Abstract
It has been reported that treatment with yeast cell wall extract (YCWE) induces PDF1 and PR-1 gene expression; these transcripts are important markers of plant disease resistance, though the detailed signaling mechanisms that induce these defense responses are still unknown. In this report, we found that YCWE treatment triggered rice cell suspension cultures to accumulate phenylalanine (Phe), cis-12-oxo-phytodienoic acid (OPDA), 12-hydroxyjasmonoyle isoleucine (12OHJA-Ile), and azelaic acid (AzA). YCWE treatment also reduced endogenous triacylglycerol (TG) content. The addition of 13C-uniform-labeled oleic, linoleic and linolenic acids to the rice cell suspension cultures gave rise to 13C-uniform-labeled AzA. It was also found that YCWE treatment for Arabidopsis thaliana resulted in accumulations of OPDA, AzA, Phe, and camalexin together with enhanced resistance against Botrytis cinerea infection. This suggested that YCWE treatment upon plants may activate JA and AzA signaling systems to induce plant disease resistance.
Yeast cell wall extract (YCWE) treatment induces plant defense response.
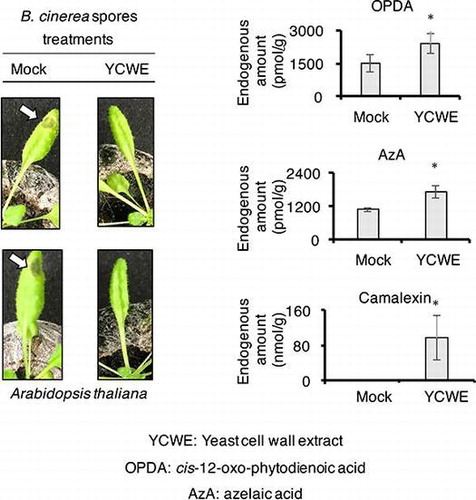
In the wild, plants continuously stand for an attack by harmful insects and pathogens including fungi, bacteria, and viruses. Each of these invaders has developed highly specialized features for targeting plants, while plants themselves are armed against the attackers’ specialized features. Among of the signs, the patterns of oligosaccharide on the surface of cell wall of the fungi were one of remarkable example. After recognizing the invasion, plants mediate a wide range of adaptive plant responses to resist infection and colonization by pathogens, during which biologically active secondary metabolites such as abscisic acid (ABA), ethylene, jasmonic acid (JA), and salicylic acid (SA) act as indispensable signaling compounds.Citation1) Plants also establish their defense systems to make provisions against secondary attacks after suffering from a first invasion. In this stage, plants are able to quickly build up their defense systems to eliminate secondary attackers. Jung et al.Citation2) reported that azelaic acid (AzA, nonane-1,9-dioic acid) was a key component for promoting prompt plant responses, which is called priming. Biosynthetic pathway to give AzA was reported by Gao et al.Citation3) and Zoeller et al.Citation4), in which unsaturated C18 lipids released from monogalactosyldiacylglycerol (MGDG) and digalactosyldiacylglycerol (DGDG) were converted to AzA. It was suggested that an artificial inducement of priming would be ideal for agriculture, as the use of innate plant potential has less of impacts on nature. Moreover, a detailed understanding of the plant immune system will underpin improvements in crop production.Citation5,6)
Budding yeast or Saccharomyces pastorianus is known as a bottom fermentation yeast used in the brewing industry. Polysaccharides such as glucan and mannan are major components of yeast cell walls.Citation7) These polysaccharides display similarity with pathogenic polysaccharides, and it is thought that these polysaccharides are able to elicit plant defense responses.Citation8) It has been reported that yeast cell wall extract (YCWE) has the potential to act as an activator to elicit plant defense responses, such as activating the PR-1a and PDF1.2 genes.Citation9,10) On the market, Housaku Monogatari (HM) was developed as a plant activator prepared from YCWE and found to have potential as an inducer of plant defense responses to reduce infections, although the detailed signaling mechanisms that induce these defense responses are still unknown. In this paper, we report that treatment of rice cell suspension cultures with YCWE decreased triacylglycerol (TG) contents and increased endogenous AzA, 12-oxo-phytodienoic acid (OPDA), phenylalanine (Phe), and 12-hydroxyjasmonoyl isoleucine (12OHJA-Ile) together with findings that oleic and linoleic acids acted as a preferential starting material to produce AzA compared with linolenic acid. We also found that YCWE treatment for Arabidopsis thaliana resulted in accumulations of OPDA, AzA, Phe, and camalexin together with enhanced resistance against Botrytis cinerea infection.
