enterocyte-like cells. J Cell Biol. 2005;169:491–501.10.1083/jcb.200407073
- Chauhan S, Kumar S, Jain A, et al. TRIMs and galectins globally cooperate and TRIM16 and galectin-3 co-direct autophagy in endomembrane damage homeostasis. Dev Cell. 2016;39:13–27.10.1016/j.devcel.2016.08.003
- Thurston TL, Wandel MP, von Muhlinen N, et al. Galectin 8 targets damaged vesicles for autophagy to defend cells against bacterial invasion. Nature. 2012;482:414–418.10.1038/nature10744
- Hughes RC. Secretion of the galectin family of mammalian carbohydrate-binding proteins. Biochim Biophys Acta. 1999;1473:172–185.10.1016/S0304-4165(99)00177-4
- Ozeki Y, Matsui T, Yamamoto Y, et al. Tissue fibronectin is an endogenous ligand for galectin-1. Glycobiology. 1995;5:255–261.10.1093/glycob/5.2.255
- Ochieng J, Furtak V, Lukyanov P. Extracellular functions of galectin-3. Glycoconj J. 2004;19:527–535.
- He J, Baum LG. Presentation of galectin-1 by extracellular matrix triggers T cell death. J Biol Chem. 2004;279:4705–4712.10.1074/jbc.M311183200
- Warfield PR, Makker PN, Raz A, et al. Adhesion of human breast carcinoma to extracellular matrix proteins is modulated by galectin-3. Invasion Metastasis. 1997;17:101–112.
- Probstmeier R, Montag D, Schachner M. Galectin-3, a beta-galactoside-binding animal lectin, binds to neural recognition molecules. J Neurochem. 1995;64:2465–2472.
- Hikita C, Vijayakumar S, Takito J, et al. Induction of terminal differentiation in epithelial cells requires polymerization of hensin by galectin 3. J Cell Biol. 2000;151:1235–1246.10.1083/jcb.151.6.1235
- Gendronneau G, Sidhu SS, Delacour D, et al. Galectin-7 in the control of epidermal homeostasis after injury. Mol Biol Cell. 2008;19:5541–5549.10.1091/mbc.E08-02-0166
- Levy Y, Arbel-Goren R, Hadari YR, et al. Galectin-8 functions as a matricellular modulator of cell adhesion. J Biol Chem. 2001;276:31285–31295.10.1074/jbc.M100340200
- Nobumoto A, Nagahara K, Oomizu S, et al. Galectin-9 suppresses tumor metastasis by blocking adhesion to endothelium and extracellular matrices. Glycobiology. 2008;18:735–744.10.1093/glycob/cwn062
- Fukata Y, Itoh A, Nonaka Y, et al. Direct cytocidal effect of galectin-9 localized on collagen matrices on human immune cell lines. Biochim Biophys Acta. 2014;1840:1892–1901.10.1016/j.bbagen.2014.01.019
- Giro MG, Davidson JM. Elastin. Methods Enzymol. 1988;163:656–673.10.1016/0076-6879(88)63055-2
- Foster JA, Bruenger E, Gray WR, et al. Isolation and amino acid sequences of tropoelastin peptides. J Biol Chem. 1973;248:2876–2879.
- Nishi N, Itoh A, Fujiyama A, et al. Development of highly stable galectins: truncation of the linker peptide confers protease-resistance on tandem-repeat type galectins. FEBS Lett. 2005;579:2058–2064.10.1016/j.febslet.2005.02.054
- Abramoff MD, Magalhaes PJ, Ram SJ. Image processing with image. J Biophotonics Int. 2004;11:36–42.
- Matsumura K, Higashida K, Ishida H, et al. Carbohydrate binding specificity of a fucose-specific lectin from Aspergillus oryzae: a novel probe for core fucose. J Biol Chem. 2007;282:15700–15708.10.1074/jbc.M701195200
- Fullmer CS. Identification of cysteine-containing peptides in protein digests by high-performance liquid chromatography. Anal Biochem. 1984;142:336–339.10.1016/0003-2697(84)90473-1
- Nishi N, Abe A, Iwaki J, et al. Functional and structural bases of a cysteine-less mutant as a long-lasting substitute for galectin-1. Glycobiology. 2008;18:1065–1073.10.1093/glycob/cwn089
- Ochieng J, Warfield P, Green-Jarvis B, et al. Galectin-3 regulates the adhesive interaction between breast carcinoma cells and elastin. J Cell Biochem. 1999;75:505–514.10.1002/(ISSN)1097-4644
- Mithieux SM, Weiss AS. Elastin. Adv Protein Chem. 2005;70:437–461.10.1016/S0065-3233(05)70013-9
- Schaefer L. Complexity of danger: the diverse nature of damage-associated molecular patterns. J Biol Chem. 2014;289:35237–35245.10.1074/jbc.R114.619304
- Schaefer L, Babelova A, Kiss E, et al. The matrix component biglycan is proinflammatory and signals through Toll-like receptors 4 and 2 in macrophages. J Clin Invest. 2005;115:2223–2233.10.1172/JCI23755
- Merline R, Moreth K, Beckmann J, et al. Signaling by the matrix proteoglycan decorin controls inflammation and cancer through PDCD4 and MicroRNA-21. Sci Signal. 2011;4:ra75.
- Wu F, Vij N, Roberts L, et al. A novel role of the lumican core protein in bacterial lipopolysaccharide-induced innate immune response. J Biol Chem. 2007;282:26409–26417.10.1074/jbc.M702402200
- Kim S, Takahashi H, Lin WW, et al. Carcinoma-produced factors activate myeloid cells through TLR2 to stimulate metastasis. Nature. 2009;457:102–106.10.1038/nature07623