Materials and methods
General
NMR spectra were recorded in CDCl3 using a JNM-EX 270 FT-NMR spectrometer (JEOL). 1H NMR spectra were recorded at 270 MHz and 13C NMR at 67.5 MHz. 1H NMR were also recorded at 500 MHz and 13C NMR at 125.8 MHz on an AMX 500 (Bruker). FDMS and FIMS analyses were performed on a JMS-T100GCV (JEOL) instrument. Ultra performance liquid chromatography (UPLC) was performed on a Waters ACQUITY UPLC system (Waters), which was equipped with a binary solvent delivery manager and a sample manager. MS was performed on a Waters Micromass Quattro Premier Tandem Quadrupole Mass Spectrometer in multiple reaction monitoring mode (MRM). UPLC/MS system control was by MassLynx 4.0. The Oryza sativa accession C5928 callus line (RPC00031) was obtained from RIKEN BRC (Tsukuba, Japan). Rice suspension cell line (RPC00031) was also provided by the Experimental Plant Division of the RIKEN BRC through the National Bio-Resource Project of the MEXT, Japan. Uniform 13C-labeled oleic, linoleic, and linolenic acids were purchased from Cambridge Isotope Laboratories, Inc. (U.S.A.). Indole-d7 from Kanto Kagaku Co., Ltd (Japan).
Preparation of YCWE
The yeast cell wall extract (YCWE) was prepared from the budding yeast Saccharomyces pastorianus during the beer brewing process. The yeast slurry collected after beer brewing was heated at 47 °C for 48 h to obtain a self-digestion yeast. The self-digestion yeast was centrifuged to remove the supernatant after treatment with an autoclave (121 °C, 15 min). YCWE was produced by drum drying the pellet.
Preparation of camalexin-d5
Deuterium-labeled camalexin was synthesized according to the reported method Citation11), except that deuterium-labeled indole-d7 was used as the deuterium source.
Camalexin-d5
1H NMR (270 MHz, CDCl3): δ 8.67 (1H, br), 8.25 (1H, d, J = 3.2 Hz), and 7.3 (1H, d, J = 3.2 Hz). HRFDMS: m/z for C111H32H5N2S1, calculated: 205.07220 and found: 205.07318.
Preparation of rice cell suspension cultures, and YCWE and labeled fatty acid treatments
Rice cell suspension cultures were maintained according to the protocol provided by the BRC.Citation12–14) The culture was pre-incubated for 5 days, and then 80 mL was dispensed into a 300 mL Erlenmeyer flask. A mixture of a sterilized YCWE (40 mg) in H2O (400 μL) was added to the culture, which was further incubated for 2 days (26 °C, 110 rpm, in the dark), and then harvested. For the feeding experiments, 13C-uniform-labeled oleic, linoleic, and linolenic acids (26.6 mmol) and vitamin E (8.69 nmol) were dissolved in EtOH (1 mL). The, the solution containing the labeled fatty acids and vitamin E (125 μL) was added to the culture (80 mL), followed by incubation (26 °C, 110 rpm, in the dark). After 5 days, sterilized YCWE (40 mg) in H2O (400 mL) was added to the culture, which was further incubated for an additional 2 days (26 °C, 110 rpm, in the dark) and then harvested.
TG isolation
The rice callus (125 g) was extracted at room temperature with EtOH (600 mL). The extracts were evaporated under reduced pressure to give a residue, which was subjected to a silica gel column chromatography (250 g, Silica Gel 60, Kanto Kagaku Co. Ltd.,) using n-hexane-EtOAc (98:2) as the eluent to afford TG (12 mg).
GC-MS analysis of TG
To a stirred solution of TG (10 mg) in anhydrous MeOH (3 mL) was added powders of NaOMe (5 mg), and the reaction mixture was stirred for 24 h at room temperature. Then, an excess of Amberlite 120B was added to the reaction mixture and the mixture was filtered to give a solution. The volatile components of the solution were removed under reduced pressure to give an oil, which was dissolved with CHCl3. A portion of the solution was subjected for GC-MS analysis according to following conditions.
GC-MS conditions
GC-MS analysis was completed on a Varian CP-3800 gas chromatograph with a Varian 1200L quadrupole MS/MS in electron ionization mode. For the analysis, the injection, detector, and transfer line temperatures were set at 200, 250, and 250 °C, respectively, and a fused-silica capillary column (TC-5; 30 m × 0.25 mm i.d., 0.25 μm film thickness; GL Sciences) was used. The temperature program started at 80 °C for 1 min and subsequently increased at 22 °C /min to 290 °C, which was maintained for 20 min. Helium was used as the carrier gas at a linear velocity of 1.2 mL/min, and all spectra were scanned within the range m/z 10–520.
Evaluation of endogenous amounts of target compounds in rice cell suspension culture
The harvested rice cell suspension culture was filtered with gauze, and the collected rice callus (ca. 2 g) was frozen in liquid nitrogen and extracted using 10 ml of ethanol. The extract was filtered using cotton, and the volatile components from the extract were removed under reduced pressure to produce a residue. Evaluation of the endogenous amounts of OPDA, 12OHJA-Ile, Phe, and AzA in the residue were performed according to reported methods.Citation15–17)
Evaluation of endogenous amounts of target compounds in A. thaliana
Seeds of A. thaliana (ecotype: Col-0) were planted in Jiffy-7 pots (ϕ 42 mm) that had each absorbed 50 mL of water. Seedlings were grown in a growth chamber at 22 °C and 60% humidity. The plants were grown in 14 h/10 h dark/light conditions. Water was provided once a day. A 500-fold dilution of Hyponex was added once a week for nutrition. To ensure that only one plant was grown per pot, excess plants were removed. After 35 days of growth, the plants were sprayed with YCWE solution (5 g/ 1L H2O) until the liquid dropping from leaves in every other day. The plants treated with the solution in three times were harvested after two day of the final treatment, frozen in liquid nitrogen, and extracted using 20 ml of ethanol. The extract was filtered using cotton, and the volatile components from the extract were removed under reduced pressure to produce a residue. Evaluation of the endogenous amounts of target compounds were performed according to reported methods Citation15−Citation17), except for the addition of camalexin-d5 (200 ng/sample) as an internal standard.
Pathogen inoculation procedure
A. thaliana was prepared according to the above-mentioned method. B. cinerea fungal culture (MAFF No. 237695) was obtained from The Genetic Resources Center, National Agriculture and Food Research Organization (NARO). The experiments were done according to the reported methodCitation18) using a solution containing spores of Botrytis cinerea (5 × 105/ mL). In briefly, total ten plants were used for the experiment, in which five plants were treated with YCWE solution and other five plants were given mock treatment. One fully expanded younger leaf in each plant was given the one drop of the solution containing the spores. The plants were put in a box keeping high humidity.
Results
YCWE treatment triggers the accumulation of OPDA, 12OHJA-Ile, AZA, and Phe in rice cell suspension culture
It is generally accepted that the induced plant disease resistance triggered by pathogenic attack is associated with endogenous amounts of disease resistance marker plant hormones such as abscisic acid (ABA), salicylic acid (SA), and jasmonic acid (JA) together with JA related compounds, OPDA, 12OHJA, 12OHJA-Il, and JA-Ile. It was also reported that azelaic acid (AzA) and pheniylalanine (Phe) were key compounds to establish plant defense system. To investigate whether YCWE acted as an inducer of resistance marker compounds in the rice cell suspension culture, endogenous amounts of OPDA, JA, JA-Ile, 12OHJA, 12OHJA-Ile, ABA, SA, Phe, and AzA (Fig. ) were examined in the rice cell suspension culture using UPLC MS/MS. The results revealed that YCWE treatment led to significant increases in endogenous amounts of OPDA, 12OHJA-Ile, Phe, and AzA in rice callus (Fig. A–D), although a significant decrease of that of JA (Fig. E), which was thought due to avid metabolism of JA into 12OHJA-Ile. However, it was not observed that significant differences in those of JA-Ile and ABA (Fig. S1 in the supplemental material), and the levels of endogenous amounts of SA and 12OHJA were under the detection limits. This suggests that YCWE application positively regulated JA and AzA signaling systems.
Fig. 2. Endogenous amounts of OPDA (A), 12OHJA-Ile (B), Phe (C), and AzA (D) in rice cell suspension cultures.
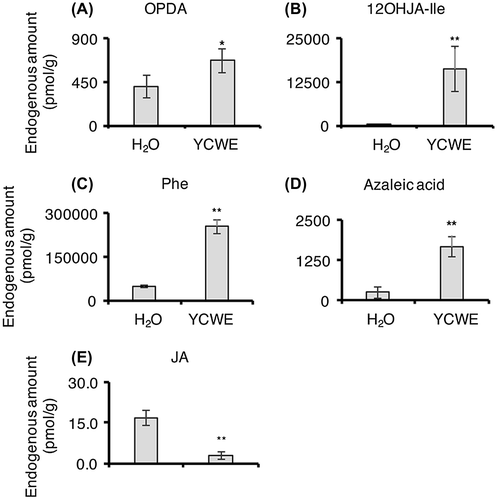
YCWE treatment induces TG disassembly in rice cell suspension culture
To investigate the other biological effects, differences in the components between the YCWE- and non-treated cell cultures were evaluated using a technique of thin layer chromatography (TLC), which were eluted with several organic solvent combinations. Distinct differences were observed when using an n-hexane:EtOAc 95:5 solvent ratio as the eluent. A representative TLC is shown in Fig. S2; the TLCs revealed that a certain ingredient was reduced. The reduced compound was isolated from the EtOH extracts in the non-treated rice cell suspension culture. The 1H- and 13C-NMR spectra are shown in Figs. S3 and S4 in the supplemental material. The 1H-NMR spectra displayed similarities with those of triacyglyceride (TG), as reported by Szczepaniak et al.Citation19) Overall, analysis of the 2D spectral data, including COSY, HMQC, and HMBC (Fig. S5–7 in the supplementary data), found that the isolated compound was a TG, whose fatty acids moieties were evaluated using GC-MS analysis after being treated with a solution of NaOMe in anhydrous MeOH. The GC-MS characteristic total ion chromatogram (TIC) and MS pattern for each peak are shown in Figs. S8 and S9 in the supplemental material. The patterns and retention times coincided well with those for authentic oleic, linoleic, linolenic, and palmitic acid methyl esters, whose authentic MS patterns are shown in Fig. S10 in the supplemental material. The portions of palmitic, linoleic, linolenic, and oleic acids were roughly determined as 5:4:2:1 based on the comparison of the ratio of their peak areas. It was hypothesized that YCWE treatment might stimulate the disassembly of TG to afford fatty acids, which can be partially utilized as intermediates for AzA biosynthesis.
Conversion of 13C-uniform-labeled C18 fatty acids into AzA
Gao et al.Citation3) showed that unsaturated C18 lipids released from monogalactosyldiacylglycerol (MGDG) and digalactosyldiacylglycerol (DGDG) were converted to AzA using 14C-labeled compounds together with TLC technique. They injected 14C-1 labeled oleic acid into A. thaliana leaves and analyzed the ingredients in leaf extracts by TLC after treatment with a CH2N2 solution in Et2O. Radioactive dimethyl azelate was detected by TLC. Zoeller et al.Citation4) reported that TG are a starting material that can give rise to AzA. To prove whether the rice cell suspension culture utilizes oleic, linoleic, and linolenic acids to afford AzA, 13C-uniform-labeled oleic, linoleic, and linolenic acids were added to the suspension cultures and the metabolites were examined using an UPLC MS/MS system. The representative UPLC MS/MS features are show in Fig. . As the result of the investigation, it became clear they revealed that 13C-uniform-labeled oleic, linoleic, and linolenic acids were metabolized into 13C-uniform-labeled AzA (Fig. B–D), while mock treatment did not give the AzA (Fig. A). Moreover, endogenous amounts of 13C-uniform-labeled AzA derived from the 13C-uniform-labeled oleic and linoleic acids were increased upon YCWE treatment (Fig. ), although the endogenous amount of 13C-uniform-labeled AzA derived from 13C-uniform-labeled linolenic acid was lower than those derived from 13C-uniform-labeled oleic and linoleic acids. Thus, we hypothesized that linolenic acid might be used preferentially for the production of JA and its derivatives upon YCWE treatment. This hypothesis was supported by the measurement of endogenous amounts of 13C-uniform-labeled 12OHJA conjugated with L-isoleucine acid (Fig. ), which revealed that amounts of 13C-uniform-labeled 12OHJA conjugated with L-isoleucine acid derived from 13C-uniform-labeled linolenic acid (Fig. C) were higher than those derived from 13C-uniform-labeled oleic and linoleic acids (Fig. A and B).
Fig. 3. Biosynthesis of AzA.
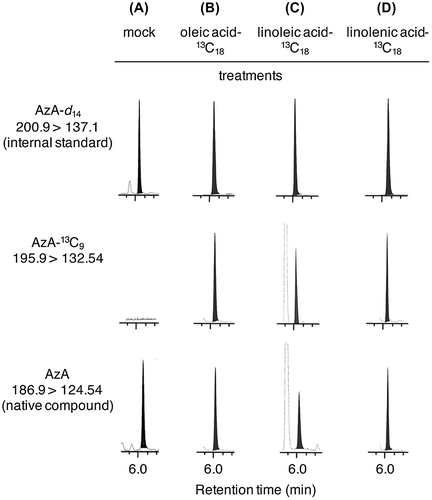
Fig. 4. Endogenous amounts of AzA-13C9 in rice cell suspension cultures treated with oleic acid-13C18 (A), linoleic acid-13C18 (B), and linolenic acid-13C18 (C).
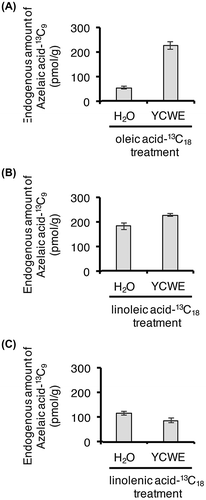
Fig. 5. (A) Endogenous amount of 13C12–12OHJA-Ile in rice cell suspension cultures treated with oleic acid-13C18 (A), linoleic acid-13C18 (B), and linolenic acid-13C18 (C). Bars indicate the mean ± S.E. (n = 6).
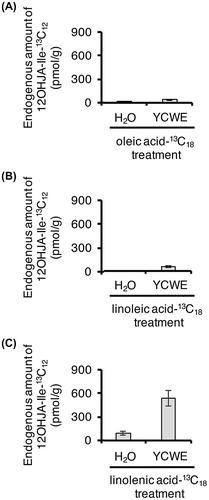
YCWE treatment stimulates the accumulation of Phe, OPDA, AzA, and camalexin in Arabidopsis thaliana
Because treatment with YCWE promoted the accumulation of Phe, OPDA, 12OHJA-Ile, and AzA in rice cell suspension culture, it was hypothesized that the treatment of YCWE upon A. thaliana might induce the accumulation of the same compounds and phytoalexin, such as camalexin (Fig. ). To test this hypothesis, an internal standard, camalexin-d5, for quantifying endogenous amounts of camalexin was synthesized according to the reported method except for using deuterium-labeled indole-d7 as a deuterium source. A. thaliana was treated with a YCWE solution, and the endogenous biological active compounds in plant extracts were examined using an UPLC MS/MS system. A schematic illustration of experimental time course is shown in Fig. A, and the results are shown in Fig. B. The results revealed that YCWE treatment stimulated the accumulation of OPDA, AzA, Phe, and camalexin, although no significant accumulation of 12OHJA, 12OHJA-Ile, JA, JA-Ile, ABA, and SA (Fig. S11 in the supplemental material). As a next step, YCWE-treated and non-treated A. thaliana leaves were treated with Botrytis cinerea spores (Fig. S12 in the supplemental material). A schematic illustration of experimental time course is shown in Fig. S12A, and the results are shown in Fig. S12B and C. In the case of the YCWE non-treated A. thaliana plants, the infection rate increased to close to 80% (Fig. S12B, n = 5), while the infection rate remained near 20% (Fig. S12C, n = 5) in the YCWE-treated plants. Other one independent experiment gave almost same result. This suggests that YCWE treatment enhanced the ability of A. thaliana plants to withstand a pathogenic attack.
Fig. 6. Endogenous amounts of OPDA, 12OHJA-Ile, Phe, AzA, and camalexin in A. thaliana.
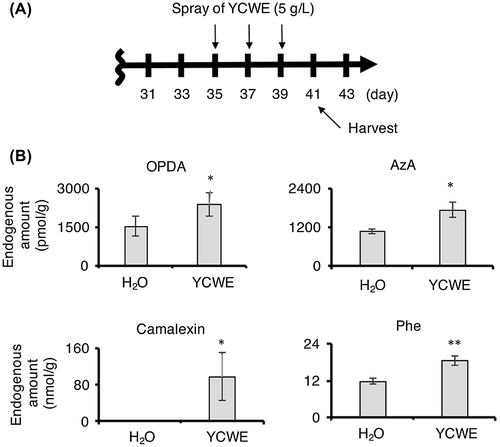
Discussion
It is generally accepted that increments of Phe are related to the up-regulation of the Phenylalanine Ammonialyase gene, whose promotion is a key factor involved in inducing plant defense systems.Citation20) OPDA is an intermediate in the biosynthetic pathway that generates JA, and Taki et al.Citation21) reported that OPDA accelerated the expression of a distinct set of genes and had a role in wound-induced gene expression in A. thaliana. 12OHJA-Ile is a JA metabolite via JA-Ile, and JA-Ile is thought to be the actual active compound in the JA-mediated signaling pathway upon wound stress.Citation22) Jung et al. reported that the biological role of AzA was as an inducer that primes plants against pathogenic attacks.Citation2) Because YCWE treatment stimulated the biosynthesis of Phe, 12OHJA-Ile, and AzA in rice cell suspension culture, it was hypothesized that YCWE treatment may promote plant defense systems through the accumulations of Phe, JA-related compounds, and the priming agent AzA. This hypothesis was supported by the experimental results of Fig. , Figs. S11, and S12 in the supplemental material, although the exact relationship between the JA and AzA signaling systems remains for scientists to argue about. In this report, we also found that rice cell suspension culture has an operative pathway for producing AzA using oleic, linoleic, and linolenic acids derived from TG. Our strategy using 13C-uniform-labeled fatty acids is the first study to determine the biosynthetic pathway of AzA using MS data.
Author contributions
TY and TK performed the experiments. TK and HM designed the study and wrote paper. TI, TY, and KT helped to draft the manuscript.
Disclosure statement
No potential conflict of interest was reported by the authors.
Supplemental materials
The supplemental materials for this paper are available at https://doi.org/10.1080/09168451.2017.1379351.
Supplementary_Fig_06.pdf
Download PDF (993.4 KB)Acknowledgements
The MeJA used as a starting material for the synthesis of deuterium-labeled compounds was kindly provided by the ZEON Corporation (Tokyo, Japan). We acknowledge the assistance of Miss. Chikako Iwabuchi (Asahi Breweries, Ltd.) and Mrs. Takase Iijima (Asahi Group Holdings, Ltd.) in cultivation of rice cell suspension. We also acknowledge the assistance of Dr. Eri Fukushi and Mr. Yusuke Takata (Research Faculty of Agriculture, Hokkaido University) in obtaining the spectroscopic data. We used the UPLC MS/MS systems at the Research Faculty of Agriculture, Hokkaido University.
References
- Pieterse CMJ, Leon-Reyes A, Van der Ent S, et al. Networking by small-molecule hormones in plant immunity. Nat Chem Biol. 2009;5:308–316.10.1038/nchembio.164
- Jung H, Tschaplinski T, Wang L, et al. Priming in systemic plant immunity. Science. 2009;324:89–91.10.1126/science.1170025
- Gao QM, Yu KS, Xia Y, et al. Mono- and digalactosyldiacylglycerol lipids function nonredundantly to regulate systemic acquired resistance in plants. Cell Rep. 2014;9:1681–1691.10.1016/j.celrep.2014.10.069
- Zoeller M, Stingl N, Krischke M, et al. Lipid profiling of the arabidopsis hypersensitive response reveals specific lipid peroxidation and fragmentation processes: biogenesis of pimelic and azelaic acid. Plant Physiol. 2012;160:365–378.10.1104/pp.112.202846
- Jones JDG, Dangl JL. The plant immune system. Nature. 2006;444:323–329.10.1038/nature05286
- Hilker M, Schwachtje J, Baier M, et al. Priming and memory of stress responses in organisms lacking a nervous system. J Biol Rev. 2016;91:1118–1133.10.1111/brv.2016.91.issue-4
- Klis FM, Boorsma A, De Groot PWJ. Cell wall construction in Saccharomyces cerevisiae. Yeast. 2006;23:185–202.10.1002/(ISSN)1097-0061
- Basse CW, Fath A, Boller T. High-affinity binding of glycopeptide elicitor to tomato cells and microsomal-membranes and displacement by specific glycan suppressors. J Biol Chem. 1993;268:14724–14731.
- Minami T, Tanaka T, Takasaki S, et al. In vivo bioluminescence monitoring of defense gene expression in response to treatment with yeast cell wall extract. Plant Biotechnol. 2011;28:481–484.10.5511/plantbiotechnology.11.1020a
- Narusaka M, Minami T, Iwabuchi C, et al. Yeast cell wall extract induces disease resistance against bacterial and fungal pathogens in arabidopsis thaliana and brassica crop. PLOS ONE. 2015;10:e0115864.10.1371/journal.pone.0115864
- Ayer WA, Craw PA, Ma YT, et al. Synthesis of camalexin and related phytoalexins. Tetrahedron. 1992;48:2919–2924.10.1016/S0040-4020(01)90973-1
- Baba A, Hasezawa S, Syono K. Cultivation of rice protoplasts and their transformation mediated by agrobacterium spheroplasts. Plant Cell Physiol. 1986;27:463–471.
- Kawaguchi M, Kobayashi M, Sakurai A, et al. The presence of an enzyme that converts indoIe-3-acetamide into IAA in wild and cultivated rice. Plant Cell Physiol. 1991;32:143–149.10.1093/oxfordjournals.pcp.a078058
- Matsubayashi Y, Takagi L, Sakagami Y. Phytosulfokine-alpha, a sulfated pentapeptide, stimulates the proliferation of rice cells by means of specific high-and low-affinity binding sites. Proc Nat Acad Sci USA. 1997;94:13357–13362.10.1073/pnas.94.24.13357
- Matsuura H, Aoi A, Satou C, et al. Simultaneous UPLC MS/MS analysis of endogenous jasmonic acid, salicylic acid, and their related compounds. Plant Growth Regul. 2009;57:293–301.
- Sato C, Oka N, Nabeta K, et al. Cellulase applied to the leaves of sweet pepper (Capsicum annuum L. var. grossum) upregulates the production of salicylic and azelaic acids. Biosci Biotechol Biochem. 2011;75:761–763.
- Kajiwara A, Abe T, Hashimoto T, et al. Efficient synthesis of (+)-cis-12-oxo-phytodienoic acid by an in vitro enzymatic reaction. Biosci Biotechol Biochem. 2012;76:2325–2328.10.1271/bbb.120506
- Liu L, Kracher B, Ziegler J, et al. Negative regulation of ABA signaling by WRKY33 is critical for Arabidopsis immunity towards Botrytis cinerea 2100. Elife. 2015;4:e07295. Doi: 10.7554/eLife.07295
- Szczepaniak LS, Babcock EE, Schick F, et al. Measurement of intracellular triglyceride stores by H-1 spectroscopy: validation in vivo. Am J Physiol-Endoc M. 1999;276:E977–E989.
- Dixon RA, Paiva NL. Stress-induced phenylpropanoid metabolism. Plant Cell. 1995;7:1085–1097.10.1105/tpc.7.7.1085
- Taki N, Sasaki-Sekimoto Y, Obayashi T, et al. 12-oxo-phytodienoic acid triggers expression of a distinct set of genes and plays a role in wound-induced gene expression in arabidopsis. Plant Physiol. 2005;139:1268–1283.10.1104/pp.105.067058
- Chini A, Fonseca S, Fernández G, et al. The JAZ family of repressors is the missing link in jasmonate signalling. Nature. 2007;448:666–671.10.1038/nature06006